Levels of Genotoxic and Carcinogenic Ingredients in Plant Food Supplements and Associated Risk Assessment ()
1. Introduction
Herbal products and ingredients such as herbal teas, food flavors and food supplements have been, and still are, widely used in Western diets. Currently, there is a growing interest in plant food supplements (PFS), which are suggested to have the ability to maintain and promote health or reduce the risk factors for diseases. In Europe, PFS are easily accessible since they can be bought in supermarkets, health-food shops or pharmacies where they are sold over the counter at relatively low costs. Moreover, PFS generally have a high acceptance by consumers who often consider that “natural” equals “safe”. However, this reasoning should be considered with care since several botanicals are known to contain toxic or even genotoxic and carcinogenic compounds [1]. Examples of genotoxic and carcinogenic botanical ingredients are the alkenylbenzenes estragole, methyleugenol and safrole, which are present in a wide range of botanicals including basil, nutmeg, tarragon and fennel [2-4]. In the past, the Scientific Committee on Food has suggested restrictions in the use of these alkenylbenzenes because of their genotoxic and carcinogenic properties [2-4].
In 2004, the Scientific Committee of the European Food Safety Authority (EFSA) presented a discussion paper describing general concerns relating to the quality and safety of botanicals and botanical preparations such as PFS and related products [5]. In line with the existing concerns with respect to the use of botanicals, a European collaborative project under the Seventh Framework Programme was recently started. This project, known as PlantLIBRA (PLANT food supplements: Levels of Intake, Benefits and Risk Assessment), aims to encourage the safe use of food supplements containing botanicals or botanical ingredients by increasing science-based decision making by regulators as well as food chain operators. Within PlantLIBRA, different methodologies for risk assessment of botanicals and botanical ingredients used as PFS will be defined, validated and disseminated in order to enlarge the knowledge on PFS and ensure a safe use of PFS.
In general, assessment of the risk for human health from genotoxic and carcinogenic compounds occurring in food, the presence of which cannot be easily avoided, is accompanied with difficulties [6]. Currently, an international scientific agreement is lacking regarding the best strategy for the risk assessment of genotoxic and carcinogenic compounds and a variety of approaches is used by different regulatory and advisory bodies [6]. While some offer qualitative advice, others present quantitative approaches with respect to the risk assessment of genotoxic carcinogens [7,8].
Amongst the different available qualitative and quantitative approaches, the use of a Margin of Exposure (MOE) approach was recommended by expert groups of EFSA, the Joint FAO/WHO expert committee on Food Additives (JECFA) and the International Life Sciences Institute (ILSI) [6,9-11]. The MOE is a dimensionless ratio based on a reference point obtained from epidemiologic or experimental data on tumor incidence which is divided by the estimated daily intake in humans [6]. The selected reference point corresponds to a dose level causing a low though quantifiable incidence of tumors [6]. Different approaches have been proposed to select an appropriate reference point from the dose-response-curve, of which the use of BenchMark Dose (BMD) modeling is the preferred approach [10]. The BMD approach makes use of different mathematical models for the analysis of the observed carcinogenicity data [12]. Making use of this approach, a dose can be estimated that causes a predefined cancer response, known as the BenchMark Response (BMR) [12]. Generally, a dose that gives 1%, 5% or 10% extra tumor incidence compared to the background level is chosen [12]. However, it is indicated that the use of a dose giving 10% extra cancer risk above the background level (BMD10) is accompanied with the least uncertainties and is therefore preferred [10]. The BMDL10, the 95% lower confidence bound of the BMD10, is frequently used as a reference point to calculate the MOE. This reference point takes into account uncertainties in the experimental data [6]. Moreover, the use of the BMDL instead of the BMD will assure with 95% confidence that the value of the BMR will not go beyond the predefined value of, for example, 10% [6].
An advantage of the use of the MOE approach is that it sets a basis for priority setting that can be used by risk managers. An MOE > 10,000 is considered as a low priority for risk management actions and would be of low concern from a public health point of view [6]. The value of 10,000 was defined based on considering various factors that cause uncertainties in the MOE including 1) a factor 100 for species differences and human variability in toxico-kinetics and toxico-dynamics, 2) a factor 10 for inter-individual human variability in cell cycle control and DNA repair, and 3) a factor 10 for the reason that the BMDL10 when used as a reference point is not identical to a NOAEL [6].
In the presented paper, the MOE approach was applied to a series of compounds known to be present in botanicals and botanical preparations including especially PFS. To this end, first an inventory was made of botanical ingredients that are of possible concern for human health because of their genotoxic and/or carcinogenic properties. In a next step cancer data for a selected number of these compounds were used to derive a reference point preferably a BMDL10. Finally the MOE values for these ingredients were calculated based on the intake estimates resulting from chemical analysis of selected PFS as well as their proposed uses. The outcomes generally pointed at a high priority for risk management of PFS containing the alkenylbenzenes estragole, methyleugenol, safrole and β-asarone.
2. Materials and Methods
2.1. Selection of Botanical Ingredients
An inventory was made of botanical ingredients that are of possible concern for human health because of their genotoxic and/or carcinogenic properties. Selection of these botanical ingredients included three strategies. First, compounds indicated to be genotoxic or carcinogenic in the EFSA compendium [13] were selected as potential candidates for risk assessment using the MOE concept. The EFSA compendium summarizes a large number of botanicals and botanical ingredients containing substances which might be of possible concern for human health. Second, the selection of botanical ingredients relevant for risk assessment using the MOE concept was based on data obtained from 2-year studies performed by the National Toxicology Program (NTP). To be eligible for selection, evidence of genotoxic and/or carcinogenic activities from these NTP studies was considered as a requirement. Furthermore, compounds tested positive for genotoxicity or carcinogenicity by the NTP were only selected if they were listed in the EFSA compendium, because that ascertained their occurrence in botanicals or botanical preparations, and they were selected even when the EFSA compendium did not list them as genotoxic or carcinogenic. Finally, as a third strategy, this list of selected compounds was completed by including compounds of known concerns because of their genotoxic and/or carcinogenic characteristics, but not (yet) included in the EFSA compendium or not (yet) tested by NTP. These included aristolochic acids as well as a number of botanical ingredients classified as alkenylbenzenes or unsaturated pyrrolizidine alkaloids. For alkenylbenzenes and unsaturated pyrrolizidine alkaloids, priority for risk assessment has been indicated by the partners collaborating within the PlantLIBRA project. Moreover, concerns for human health were raised by the EU with respect to the occurrence of unsaturated pyrrolizidine alkaloids in plants [14], and the alkenylbenzenes including estragole, methyleugenol, safrole, elemicin, myristicin, and apiol have been given priority by JECFA for evaluation of their risks resulting from consumption of botanicals and botanical preparations [15].
2.2. Selection of Carcinogenicity Data Relevant for Benchmark Modelling
In general, the calculation of BMD(L)10 values was based on rodent carcinogenicity bioassays preferably accomplished under current guidelines. For this purpose, several studies implemented by the NTP were used for modeling. These studies often included three exposure concentrations administered to rats and mice of both sexes (50 animals per group) during a lifetime (i.e. 2 years). However, not for all selected botanical compounds such studies were available. Therefore, studies were included that were not performed according to current standards. Selection of studies suitable for BMDL10 modeling included the requirement that studies were conducted using a control group and two or more exposure levels. When studies were completed in a period shorter than the standard lifespan of 2 years, an adjustment for the length of treatment and the observation period was made. This was done following the method reported by the European Chemical Agency (ECHA) [16] and previously applied in the MOE approach as performed by an Expert Panel of ILSI [17]. This adjustment allows a correction for the underestimation in tumor incidence that would occur when an experiment is discontinued before the standard lifespan [17]. For this purpose, a correction of (w1/104) × (w2/104) was applied to the dose in which w1 represents the duration of dosing in weeks and w2 reflects the period of observation in weeks. For example, if rodents were treated for 52 weeks in an experiment proceeding for 80 weeks, the dose (d) was corrected following (52/104) × (80/104) × d. In addition, the dose levels were also corrected for the number of doses per week by adjusting the dose to equal a daily administration of the test compound keeping the total weekly dose constant. Thus, if the test compound was administered for 5 times a week, a correction factor of 5/7 was applied to the dose. When studies reported the dose administered in mg/kg diet, a daily food consumption of 130 g diet/kg bw.day, 120 g diet/kg bw.day and 40 g/kg bw.day for female and male mice and male rats respectively was used to calculate the dose in mg/kg bw.day [18]. Only organ-specific carcinogenicity data were included for modeling with the emphasis on malignant neoplasms. Data on benign tumors were precluded since these neoplastic lesions do not express the same characteristics as malignant tumors that are capable of spreading by invading surrounding tissues or by metastasizing. Although malignant tumors are able of metastasizing, only primary tumors were taken into consideration for modeling. When different malignant tumors were observed in a cancer bioassay, the tumorsite with the highest incidence at comparable dose levels was chosen. Data for males and females were not combined. Other criteria for selecting carcinogenicity data were: the occurrence of a treatment-related doseresponse relationship, oral administration of the compound (i.e. through the diet, drinking water or by gavage) and a tumor type that is of relevance for humans. The number of animals in a group is also needed for adequate BMD modeling and equaled the number of animals that were examined microscopically.
2.3. Benchmark Dose Modeling
BMD(L)10 values were obtained by fitting the carcinogenicity data to a number of different mathematical models using EPA BMDS software, version 2.1.2. The following models were used: Gamma, Logistic, LogLogistic Probit, LogProbit, Multistage, Weibull and the Quantallinear model. BMDS software was applied using default settings for model restrictions, risk type (extra), confidence level (95%), and BMR (10%). The BMD(L)10 values derived from the different fitted models were only accepted if the fit of the selected model was not of poorer quality than that of the so-called full model representing a perfect fit to the dose-response data. For this purpose, the log-likelihood ratio test was applied in order to measure the goodness-of-fit of the chosen models. The acceptance of a model was based on comparing the critical differences in the log-likelihood values between the full and the fitted model as reported by Slob (2002) [19]. The evaluation of these critical differences was based on the discrepancy in the amount of parameters used by the full and the selected model (i.e. the degrees of freedom) [19]. Exceeding the critical difference implied that the model with the highest number of parameters (i.e. the full model) had the best fit [19] and consequently the selected model was not accepted for BMD(L) calculation. Moreover, the p-value representing the goodness-of-fit of the selected dose was taken into account with a value below 0.05 resulting in model rejection.
2.4. Method for Determination of Intake Levels
2.4.1. Theoretical Estimations
Intake estimates resulting from use of PFS were not available in the literature and were made in the present study. PFS were selected that are offered for sale on the market via the internet and/or health shops and that consist of botanicals known to contain different quantities of one or more of the compounds of interest. Exposure estimates were made taking into consideration the content of the compound of interest in the plant as reported in literature. When such data were not available, the amount of essential oil that is reported to be present in the plant and the amount of the compound in the essential oil were used to estimate the ingredient level in the plant. When the amount of compound (in mg) present in a particular botanical was determined, the daily exposure to the compound of interest (in mg/kg bw.day) was calculated based on the daily intake of that botanical resulting from the use of PFS at the dose recommended by the respective manufacturer. In general, data of PFS prepared from one botanical were used. When a PFS consisted of two or more botanicals, the relative amount of the botanical of interest present in the PFS as indicated by the manufacturer was used to correct the daily dose of the PFS. Since manufacturing methods for preparing the PFS from the original plant were not available, it was assumed that the PFS equals the botanical as such.
2.4.2. Quantitative Detection of Alkenylbenzenes in PFS
To determine if the theoretical levels represent the actual levels of the genotoxic carcinogens of interest in commercially available PFS, a quantitative analysis was performed. To this end, the content of the alkenylbenzenes of interest in 28 different PFS containing basil, cinnamon, Sassafras, nutmeg, fennel or calamus was measured. Exposure estimates were made based on the content of the compound of interest in the PFS as measured together with the weight of the capsules and the recommended daily dose as indicated on the label.
1) PFS and chemicals
PFS were selected that are widely offered for sale on the market via the internet and/or health shops and consist of botanicals known to contain different quantities of one or more of the compounds of interest. 28 PFS were purchased via the internet, local Dutch and Italian health shops and a Dutch pharmacy. Methyleugenol (purity 99%), safrole (purity > 97%), β-asarone (purity 70%), methanol (HPLC supra gradient) and acetonitrile (ULC/MS gradient) were supplied by SigmaAldrich (Steinheim, Germany). Estragole (purity 98%) was purchased from Acros Organics (Geel, Belgium). Nano pure water was obtained from a Barnstead Nanopure Type I ultrapure water system.
2) Sample preparation
All 28 alkenylbenzene-containing PFS were extracted using methanol based on the method described by Gursale et al. (2010) [20] with minor modifications. To prepare these methanolic extracts, 1 g of powdered PFS material was suspended in 25 ml methanol followed by ultrasonic extraction for 15 minutes at room temperature. An aliquot of each extract solution was filtered through a 0.45 µm cellulose acetate membrane filter (VWR International) and stored at −20˚C until Ultra Performance Liquid Chromatography (UPLC)-analysis.
3) UPLC analysis
Before UPLC analysis, aliquots of the extract solutions were diluted in nanopure water (1:100 v/v). However, when the level of the analyte was found to be outside the acceptable level for quantification, the final dilution factor was adjusted. Accordingly, extract solutions were diluted in a range of 2 to 1000 fold depending on the quantity of estragole, methyleugenol, safrole or β-asarone present in the sample. After centrifugation at 16,000g for 5 minutes, 3.5 µl of each sample was subjected to UPLC analysis (n = 3).
UPLC analysis was performed on a Waters ACQUITY UPLC H-Class system connected to an ACQUITY UPLC photodiode array detector and a quaternary solvent manager. Chromatographic separation was achieved using an ACQUITY UPLC BEH C18 1.7 µm column, 2.1 × 50 mm. The column was thermostated at 30˚C and the sample manager was set at 10˚C. The gradient was made with nanopure water and acetonitrile. The mobile phase started with 23% acetonitrile for 10 minutes after which the acetonitrile percentage was increased to 58% in 3 minutes and further increased to 80% in 1 minute at which it was kept for 30 seconds. Starting conditions were reached within the next 30 seconds and retained for another 30 seconds. During the whole run, the flow rate was set at 0.6 ml/min. Under these conditions, the retention times for methyleugenol, β-asarone, safrole and estragole were 9.0, 9.6, 12.3, and 12.7 minutes, respectively. The levels of the alkenylbenzenes of interest were quantified by comparing the peak areas to the calibration curves of these compounds derived from commercially available standards.
For nutmeg-containing PFS, a different method was used to separate the structurally related alkenylbenzenes elemicin and methyleugenol which both occur in nutmeg [13]. The gradient was made with nanopure water and acetonitrile. The acetonitrile percentage was increased from 32% to 35% in 3.5 minutes and further increased to 60% in 1.5 minutes and to 80% in 30 seconds at which it was kept for 30 seconds. Starting conditions were obtained in 30 seconds and the system was equilibrated for another 30 seconds. Elution was at a flow rate of 0.6 ml/min and the column temperature and sample temperature were set at 22˚C and 10˚C respectively. Under these conditions, the retention times were 2.9, 3.1 and 4.8 minutes for elemicin, methyleugenol and safrole in the order given.
4) Accuracy
The accuracy of the developed method was assessed by means of a recovery study. Pure standards of commercially available estragole, methyleugenol, safrole or β-asarone were added in different quantities (final concentrations of 250 µM, 500 µM and 750 µM) to 1 g powdered PFS consisting of fennel, nutmeg or calamus dissolved in a final volume of 25 ml methanol. Samples were prepared and analyzed by UPLC as described above (n = 6). The average percentage recoveries were found to equal 87.6% ± 4.9% for estragole, 89.7% ± 8.1% for methyleugenol, 98.9 % ± 15.0% for safrole and for β- asarone this was 81.1% ± 7.7%. Based on these outcomes the levels of the alkenylbenzenes detected in the different PFS were corrected for sample recovery.
2.5. Calculation of the Margin of Exposure
The full range of BMDL10 values (i.e. lowest to highest BMDL10 value) was compared with the estimated daily intakes of the alkenylbenzenes of interest resulting from the use of PFS to derive an MOE range. MOE values are rounded to a single significant figure.
3. Results
3.1. Selection of Compounds
First an inventory was made of botanical ingredients that are of possible concern for human health because of their genotoxic and/or carcinogenic properties. As described in the Materials and Method section, the selection of these botanical ingredients was performed based on 1) the EFSA compendium, 2) the available NTP studies on botanical ingredients and 3) knowledge from partners within the PlantLIBRA consortium. Table 1 provides an overview of the 30 compounds thus selected that might be of concern because of their genotoxic and/or carcinogenic potentials. Most of these compounds occur in a variety of botanicals several of which, such as fennel, are commonly used in PFS. Table 1 also lists the overall judgement on the genotoxicity and carcinogenicity of the selected compounds as based on available literature data. This reveals that not all 30 selected compounds appear to be both genotoxic and carcinogenic. For the majority of the selected botanical ingredients carcinogenicity has been reported or suspected. However, for several compounds, such as for coumarin and trans-anethole, a genotoxic mode of action is not indicated. 18 Of the 30 selected compounds, appeared to be both genotoxic and carcinogenic. These compounds include apiol, aristolochic acid (I and II), elemicin, estragole, heliotrine, lasiocarpine, lucidin, methyleugenol, monocrotaline, myristicin, ptaquiloside, reserpine, riddelliine, safrole, senecionine and symphytine. Although for β-asarone the genotoxicity data are equivocal, EFSA previously concluded that when the carcinogenic mode of action of a certain compound is not identified, a genotoxic mode of action will usually be assumed [6]. However, EFSA mentioned that this presumption of genotoxicity is based on a lack of other information and should not be interpreted as the actual mode of action. Interestingly, most of the selected botanical ingredients that are both genotoxic and carcinogenic belong to the groups of the alkenylbenzenes or the unsaturated pyrrolizidine alkaloids, with the exception of aristolochic acid (I and II), lucidin, reserpine and ptaquiloside. For 8 of the 18 compounds that appeared to be both genotoxic and carcinogenic, data on carcinogenicity are available from which a reference point (e.g. BMDL10 or T25) could be obtained, allowing the use of the MOE concept. These compounds included reserpine, the alkenylbenzenes estragole, methyleugenol, safrole and β-asarone and the unsaturated pyrrolizidine alkaloids riddelliine, lasiocarpine and monocrotaline. Given that the use of unsaturated pyrrolizidine alkaloids in food including PFS is already regulated and not allowed, in the present study further emphasis was placed on the risk assessment of PFS containing the alkenylbenzenes estragole, methyleugenol, safrole and/or β-asarone.
3.2. Carcinogenicity Data and BMDL10
Table 2 gives an overview of the carcinogenicity data describing the incidences of hepatocellular carcinomas in rodents exposed to estragole, methyleugenol or safrole. In the same table, the results of an unpublished study referred to by JECFA (1981) [64] is given on the incidences of leiomyosarcomas of the small intestines of male rats exposed to increasing concentrations of β- asarone. Table 3 presents the results of BMD analysis of these data (for details see Tables 4-7). For estragole, the BMDL10 value varies between 3.3 - 6.5 mg/kg bw.day. For methyleugenol, BMDL10 values ranging between 15.3 and 34.0 mg/kg bw.day were found for male rats whereas BMDL10 values of 48.8 - 73.6 mg/kg bw.day were found for female rats. The BMDL10 values range between 1.9 - 5.1 mg/kg bw.day for safrole and between
9.6 - 21.5 mg/kg bw.day for β-asarone.
3.3. Exposure Data
3.3.1. Intake Estimates of Alkenylbenzenes from Consumption of PFS
Characteristics of the PFS for which intake estimates were made are presented in Table 8. Table 9 presents estimated daily exposure to estragole, methyleugenol, safrole or β-asarone from the use of PFS. These estimates were based on PFS available on the market and known to contain different quantities of botanicals that contain one or more of the alkenylbenzenes of interest. Using the suggested dose of PFS intake as recommended by the manufacturer of the PFS and the estimated alkenylbenzene level in the PFS, daily intake estimates of the alkenylbenzenes resulting from use of the various PFS were obtained. The alkenylbenzene level in the PFS was calculated based on the percentage of the alkenylbenzene present in the essential oil, the percentage of the essential oil in the plant and the amount of the plant in the PFS. Thus, assuming a dose of 230 - 690 mg basil supplement, 0.11% - 85% of estragole present in the essential oil of sweet basil [13,65] and 0.11% - 1.9% of essential oil in this plant [65], the estimated daily intake of estragole
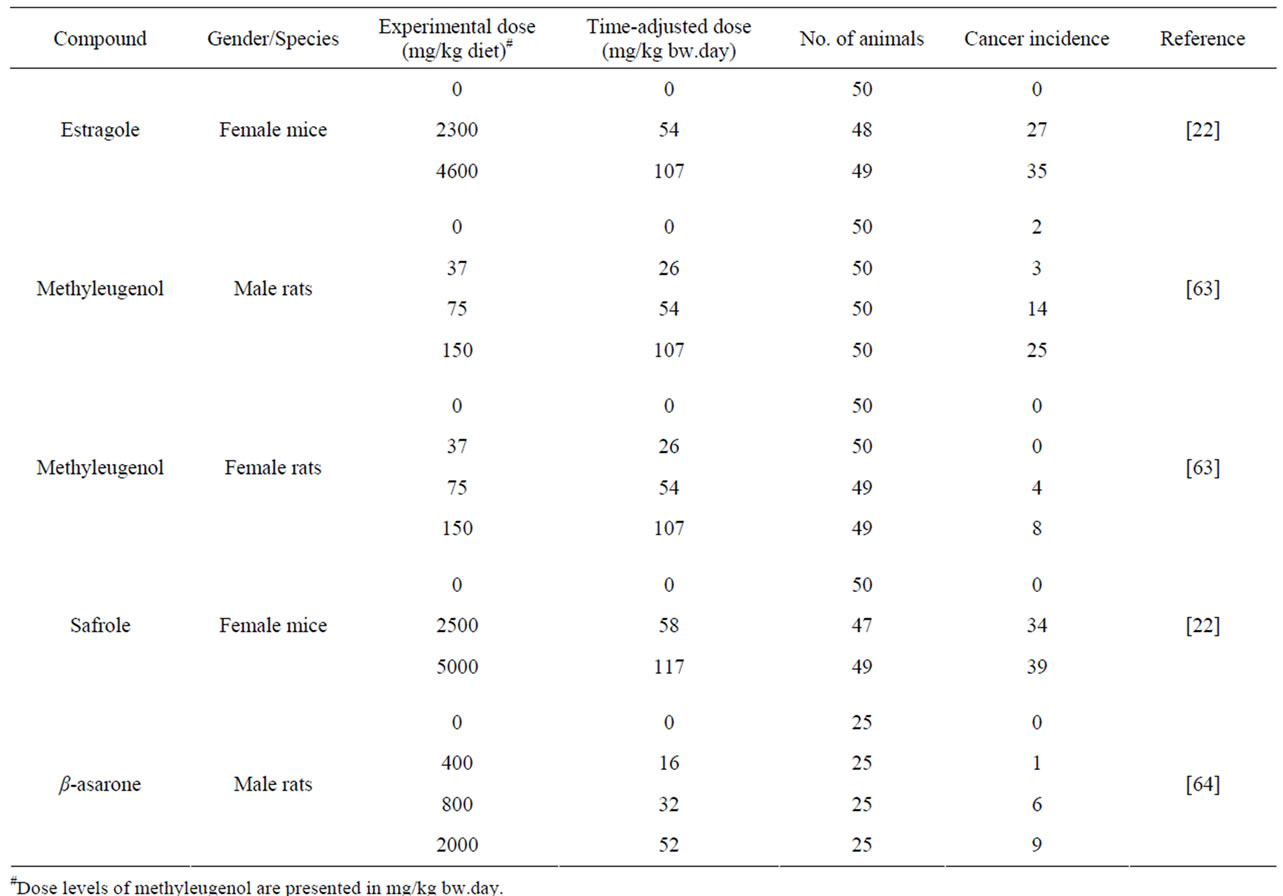
Table 2. Summary of malignant tumors formed after administration to estragole, methyleugenol, safrole or β-asarone.
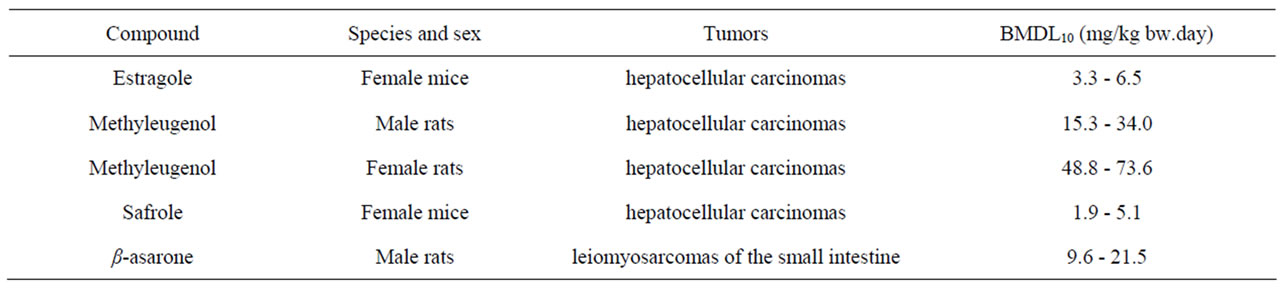
Table 3. Results of a BMD analysis of the carcinogenicity data on the incidence of malignant tumors in rodents exposed to estragole, methyleugenol, safrole, or β-asarone. The data used as input for the BMD analysis are presented in Table 2.
would amount to 0.005 - 186 µg/kg bw.day for a 60 kg person considering the full range of data. For methyleugenol, the daily intake resulting from the use of the same PFS amounts to 0.02 -200 µg/kg bw.day taking into account that the essential oil contains 0.46% - 91.1% methyleugenol [65]. In general, intake estimates for estragole, methyleugenol, safrole and β-asarone, resulting from the use of PFS, range between 0.004 µg/kg bw.day to 2.96 mg/kg bw.day, depending on the botanical of interest, the variable amounts of the genotoxic carcinogens within the plants, and the different recommended daily intakes for the PFS under consideration. When interpreting these intake estimates, it should be kept in mind that all calculations were performed under the assumption that PFS contained the botanical as such and corrections for the manufacturing procedure were not applied. However, when PFS consist of powdered herb material prepared by freeze drying for example, the actual level of the compound of interest may be higher compared to the intake estimates that have been made
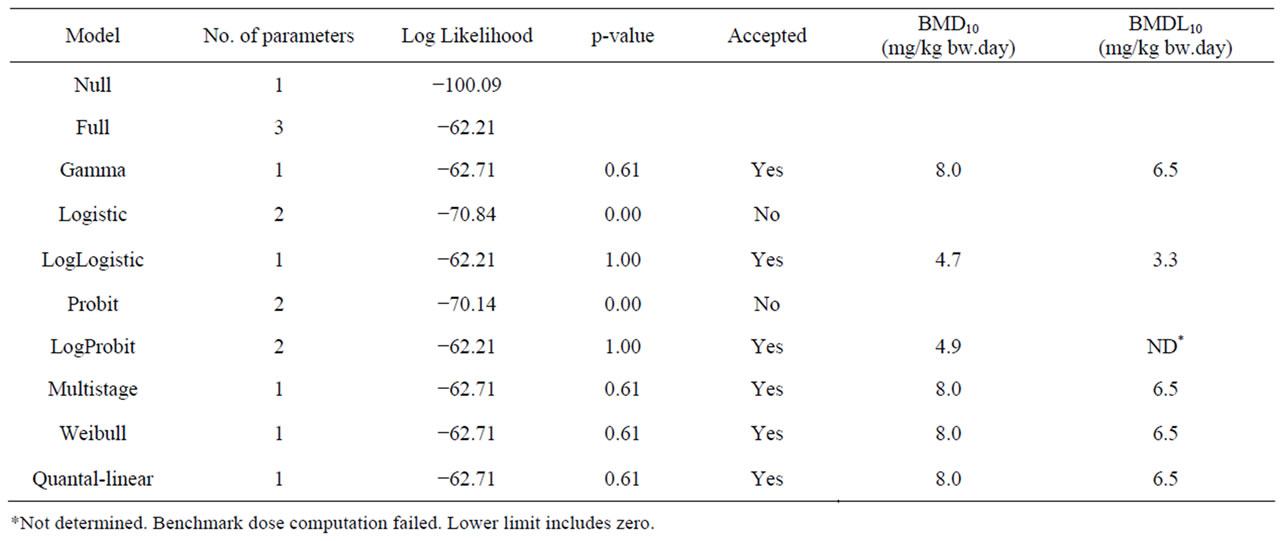
Table 4. Results from a BMD analysis for induction of hepatocellular carcinomas in mice administered 0, 2300 or 4600 mg/kg diet estragole 3 days a week for 12 months [22] using BMDS software version 2.1.2, a BMD of 10% and default settings. The data used as input for the BMD analysis are presented in Table 2.
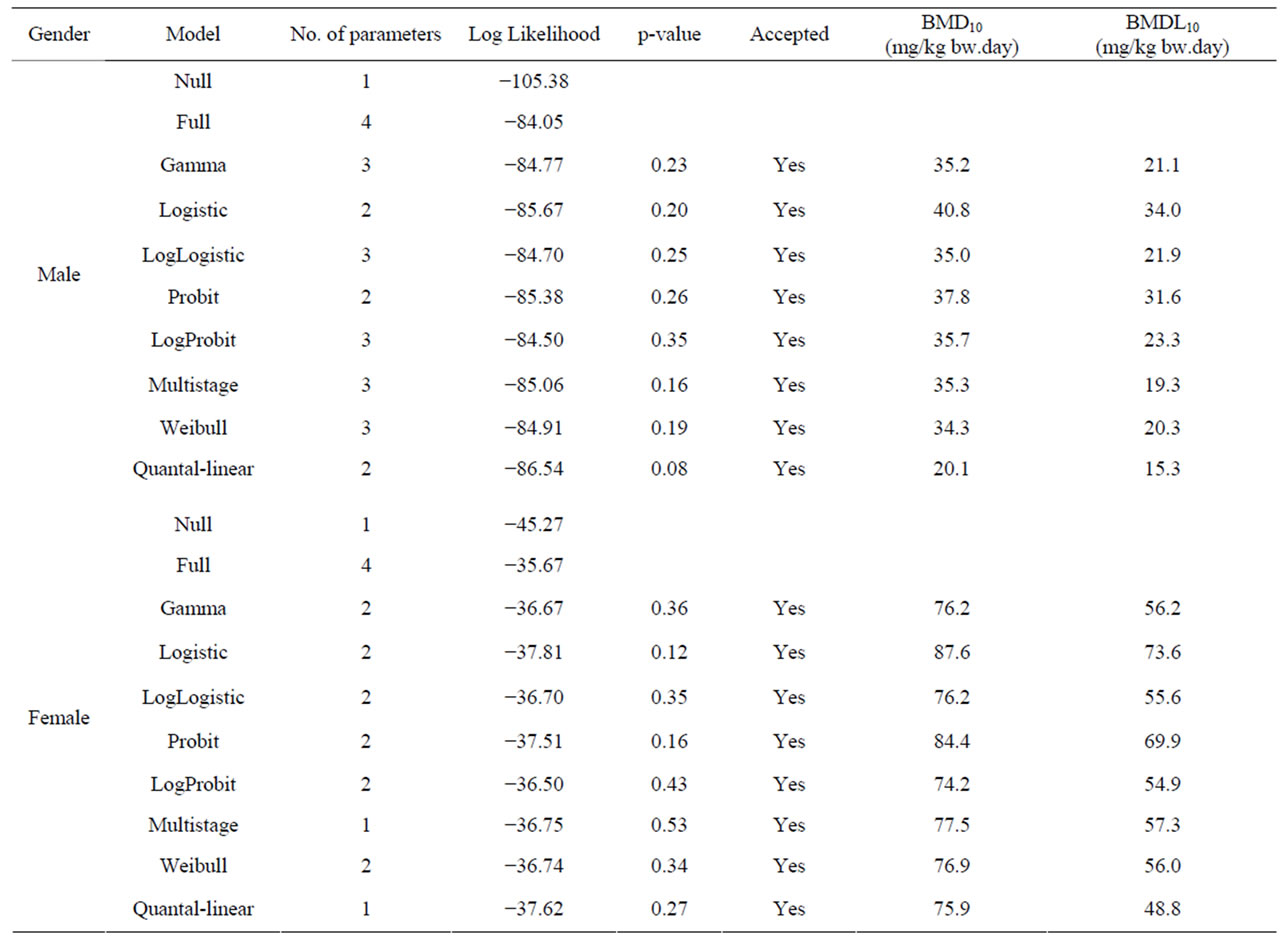
Table 5. Overview of BMD analysis for hepatocellular carcinomas in male and female rats administered 0, 37, 75 or 150 mg/kg bw methyleugenol 5 days per week for 105 weeks [63]. BMDS software version 2.1.2 was used for BMD analysis using a BMD of 10% and default settings. The data used as input for the BMD analysis are presented in Table 2.

Table 6. Results from a BMD analysis for induction of hepatocellular carcinomas in mice administered 0, 2500 or 5000 mg/kg diet safrole 3 days a week for 12 months [22] using BMDS software version 2.1.2, a BMD of 10% and default settings. The data used as input for the BMD analysis are presented in Table 2.
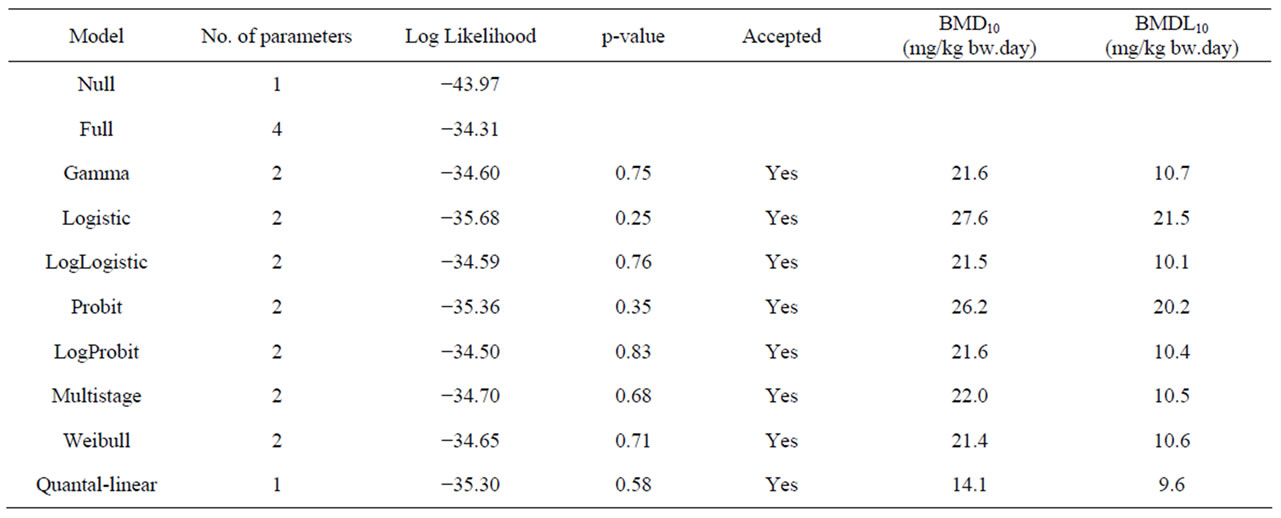
Table 7. Overview of BMD analysis performed using BMDS software version 2.1.2, a BMD of 10% and default settings. BMD10 and BMDL10 values are derived from the incidence of leiomyosarcomas of the small intestine in male rats following a 2 year period of treatment with 0, 400, 800, or 2000 mg/kg diet β-asarone [64]. The data used as input for the BMD analysis are presented in Table 2.

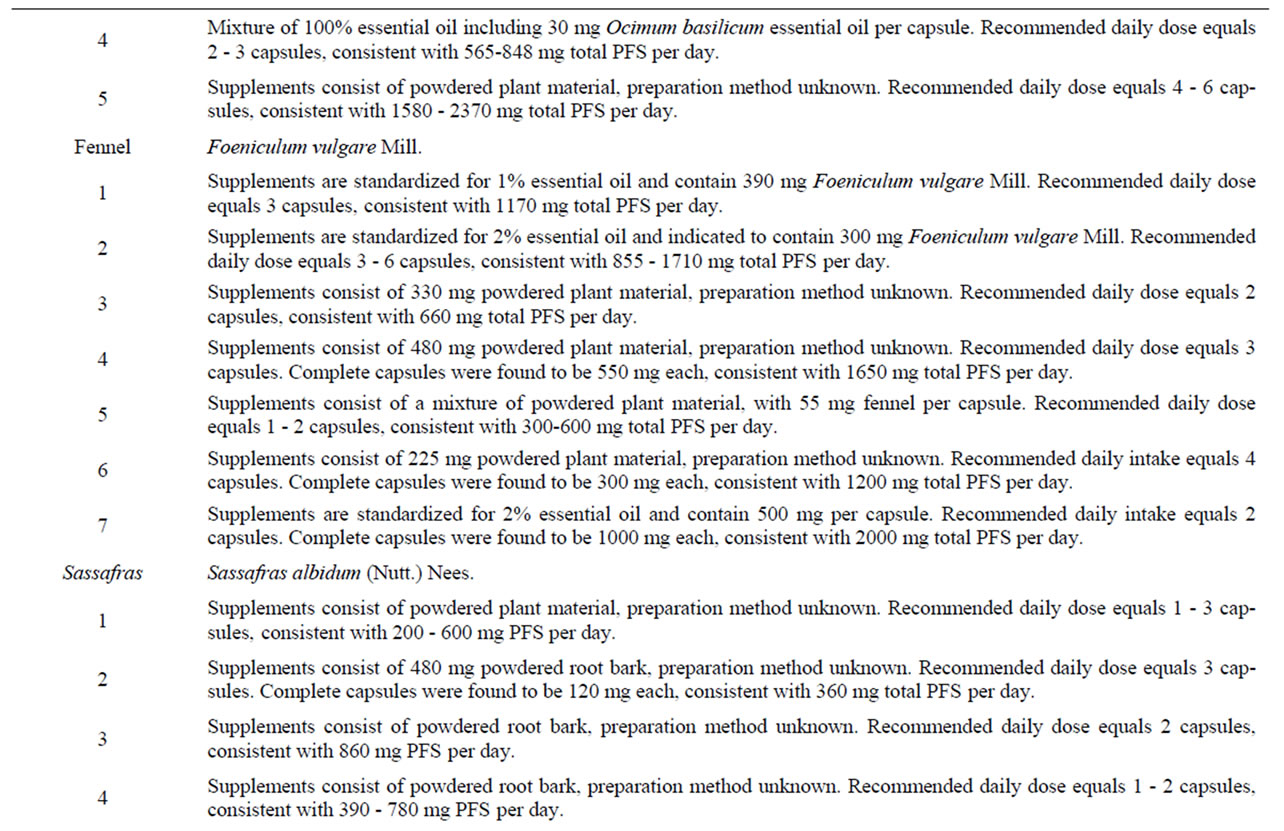

Table 8. Characteristics of PFS used to derive (estimated) daily intakes of estragole, methyleugenol, safrole or β-asarone resulting from the use of the respective PFS.
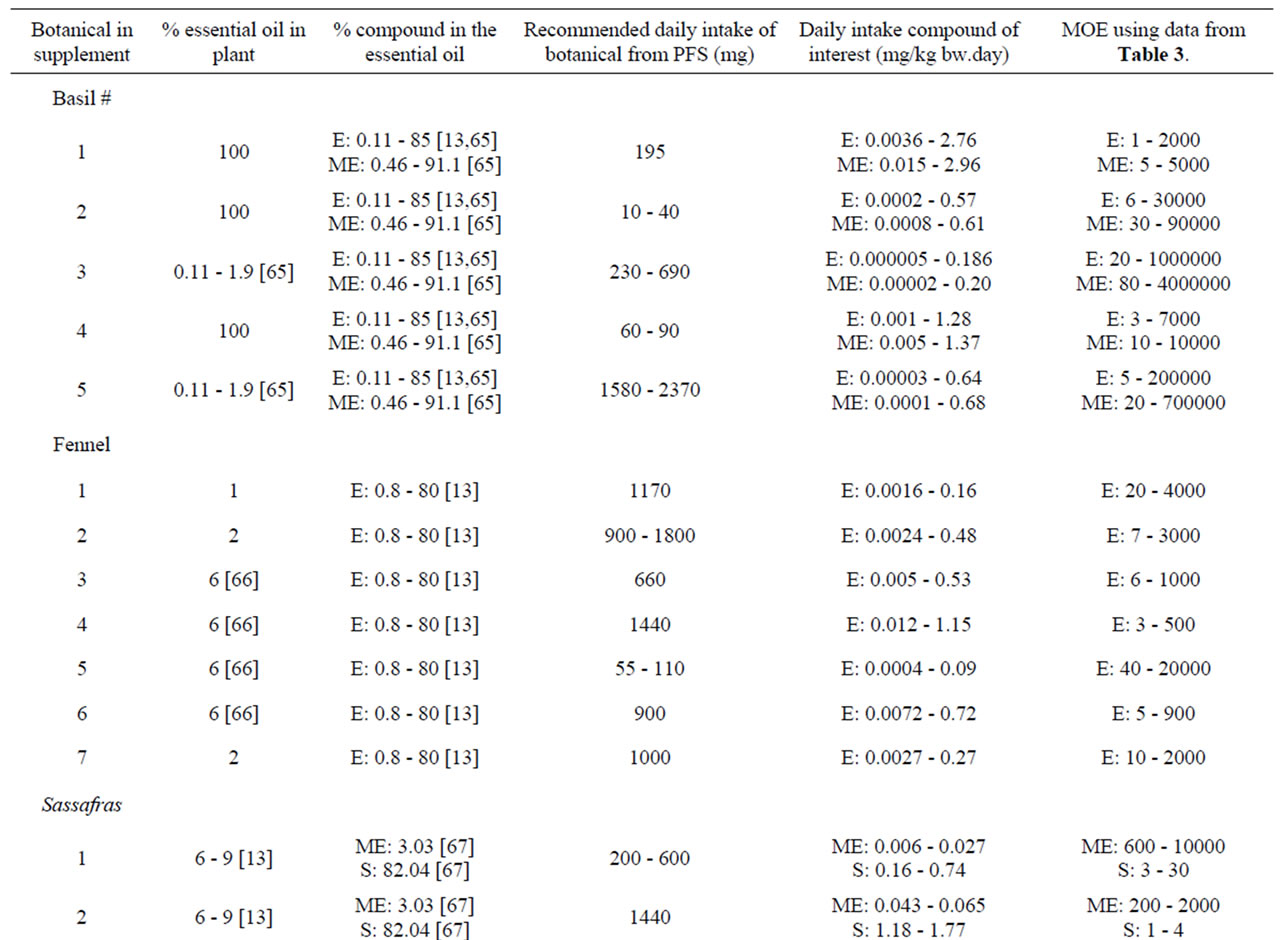
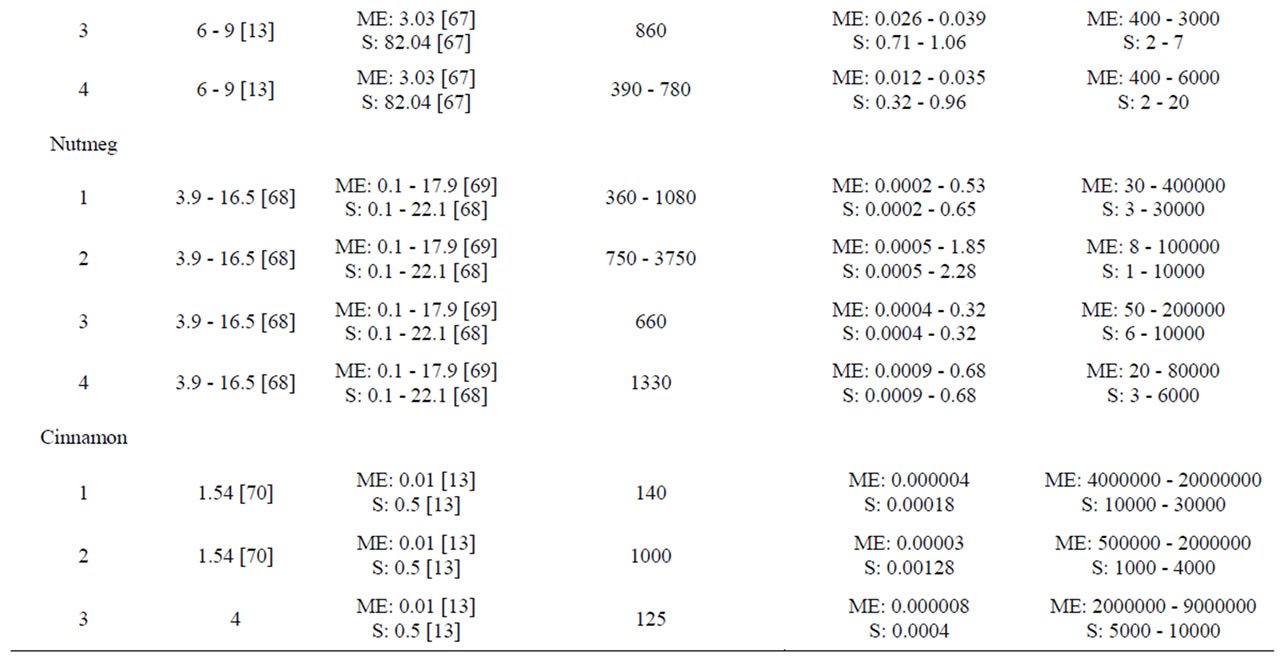

Table 9. Estimated daily exposure to estragole, methyleugenol, safrole or β-asarone present in a variety of PFS. Numbers in square brackets refer to the respective literature references. E estragole; ME methyleugenol; S safrole; BA β-asarone.
for the use of the botanical as such leading to lower MOE values. On the contrary, levels may be lower when the PFS is prepared by decoction. Therefore, in a next step the levels of these alkenylbenzenes in commercial available PFS and the resulting estimated human intakes were quantified based on chemical analysis.
3.3.2. Intake Estimates of Alkenylbenzenes from Consumption of PFS Based on Analytical Quantification
Table 10 shows the results of the quantitative measure ment of estragole, methyleugenol, safrole and β-asarone present in different commercially available PFS for which also theoretical estimations were made. Among the different PFS, estragole was found in a range of 0.07 - 241.56 mg/g PFS corresponding to an estimated daily intake of 0.001 - 4.78 mg/kg bw.day based on the recommended daily intake of the PFS as indicated on the label of the respective supplements. The levels of methyleugenol were equal to 0.34 - 1.04 mg/g PFS in the tested nutmeg-containing PFS. Based on the recommended daily intake of the corresponding nutmeg PFS, the daily methyleugenol intake would equal 0.005 0.065 mg/kg bw.day. For calamus-containing PFS, the amount of β-asarone was found to be in the range of 0.12 - 44.28 mg/g PFS, resulting in a daily intake of β-asarone equal to 0.003 - 0.635 mg/kg bw.day. These daily intake estimates based on chemical analysis of the alkenylbenzene levels in the different PFS are in line with the estimated intake levels of the alkenylbenzenes obtained on the basis of the theoretical estimates for the levels of the alkenylbenzenes in these PFS.
3.4. Margins of Exposure for Alkenylbenzenes
The MOE values obtained by comparison of the BMDL10 values with the estimated daily intake of estragole, methyleugenol, safrole or β-asarone resulting from the use of different PFS, appear to be relatively low (Table 9). For example, upon use of a particular basil containing PFS consisting of the essential oil, the MOE amounts to 1 - 2000 for estragole and a value ranging between 5 - 5000 was found for methyleugenol. For fennel containing PFS, the MOE values range between 3 - 20000 for estragole. Taking into account the consumption of a β-asarone containing calamus PFS, the MOE was found equal to 60 - 7000. Also when considering the actual levels of these compounds in commercially available PFS as quantitatively determined, relatively low MOE values were derived (Table 10). In some cases the MOE was even found to be below 10 which means that the daily intake of the compounds of interest are within the range of the dose levels causing tumors in experimental animals.
4. Discussion
In the presented paper, the MOE approach was used for the risk assessment of a series of compounds that are both genotoxic and carcinogenic and known to be present in botanicals and PFS. In total, 30 botanical ingredients were identified as a possible risk for human health based on their genotoxic and/or carcinogenic characteristics. Out of these selected botanical ingredients 18 compounds appeared to be both genotoxic and carcinogenic. Interestingly, most of these compounds are a member of the group of alkenylbenzenes or unsaturated pyrrolizidine alkaloids. Based on this thorough selection of genotoxic and carcinogenic botanical compounds of interest and the availability of carcinogenicity data allowing the use of the MOE concept, this concept was applied to the alkenylbenzenes estragole, methyleugenol, safrole and β-asarone. The results presented show that the consumption of PFS containing estragole, methyleugenol, safrole or β-asarone may represent a high priority for risk management actions.
Performing the exposure assessment, intake estimates were made based on available literature data. However, the prediction of the content of the botanical compounds of interest in PFS was hampered by uncertainties in the processing techniques used to prepare the respective PFS and varying amounts of these compounds found in plants belonging to the same species depending on the plant maturity at harvest and climatic conditions among others [66]. To determine if the estimates of human dietary
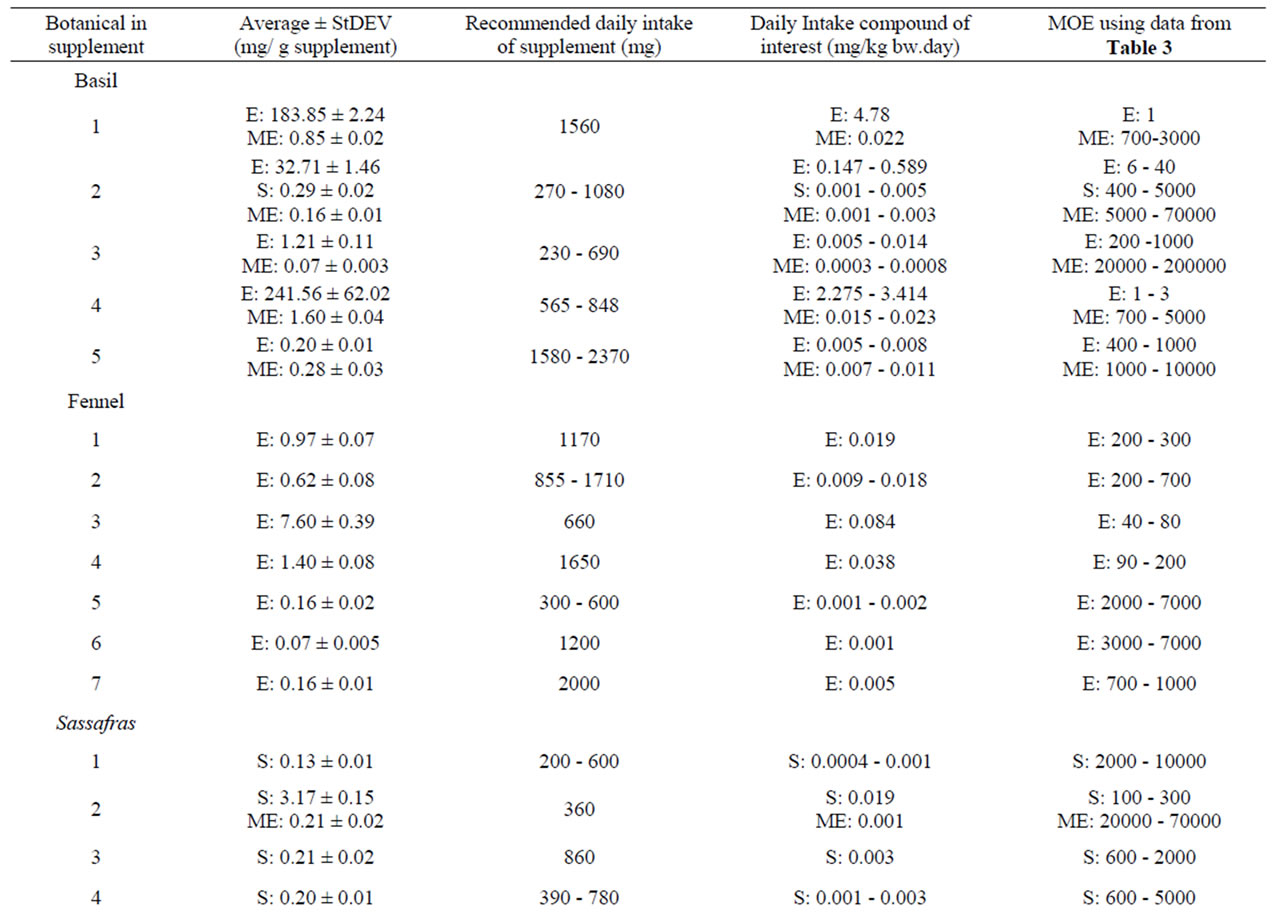
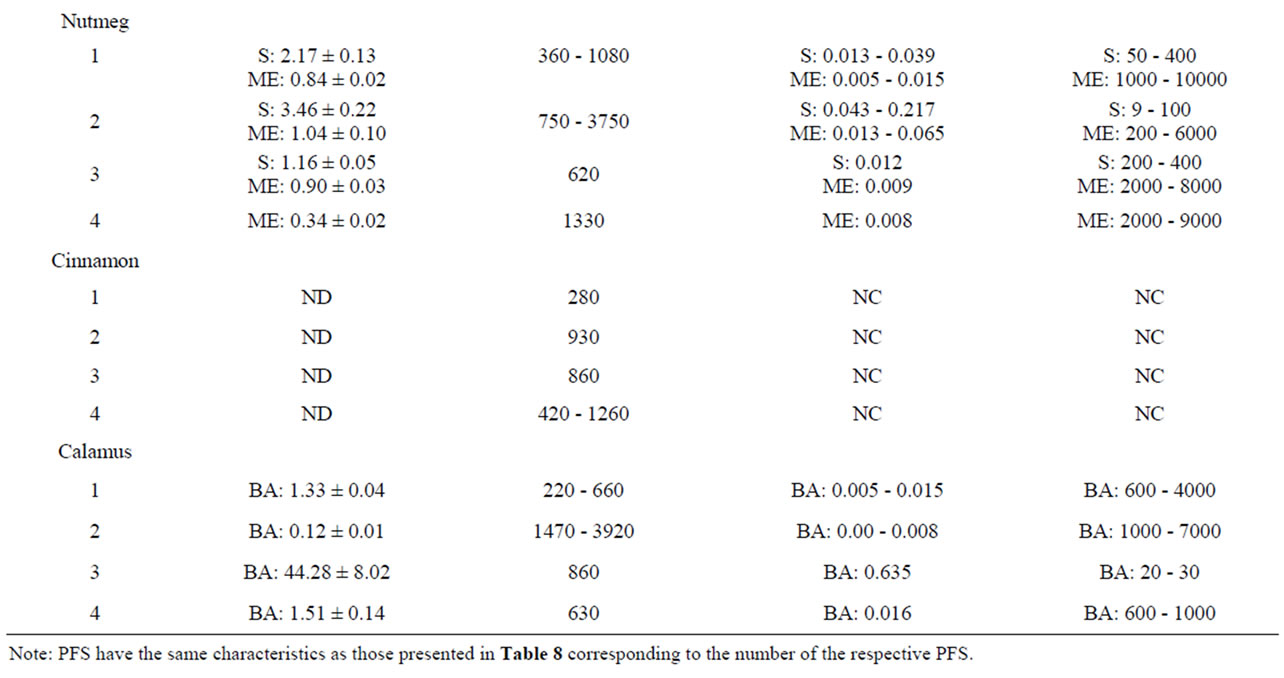
Table 10. Chemically determined levels of estragole, methyleugenol, safrole or β-asarone present in a variety of PFS, corresponding daily exposures and MOEs. ND Not detected (detection limit 0.02 mg/g supplement); NC Not Calculated: E Estragole; ME Methyleugenol; S Safrole; BA β-asarone.
exposure represent the actual levels of the genotoxic carcinogens of interest in commercially available PFS, the content of alkenylbenzenes in different PFS was analyzed. The theoretical estimates made for the levels of estragole, methyleugenol, safrole and β-asarone in the different PFS were generally in agreement with the levels of these compounds actually detected in chemical analysis. In general a wide range of estimated intake levels was obtained for each PFS of interest because of the different amounts of the respective compounds in the plants and their corresponding PFS, resulting in a wide-range of MOE values. Moreover, making use of the levels of the alkenylbenzenes of interest in a particular PFS as determined by chemical analysis resulted also in a wide range of MOEs. It was seen that the wide variations in MOE values for a specific PFS is not due to a large variation in the BMDL10 values, because these values vary only 2 to 3 fold. On the contrary, intake estimates may vary greatly. For example, for a basil-containing PFS, the recommended daily intake equals 1 - 4 tablets (i.e. 270 - 1080 mg/day) and while the daily methyleugenol exposure resulting from the daily use of 1 tablet does not indicate a potential risk for human health, the daily intake of 4 tablets does. As a consequence, it is difficult to express these relatively wide ranging MOEs in terms of concern for human health and this involves an increased risk of misinterpretation, demonstrating the importance of a narrative describing the context of uncertainties in the exposure assessment. The importance of an explanatory narrative was previously indicated [10,17] and will allow a better interpretation of the potential risk for human health.
Based on the analytical measurements it was shown that also between the PFS consisting of the same botanical species, differences occur in the levels of the alkenylbenzenes of interest. The variability in these alkenylbenzene concentrations in the different products can have several causes. The theoretical approach presented in this paper and tested towards a number of PFS available on the market, made heavily clear some points of concern. Alkenylbenzenes are present in the essential oil of the plant. The quantity of the essential oil and the amount of alkenylbenzenes is dependent on genetic factors and geographical influences in cultivation. Further compositional changes are seen depending on the moment of harvesting, the way of drying, processing and manufacturing conditions. Therefore, when manufacturing PFS a strict analytical procedure is necessary in order to determine and follow up the amount of the alkenylbenzenes. In herbal trade, the use of the binomial plant name including the author, the chemotype, variety, hybrid or cultivar should be used and when possible, the geographical origin. In case of using botanicals known to contain genotoxic and carcinogenic compounds, this should be a legal requirement and in fact also be a requirement for all botanicals used in food and food supplements. Basil is an example. “Basil” is an undefined vernacular name. The sweet basil is Ocimum basilicum L. containing often less than 0.2% methyleugenol. However, when cultivated in Egypt the amount increases to an average of 5.6%. O. basilicum var. basilicum contains on average 1.6% methyleugenol and O. basilicum L. var. minimum up to 55% methyleugenol. Both are sold as “basil” or also as Ocimum basilicum L. Even for a morphological well defined species, also different chemotypes exist: “linalool” type, “linalool and estragole” and “linalool and eugenol” all having huge differences in the amount of the alkenylbenzenes. The amount of methyleugenol and estragole also depends on the size of the herb with small plants having a higher amount of methyleugenol and less estragole whereas taller plants showing a higher amount of estragole and lower methyleugenol levels. The moment of harvesting is therefore important as well as the drying methods which heavily influence the content of the volatile compounds of basil [72,73].
The potential risks resulting from the use of alkenylbenzene-containing PFS are especially of concern because multiple supplements might be consumed in addition to the regular daily diet. Previous studies already performed a risk assessment based on the MOE concept for the botanical ingredients estragole [74] and methyleugenol [1,17] indicating that the use of a regular diet containing estragole or methyleugenol is associated with concerns from the human health point of view since low MOEs ranging between 129 - 3300 were found for estragole taking into account the total estimated daily intake [74]. Also for methyleugenol low MOEs were obtained amounting to 42 - 416 [1] and 100 - 800 [17]. Moreover, it is important to recognize that often various structurally-related compounds, causing the same adverse effects, occur together in one botanical such as the alkenylbenzenes estragole and methyleugenol which can be found in sweet basil. However, one should keep in mind that whenever basil (Ocimum basilicum L.) expresses for example high levels of estragole, methyleugenol levels are often low and vice versa [65]. Although the addition of estragole, methyleugenol (Regulation (EC) No 1334/2008 of the European Parliament and of the Council 16 December 2008), safrole and β-asarone (Council Directive 88/388/EEC of 22 June 1988), as pure compounds in food, is prohibited within the EU because of their genotoxic and carcinogenic potentials, currently no restrictions have been made with regard to the use of alkenylbenzene-containing PFS.
Methyleugenol and structurally related alkenylbenzenes are not carcinogenic by nature but metabolic conversion into a biological active form is required to give expression to the carcinogenic capacities in rodent bioassays. It is reported that the metabolic pathway responsible for the formation of a DNA reactive carbonium ion occurs at relatively high intake levels when metabolic conversion through a standard metabolic pathway is saturated [66]. Accordingly, Benford et al. (2010) stated that “derivation of a BMD/BMDL based on modeling of high-dose animal study data does not allow threshold effects in metabolism to be accounted for and thus alters the interpretation of the calculated MOE” [17]. However, a recent study showed only a minor increase (<1 fold) in the relative formation of the ultimate carcinogenic metabolite of methyleugenol at high dose levels as compared to low dose levels [75]. Using a physiologically based kinetic (PBK) model, it was predicted that the formation of the ultimate carcinogen (i.e. 1’-sulfooxymethyleugenol) in rats would correspond to 0.043% of the initial dose at 0.05 mg/kg bw followed by an increase to 0.06% of the dose at a dose of 300 mg/kg bw [75]. These data are also available for the bioactivation of estragole and safrole. For estragole, the formation of the ultimate carcinogenic metabolite was predicted to show a 2-fold relative increase when comparing a low dose (0.05 mg/kg bw) with a high dose (300 mg/kg bw) of estragole [76]. Whereas for safrole, the PBK model predicted that the increase in the relative formation of the ultimate carcinogen with the dose is negligible [77].
5. Conclusions
In conclusion, a number of genotoxic and/or carcinogenic compounds can be found in botanicals and botanical preparations. Especially compounds belonging to the group of alkenylbenzenes and unsaturated pyrrolizidine alkaloids are of possible concern for human health resulting from their genotoxic and carcinogenic potentials. In fact, PFS that are on the market may contain alkenylbenzenes at levels that, at the recommended daily dose of the supplement, may result in a daily intake that is in the range of dose levels causing malignant tumors in experimental animals. While in several countries the use of the alkenylbenzenes estragole, methyleugenol, safrole and β-asarone as flavorings in food products is regulated and prohibited, the use of PFS containing these ingredients in high levels is not regulated. This is remarkable since the use of PFS might result in high exposures to these compounds and consequently low MOE values suggesting a high priority for risk management. It must be emphasized that in the present paper the MOE values are calculated based on BMDL10 values obtained in studies administering high doses of the pure alkenylbenzene compounds to rodents, instead of dosing the botanical as such or in a form of a multicomponent extract. Ideally the studies in rodents should be performed with the botanical or the standardized traditional extract(s) of that botanical. This because studies are already available suggesting that results obtained with the botanical or its extract, containing the genotoxic compound in its food matrix, may be different from those obtained when using the purified genotoxic carcinogenic compounds [66,78,79]. Such differences may occur for example when additional compounds present in the botanical or its extract may modulate the bioactivation and/or detoxification pathways of the genotoxic carcinogen of interest. For example, Jeurissen et al. [79] demonstrated that a methanolic basil extract was able to adequately inhibit the sulfotransferase catalyzed bioactivation and DNA adduct formation in HepG2 human hepatoma cells exposed to the proximate carcinogen of estragole. The basil compound responsible for the inhibition was subsequently identified as nevadensin [80]. These results indicate that the bioactivation of estragole is likely to be lower when estragole is consumed in the presence of other herbal ingredients compared to the exposure of estragole as a pure compound. If such a matrix effect would occur also upon intake of the alkenylbenzenes from PFS, the use of the BMDL10 data obtained in studies with pure estragole, methyleugenol, safrole and β-asarone to calculate the MOE values, would be a worst-case approach and may result in an overestimation of the potential risk for human health. In spite of this it is still concluded that the use of PFS containing the genotoxic carcinogens estragole, methyleugenol, safrole or β-asarone might raise a potential concern for human health and would be of high priority for risk management.
6. Acknowledgements
The research leading to these results has received funding from the European Community's Seventh Framework Programme (FP7/2007-2013) under grant agreement n° 245199. It has been carried out within the PlantLIBRA project (website: www.plantlibra.eu). This report does not necessarily reflect the Commission views or its future policy on this area.