1. Introduction
Microbes are living organisms, which can adapt to antimicrobial systems applied to control them during food processing and storage. Mechanisms to develop resistance include the modification of the genetic pool by spontaneous mutation or acquisition of foreign fragments of DNA. However, cell adaptation and survival do not always imply modification of the organisms genetic makeup, as it is the case with antibiotic resistance for instance. At the molecular level, stress proteins may be induced as a result of prior exposure to sublethal physical or chemical stresses, such as heat and acid. Various stresses also generate large amounts of unfolding and misfolding proteins which will induce the synthesis of numerous chaperones and proteases to restore homeostasis [1]. Molecular chaperones, like DnaK, are present during non-restricted growth but their role becomes more crucial for cell damages repair under inimical conditions, such as heat shock and oxidative stress [2].
In this context, cell counts alone cannot properly assess the efficacy of antimicrobial treatments for controlling microbial contamination in foods. Previous work has demonstrated that heating can induce a higher intracellular concentration of DnaK which allows Escherichia coli cells to better resist a subsequent, more severe stress [3]. Lemay et al. [4] also demonstrated that cell survival may vary according to the order in which antimicrobial treatments are applied. Lactobacillus alimentarius cells had a higher survival rate when they were exposed to sublethal osmotic shock (NaCl) prior to acid stress (citric or glutamic acid) compared to the reverse. The lowest survival was obtained when the treatments were applied simultaneously. Hence, a deeper understanding of the physiological state of microorganisms and their response to stress is necessary to evaluate the efficacy of antimicrobial systems used in foods.
DnaK and its co-chaperones (DnaJ and GrpE) are involved in many cellular processes including folding of nascent polypeptides notably during their biosynthesis and translocation through membranes, repair of denatured proteins and degradation of non-functional ones [5,6]. DnaK is also known as a heat shock protein because its expression is increased to higher intracellular levels in response to heating [7]. Its protective role during cell adaptation and survival to heat shock is well documented [8]. Heat shock proteins have also been demonstrated to be induced by other stresses, such as exposure to ethanol, UV radiation and DNA gyrase inhibitors [9], including gamma rays in eukaryotic [10] and prokaryotic cells [11]. Furthermore, cross protection between different stresses has also been observed [4,12]. Heat is detrimental to cells due to protein denaturation. The denatured proteins are inactive and no longer able to sustain their functions or meet the cell’s metabolic needs. Gamma irradiation, on the other hand, causes DNA damages by direct ionization of molecules and the production of hydroxyl radicals [13]. Nevertheless, several proteins, such as DNA glycosylases and RecA, B, C, D, are involved in DNA repair to help the cell survive irradiation [14]. The maintenance of these important proteins in their active form is, therefore, essential for cell survival and the chaperones, like DnaK, may be induced differently according to the irradiation treatment.
Food irradiation is an effective method to reduce food borne pathogens, to prevent spoilage and to increase shelf life [15,16]. A better understanding, at the molecular level, of the bacterial stress response generated by irradiation treatment is important to assess its efficacy. In this study, the effect of similar doses of γ-rays applied at different rates on the viability of E. coli cells was evaluated. Intracellar DnaK concentration was also measured as a physiological biomarker.
2. Materials and Methods
2.1. Bacterial Strain and Growth Conditions
Stock cultures of Escherichia coli ATCC 25922 (clinical isolate, FDA strain Seattle 1946) were stored at −80˚C in brain heart infusion broth (BHI; Difco Laboratories, Detroit, MI) supplemented with 20% glycerol as cryoprotectant. Prior to the experiments, the frozen cultures were thawed and subcultured (1% v/v) daily in BHI broth for a minimum of 2 and a maximum of 7 days. Cultures were incubated overnight at 37˚C. Cell enumeration was performed by plating serial dilutions (1:10) onto BHI agar. Colony forming units were counted after incubation at 37˚C for 24 h.
2.2. Irradiation Treatment
E. coli cells to be irradiated were inoculated at a concentration of 1% (v/v) in 200 ml of BHI broth and grown at 37˚C to an OD600 of 0.5. Cultures were gently stirred during irradiation treatment in static air, at ambient temperature (23˚C) with g-rays (Co60, Gammabeam 651 PT #3, MDS Nordion, Kanata, ON). The radiation dose was monitored by dosimetry using a MSD-Nordion cericcerous dosimeter (GFS-X2-03) for a dose range of 0 - 10 KGy. A final dose of 0.3 KGy of g-rays, without shielding, was selected and applied either at a low (3 × 10−3 KGy/min) or high rate (8 × 10−2 KGy/min).
2.3. Determination of Intracellular DnaK by Competitive ELISA
Intracellular DnaK concentration was determined immediately after the irradiation treatment and after 22 h of recuperation at 37˚C according to the procedure described by Seyer et al. [3]. Briefly, cells from the 200 ml culture were collected by centrifugation (13,000 g, 15 min), washed and resuspended in 0.1 M NaHPO4 buffer at pH 7 prior to sonication (Sonic Dismembrator model 300, Artek Systems Corp.). The sonicated suspension was centrifuged and the supernatant frozen at −80˚C until the DnaK concentration was determined by a competitive ELISA as previously described [17]. Microplates were coated (100 µl/well) with purified DnaK, (0.25 µg/ml, Assay Designs, Inc., Ann Arbor, MI) prepared in 0.1 M NaHCO3 at pH 9.6 and incubated at 4˚C overnight. The microplates were washed four times with phosphatebuffered saline (PBS) supplemented with 0.05% (v/v) Tween 20, and blocked with 200 µl/well of PBS-1% (w/v) bovine serum albumin solution (PBS-BSA) for 1 h at room temperature (22˚C). Coated wells were then washed four times with PBS-0.05% Tween solution. For the competition step, 150 µl of a mouse anti-DnaK monoclonal antibody (1 mg/ml; Assay Designs, Inc.) was diluted to 1:10,000 in PBS-1% BSA-0.05% Tween 20 and mixed with 150 µl of the supernatant to be tested for 1 h at room temperature (22˚C). The antibody solution (100 µl) was then added to the coated well. After incubating for 2 h at 37˚C, the plates were washed four times with PBS-0.05% Tween solution and 100 µl of a sheep antimouse immunoglobulin G-peroxydase conjugate (5 mg/ ml; Medicorp Inc.) was added per well. The plates were incubated for 90 min at 37˚C. They were washed four times with PBS-0.05% Tween solution and tetramethylbenzene (Medicorp Inc.) was added for colorimetric reaction. After 40, 50 and 60 min of reaction, absorbance was read at 370 nm (PowerWaveX, Bio-Tec Instruments, Inc.). A standard curve was prepared with purified DnaK diluted in PBS-1% BSA-0.05% Tween 20 solution and DnaK concentration was expressed as molecules/cell since the molecular weight of DnaK (70 KDa) and the initial cell number (cfu/ml) are known. All measurements were repeated three times and means of intracellular DnaK concentrations were calculated from these biological repetitions.
2.4. Statistical Analysis
Results are expressed as mean ± standard error of three replicates. Statistical analysis was performed using the Statview 5.0 software (SAS Institute Inc., Cary, NC). Homogeneity of the variance between groups was assessed by the Bartlett test. The global effect of irradiation treatments on cell counts and DnaK concentrations were tested by a one-way analysis of variance (ANOVA) and differences between non-treated and irradiated cells (i.e. low and high rate, immediately after treatment or after recuperation) were then investigated by a Student-Newman-Keuls test. All tests were performed with a 5% significance level.
3. Results and Discussion
3.1. Sensitivity of E. coli to Irradiation
E. coli is not particularly resistant to γ-irradiation [18]. A D10 value of 0.21 KGy was reported in broth, meaning that the cell count will decrease by one log unit with an irradiation dose of 0.21 KGy [19]. In low and high fat beef, D10 values of 0.43 and 0.42 KGy were reported, respectively. For Clostridium botulinum, D10 values from 1.29 to 5.9 KGy were reported [19]. A dose of 1.0 to 2.3 KGy is usually sufficient to control E. coli contamination in foods [20]. Sublethal conditions are most favorable to induce bacterial stress response and cell adaptation. They refer to treatments that are not severe enough to annihilate the target microorganism. The lowest radiation dose, which could technically and consistently be generated with the Co60 system used without shielding, was 0.3 KGy.
3.2. Effect of Irradiation on Cell Counts
The treatment at 0.3 KGy, both at low (3 × 10−3 KGy/ min) and high (8 × 10−2 KGy/min) irradiation rates, significantly reduced initial cell counts from 8 log cfu/ml to 4.1 log and 3.8 cfu/ml, respectively (P < 0.0001; Figure 1).
No difference in cell counts was observed between low and high radiation rates either immediately after irradiation or after 22 h of recuperation at 37˚C (P > 0.05). Hence, cells never resumed active growth after irradiation treatments under the conditions tested. For E. coli prepared under similar conditions and exposed to a heat treatment of 55˚C for 105 min, cells were still able to resume growth after 12 h of recuperation to reach 7.06 log cfu/ml [3]. These discrepancies between heat shocks and γ-irradiations suggest that the severity of different antimicrobial systems influences cell recovery. Stressed and injured cells must first overcome their cellular damages before they can return to active cell division and growth to a point where increase in cell counts can be observed again.
3.3. Effect of Irradiation on the Intracellular Concentration of DnaK
When cells were grown at 37˚C to mid-log phase and then irradiated at 0.3 KGy using a high (8 × 10−2 KGy/ min) or low (3 × 10−3 KGy/min) rate, intracellular DnaK concentration increased significantly from 38,500 ± 3496 molecules/cell to 76,825 ± 8864 and 105,416 ± 5717 molecules/cell, respectively (P = 0.0001; Figure 2). However after recuperation, DnaK concentration of cells irradiated at a low rate decreased significantly from 105,416 ± 5717 molecules/cell to reach the same level (67,704 ± 4711 molecules/cell) as for the cells irradiated at high rate with (76,764 ± 6875 molecules/cell) or without recuperation (76,825 ± 8864 molecules/cell). Interestingly, intracellular DnaK concentration measured after 22 h of recuperation at 37˚C remained higher than in the control cells independently of the irradiation rate (P < 0.05). This result suggests that irradiated cells may conserve a stress memory symbolized by higher amounts of DnaK protein. Seyer et al. [3] demonstrated that an increase in DnaK concentration provided a higher resistance to a lethal heat treatment. Exposure to weak magnetic field is also known to induce synthesis of Heat
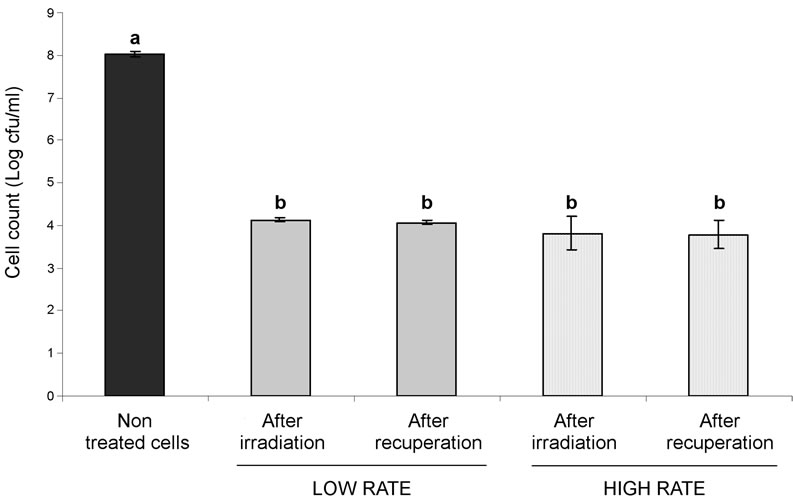
Figure 1. Cell counts of E. coli ATCC 25922 before and after irradiation (0.3 KGy) at low or high rate and after 22 h of recuperation at 37˚C. Means (Log cfu/ml) were obtained from three biological repetitions performed in duplicate. Means ± standard error with the same letter do not differ significantly (P > 0.05).
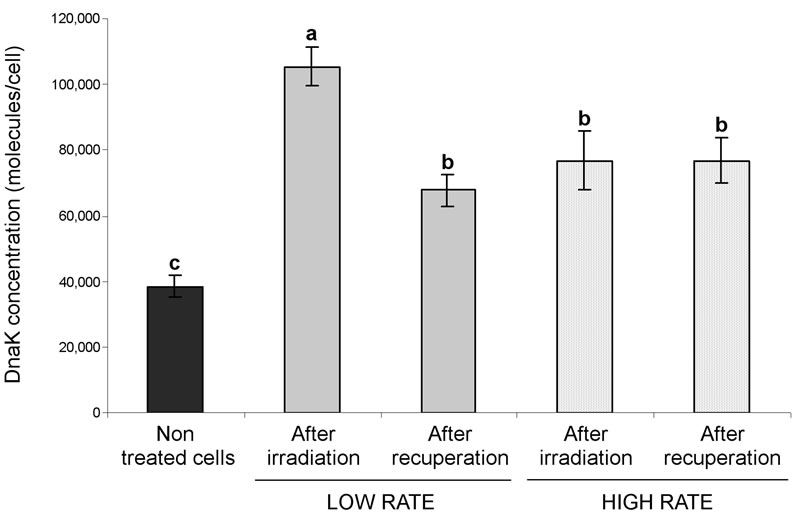
Figure 2. Intracellular DnaK concentration after irradiation at 0.3 KGy followed, or not, by 22 h of recuperation at 37˚C. Means ± standard error were obtained from three biological repetitions of three independent measurements. Detection limit corresponds to 1500 molecules/cell. Means with the same letter do not differ significantly (P > 0.05).
Shock Proteins, like DnaK/J and GroEL [21,22] and to enhance bacteria UV resistance [23]. Nonetheless, the selective development of a more resistant subpopulation is also a possible explanation.
The ELISA test performed in our laboratory revealed an increase of nascent and correctly folded DnaK proteins remaining in exponentially growing and irradiated cells. Using Western blot analysis after protein denaturetion (SDS-PAGE), Caillet et al. [11] were also able to demonstrate that DnaK expression is induced in E. coli O157:H7 strain EDL933 grown to stationary phase with irradiation doses of 0.4, 1.1 and 1.3 KGy. Such doses were obtained in 5.2, 14 and 19 min, respectively at a constant irradiation rate of 4.6 KGy/hour. The first dose studied was used to generate damaged cells but cell count reduction by such treatment was not mentioned. Upon heat treatment, protein denaturation causes cell injury and death, whereas γ-irradiation damages DNA structure. Proteins, such as RecA, Fpg and other proteins of the SOS pathway, are important for DNA repair [14]. Induction of a higher level of chaperones following γ-irradiation might be important for maintaining the functionality of these proteins and for improving cell adaptation and survival.
4. Conclusion
Simply looking at the cell counts, one would be tempted to conclude that the same irradiation dose applied at different rates produces a similar effect on the irradiated cells whereas the intracellular DnaK concentration rather suggests that although at a similar number, the cells are not in the same physiological state after irradiation for cells treated at a low irradiation rate. Our quest to find suitable stress proteins for food process validation is still underway. At this time, we do not know if a single general protein, or many, is required to accurately determine the efficacy of the food processes currently used in industry.
5. Acknowledgements
The authors thank Mr. Marcel Tanguay for his technical support. This research was sponsored by a Discovery grant from the Natural Sciences and Engineering Research Council of Canada (NSERC).