L-Arginine Supplementation and Nitric Oxide Production:No Additional Effect When Associated to Exercise ()
1. Introduction
Several nutritional supplements have been used with the objective of optimizing performance of physically active individuals [1]. Recently, amino acid supplements based on the amino acid L-arginine have been recommended to promote vasodilation due to increased nitric oxide (NO) production in exercising muscle [2]. NO is a gas produced in endothelial cells from L-arginine. After synthesis, NO diffuses rapidly into the smooth muscle cells, where it activates the soluble enzyme guanylate cyclase. In turn, guanylate cyclase converts guanosine triphosphate to cyclic guanosine monophosphate (cGMP). The cGMP activates the calcium pump in the smooth muscle cells, decreasing intracellular calcium concentration and causing smooth muscle relaxation [3-5]. The resulting vasodilation from this relaxation supposedly increases blood perfusion and theoretically promotes a greater nutrient and oxygen supply to the muscle cells during exercise. A secondary effect of increased blood perfusion may be greater metabolite removal, such as that of lactate and ammonia, which may contribute to muscle fatigue during intense exercise [6,7].
During physical exercise, cardiac output increases and blood is redistributed to the active muscles. This adjustment causes an increase in the oxygen supply to withstand the O2 deficit during the initial stage of exercise. Since L-arginine supplementation supposedly stimulates NO synthesis—thereby increasing blood perfusion to the cell tissues—it is of great interest to those who perform aerobic and anaerobic activities: considering that aerobic production of ATP may be quickly activated at the beginning of exercise, resulting in less accumulation of metabolites related to muscle fatigue (e.g., lactate and ammonia).
Some evidence in the literature shows beneficial effects of L-arginine supplementation on the metabolic parameters. Schaefer et al. [8], evaluate metabolic changes of L-arginine supplementation during exercise performed on a cycle ergometer. They observed significantly lower plasma lactate and ammonia, as well as a significant increase in the L-citrulline concentrations (coproduct of NO synthesis). This suggests that part of Larginine was used to synthesize L-citrulline and NO during exercise. Considering that lactate and ammonia accumulation increase hydrogen ion concentrations and causes intramuscular acidity, which may reduce muscle strength and the ability of muscle work [6,7], L-arginine supplementation could be effective in reducing the accumulation of the abovementioned metabolites and thus promote greater ability of muscle work and strength during exercise.
Matsumoto et al. [9], supplemented eight volunteers (4 men) with 2 g of branched chain amino acids (BCAA) + 0.5 g of L-arginine or placebo 10 min before performing the first exercise session. The exercise consisted of cycle ergometer (three sets of 20 minutes) at an intensity of 126 W, which corresponded to 50% of the maximum intensity of work. The authors observed that L-arginine supplementation associated with BCAA resulted in significant suppression of muscle proteolysis induced by exercise when compared to placebo.
It appears that L-arginine can act to reduce muscle fatigue by increasing vasodilation from the increased NO synthesis and thereby removing metabolites related to muscle fatigue. Furthermore, L-arginine may improve muscle recovery by reducing the muscle protein oxidation. Both mechanisms are important in optimizing physical performance. However, it is not still completely clear whether the presumed L-arginine effect on reduction muscle fatigue during exercise is related to an increase in NO synthesis. Therefore, this study aimed to evaluate the effect of L-arginine supplementation on NO production and the possible changes in biochemical parameters related to muscle fatigue in response to exercise.
2. Materials and Methods
2.1. Participants
15 healthy and physically active individuals (including 4 women) with at least one year’s experience in running were recruited to participate in this study. The inclusion criteria were: participants who could run 10 km in less than 45 min (4'30''/km) for men, and less than 50 min (5'00''/km) for women, both in an official race competition. The exclusion criteria of the study were: any presence of cardiovascular, pulmonary or metabolic diseases (hypertension, diabetes, dyslipidemia, smoking, etc.), osteomyoarticular injuries, and/or the use of any kind of nutritional ergogenic or anabolic steroids during the six months prior to the beginning of the study.
All participants were fully informed of the nature and purpose of the investigation and gave their written consent to participate. The experimental procedures were performed in accordance with the ethical standards of the Helsinki Declaration and were duly approved by the Institutional Ethics Committee.
2.2. Experimental Design
The study was conducted in a randomized, double blind and placebo-controlled manner. On the day of the study, after an overnight fast, blood samples were collected for baseline measurements (PRE). After blood collection, the participants were divided into the L-arginine supplemented group (ARG) or placebo supplemented group (PLA) via simple randomization by an individual outside the research group. Thirty minutes after supplementation, volunteers performed two bouts of a running exercise tests. The recovery period between the exercise tests was 10 minutes. Blood samples were also collected immediately after the first running exercise test (T1), immediately after the second running exercise test (T2), and after 20 min of rest (T3) (see Figure 1). The experimental procedures were performed between 06:00 and 09:00 am. The participants were instructed to not perform physical exercise 24 hours before the beginning of the study.
2.3. Supplementation
All participants orally consumed a single dose of 6 g of either L-arginine hydrochloride or cornstarch as placebo (12 caplets containing 500 mg each) in 500 mL of water. Based on previous studies, the total dose of L-arginine offered in this study are well tolerated and safe for human health [2,3], as well as sufficient to elevate plasma L-arginina concentrations [2] and promote hemodynamic

Figure 1. Experimental design of the study.
changes [10].
2.4. Exercise Protocols
The two running exercise test were 5 km time-trials (5 km-TT). In each test, the participants were instructed to run the 5 km as fast as possible. The recovery period between tests was 10 min. All the participants were familiarized with the exercise protocol before the beginning of the study. The exercise tests were conducted in the morning and were accompanied by experienced and registered physical education professionals.
2.5. Blood Samples Analysis
Blood samples were collected in vacuum tubes containing EDTA and centrifuged immediately at 3000 g for 10 min at 4˚C for plasma separation and stored at −80˚C for subsequent analysis.
NO production was assayed by measuring plasma cGMP. For this analysis, plasma was firstly treated with ethanol and the precipitate was removed by centrifugation at 1500 g for 10 minutes. The supernatant was concentrated using a vacuum sample concentrator (Savant SpeedVac Concentrator, Thermo Fisher Scientific Inc.) and subsequently resuspended in deionized water. From this stage, cGMP quantification was performed using an enzyme immunoassay kit (Cayman Chemical Company, Michigan, USA) following the manufacturer’s guidelines.
Plasma lactate and ammonia concentrations were analyzed by a colorimetric method using commercially available kits (Abcan Inc. Cambridge, UK). These analyses were also performed according to the manufacturer’s instructions. For all analyses, the reading was performed using a microplate reader (VICTORTM X4 Multilabel Plate Reader, Perkin Elmer, CA, USA). The coefficient of variation (n = 4, in triplicates) of the methods for analysis of cGMP, lactate and ammonia were respectively 7.7%, 5.4%, 6.5% to intra-assay and 11.2%, 9.4%, 10.1% to inter-assay.
2.6. Statistical Analysis
The assumptions of normality, homogeneity of variances, and sphericity were examined with the Shapiro-Wilk, Levene and Mauchly tests, respectively. A two-way analysis of variance with repeated measures on two factors (2 × 4; group × time) was used to identify differences in cGMP, lactate and ammonia between the groups ARG and PLA and between time (T0, T1, T2 and T3). When F was significant, an additional post hoc test with Bonferroni adjustment was perfomed. Data were considered statistically significant at a confidence level of P < 0.01. All analyzes were performed using commercially available statistical package (IBM SPSS statistics version 20 for Windows, Chicago, IL, USA).
3. Results
The normality, variance homogeneity and sphericity assumptions of the data were not violated in either the ARG or the PLA group.
3.1. Baseline Volunteers Characteristics
At baseline, no significant differences were observed with respect to age (ARG: 36.8 ± 7.1 vs. PLA: 30.6 ± 9.5 years), height (ARG: 172.3 ± 10.6 vs. 175.0 ± 7.5 cm), body weight (66.7 ± 14.4 vs. 68.1 ± 10.6 kg), body mass index (22.2 ± 2.6 vs. 22.1 ± 2.2 kg/m2), and total running time in official competition of 10 km (43.9 ± 4.3 vs. 43.0 ± 3.9 min).
3.2. NO Production
The plasma cGMP concentrations during the study period are shown in Figure 2. A significant increase in plasma cGMP in the period immediately after the two 5 km-TT tests (T1 and T2) in both groups ARG (T0: 3.6 ± 1.4; T1: 17.9 ± 5.8; T2: 15.9 ± 5.3; T3: 7.3 ± 2.5 pmol/mL) and PLA (T0: 4.1 ± 1.1; T1:18.8 ± 9.9; T2: 16.1 ± 3.5; T3: 9.3 ± 3.7 pmol/mL) when compared to T0.
3.3. Lactate and Ammonia
Plasma lactate and ammonia concentrations are described in Table 1. Lactate and ammonia increased significantly in response to exercise (T1 and T2) in both ARG and PLA groups when compared to T0. However, only in the ARG group were both lactate and ammonia concentrations significantly decreased during recovery (T3) when compared to T1 and T2. Despite this reduction, no significant difference between the groups was observed.
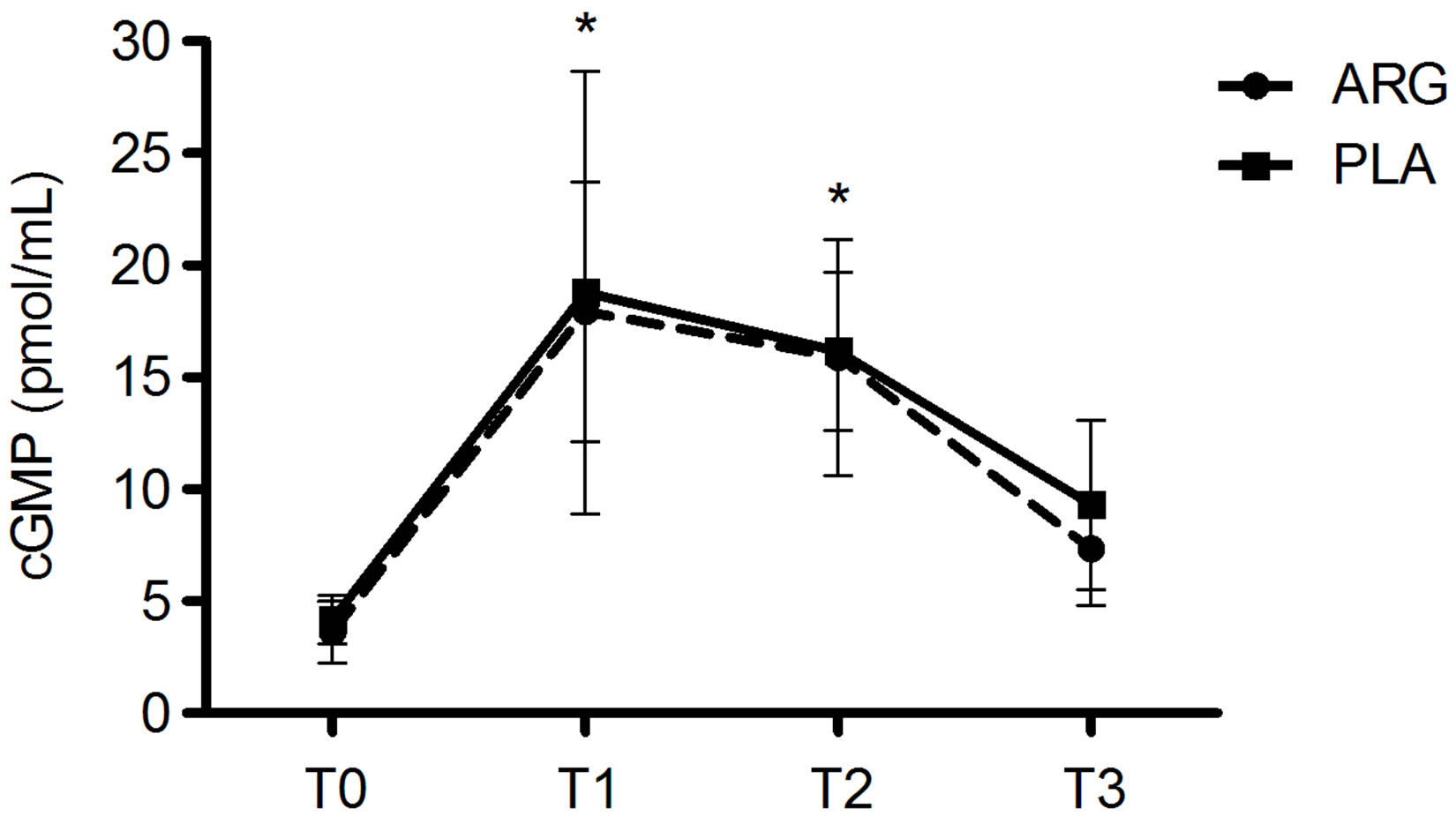
Figure 2. Plasma concentrations of guanosine monophosphate (cGMP) over the time points of the study. T0 = baseline; T1 = immediately after the first exercise test; T2 = immediately after the second exercise test; T3 = after 20 min of rest; ARG = L-arginine supplemented group; PLA = placebo group. The symbol *(P < 0.01) denotes significantly different from T0.

Table 1. Plasma concentration of cGMP, ammonia and lactate over the time points of the study in both the ARG and PLA groups.
4. Discussion
The present study was designed with the general purpose of investigating whether the supplementation of the amino acid L-arginine could be a nutritional strategy to increase NO synthesis, and thereby reduce biochemical parameters related to muscle fatigue, such as lactate and ammonia.
The main finding of this study was that plasma cGMP concentrations increased significantly in both ARG and PLA groups in response to exercise. However, no significant difference was observed between the groups. Furthermore, no significant difference was observed in plasma lactate and ammonia concentrations between the groups.
NO synthesis is totally dependent upon the enzyme group, called nitric oxide synthase (NOS). These enzymes catalyze NO and L-citrulline production from L-arginine. NO is a highly reactive molecule produced endogenously in the gas form and is distinguished by its effect on vascular tone modulation [11]. Once released, NO diffuses rapidly from endothelial cells to the smooth muscle of blood vessels. In smooth muscle cells, NO activates the enzyme guanylate cyclase, which in turn converts guanosine triphosphate to cGMP. The cGMP promotes the activation of the calcium pump within the smooth muscle cell, decreasing intracellular calcium concentrations. In this manner, the increase of intracellular cGMP induces smooth muscle relaxation and thus vasodilation. Therefore, measuring cGMP may be an effective method of evaluating the relationship of plasma NO levels on vascular tone. Böger et al. [3] and Bode-Böger et al. [4,5] observed significant increases in cGMP concentration in urine after intravenous L-arginine administration. Furthermore, after oral L-arginine supplementation, Lucotti et al. [12] also observed a significant increase in plasma cGMP concentrations. This data indicates that the endogenous NO synthesis was increased after L-arginine administration both orally and intravenously.
Contrary to these aforementioned authors, in the present study no significant difference in plasma cGMP concentrations between ARG and PLA groups were observed. One possible explanation for the absence of differences between the groups may be the supplementation coupled with exercise. It is known that L-arginine is the sole substrate to synthesize NO from nitric oxide synthase (NOS). However, the shear stress or friction caused by the blood flow through the vessels also activates NOS, and consequently stimulates NO synthesis regardless of Larginine. Therefore, exercise is an important stimulus for endothelial NO synthesis. Based on this theory and the results of this study, it appears that L-arginine supplementation has no additional effects on NO synthesis when associated with running exercise. However, Bailey et al. [13] observed a significant increase in plasma nitrite concentrations (an indirect indicator of NO synthesis) after supplementing healthy and physically active subjects with L-arginine (6 g) and other amino acids (L-glutamine, L-leucine, L-valine, L-carnitine, L-citrulline, L-isoleucine and L-cysteine) together with cycle ergometer exercise sessions for 3 days. However, the Bailey et al. [13] study has an important methodological limitation: the authors used nitrite as an indicator of NO production. Since many foods consumed in the human diet are sources of nitrite and nitrate, the plasma concentration of nitrite may vary significantly if the diet is not controlled. The volunteers of the study conducted by Bailey et al. [13] were not instructed to avoid consumption of rich nitrite and nitrate foods. Therefore, the significant increase in plasma nitrite observed by the authors may have been influenced by an uncontrolled diet.
With respect to the biochemical indicators of muscle fatigue, there was a significant increase in plasma lactate and ammonia in both groups immediately after the two 5 km-TT. Although this was an expected physiological response, this increase suggests that there was an imbalance between production and removal of plasma lactate and ammonia as the exercise intensity increased, which induced high levels of these metabolites in the blood. After 20 minutes of recovery (time T3), the plasma lactate and ammonia concentrations only reduced in the ARG group. This reduction was significant when compared to the period after the two 5km-TT. However, no significant difference was observed between groups.
Although some studies have shown the positive effect of L-arginine supplementation in reducing exercise-induced lactate [8,14,15] and ammonia [16,17] accumulation, none of these studies attempted to measure NO synthesis. Therefore, no conclusions can be drawn associating the reduced plasma lactate and ammonia with the possible effect of L-arginine in stimulating NO synthesis. Furthermore, since no additional effect of L-arginine supplementation on NO synthesis was observed in the present study, no beneficial effect of supplementation in reducing plasma lactate and ammonia concentration could be expected.
It is important to reinforce the theory that physiological L-arginine concentrations in healthy subjects are sufficient to saturate the endothelial NOS enzyme (MichaelisMenten constant is approximately 3 mmol/L). Therefore, supplementation with the substrate L-arginine should not necessarily increase NOS enzyme activity and, therefore, no additional NO production should occur.
5. Conclusion
Nutritional supplementation with 6 g of L-arginine did not promote additional effects on NO synthesis when associated with running exercise. Furthermore, the supplementation did not reduce the accumulation of plasma lactate and ammonia induced by exercise. Based on these results, the nutritional supplements containing the amino acid L-arginine should not be recommended as a nutriational ergogenic aid to improve NO synthesis in healthy and physically active subjects.
6. Acknowledgements
The authors thank Ricky Toledano for preparing the English version of the manuscript and The Research Foundation of the State of Rio de Janeiro-FAPERJ by provided financial support for the study (grant No. E- 26/100.499/2012 and E-26/100.500/2012).
NOTES