Perfect Solvent- and Catalyst-Free Syntheses of Imine Derivatives Using the Pressure Reduction Technique ()
1. Introduction
From the viewpoint of green chemistry, it was considered that using organic solvents in organic syntheses aimed at formation convenient compounds was not environmentally friendly, in recent years. Several attempts have been made to avoid using organic solvents in organic synthetic methods. Among them, the method attracting attention was to use water instead of an organic solvent [1] [2] [3] [4]. These investigations were very interesting because water was indispensable to the natural environment and was unlikely to cause environmental destruction. Since water was more inexpensive than organic solvents, the cost of the synthetic reaction could be reduced. Furthermore, it was possible to develop a completely different reaction field when organic reactions proceeded in water, and further development was expected in the future. On the other hand, a solvent-free reaction had also been recognized as a suitable reaction for green chemistry [5] [6] [7] [8]. Therefore, various solvent-free reaction systems had been developed [9] [10] [11]. In the last year, we reported that efficient solvent- and catalyst-free syntheses of imine derivatives apply the pressure reduction technique [12]. And more, other researchers also reported that reducing pressure technology was effective for syntheses of some imines [13]. In our previous reaction system, it was found that target imines could be obtained in good yields by removing water under reduced pressure conditions. We also found that this reaction proceeded rapidly in the initial stage, but its rate decreased with the passage of time. Although this reaction system gave target products in good yields, the purification of the target product was required, such as the column chromatography using hexane/ether combination or the recrystallization using dichloromethane/hexane combination. Thus, our previous system was not a complete solvent-free synthesis system. Therefore, we tried two methods in order to improve this point. One was exactly mixing (1:1, substance ratio) aldehydes and amines and the other was employing lower pressure (>0.1 mmHg, previous method: 1.0 mmHg) at the pressure reducing technique to remove water and unreacted materials perfectly. Actually, it was found that pure target imines were obtained in excellent yields without any purification using organic solvents, when we tried improved reaction methods (Scheme).
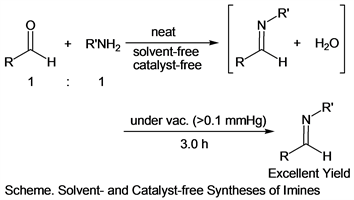
In this paper, we will report the details of the improved reaction method.
2. Experimental
2.1. Chemicals and Instruments
Standard bench top techniques were employed for handling air-sensitive reagent. Liquid aromatic aldehydes and all amines were distilled under argon before use. p-Bromobenzaldehyde and p-chlorobenzaldehyde were purified by recrystallization before use. All reactions were carried out under nitrogen atmospheres. All yields of target compounds were isolated yields. ULVAC G-50DA (ULVAC KIKO Inc.) was used for carrying out this reducing pressure operation. IR spectra were recorded on an FT/IR-610 (JASCO) spectrophotometer. 1H-NMR, 13C-NMR and 19F-NMR spectra were measured on Bruker BioSpin AVANCE III 400 Nanobay spectrometer at 400.1, 100.6 and 376.5 MHz, respectively. Chemical shifts were given in ppm relative to TMS (1H and 13C) or CFCl3 (19F).
2.2. Typical Experimental Procedure 1 (Reaction of p-Tolualdehyde with o-Toluidine)
To a stirring p-tolualdehyde (1.20 g, 10.0 mmol) was added dropwise o-toluidine (1.07 g, 10.0 mmol) at 25˚C After 1.5 hours, the reaction system was connected to a vacuum pump, the pressure was reduced to >0.1 mmHg (13.3 Pa) and stirred for 3.0 hours to give the desired pure compound (Compound 1) as a pale yellow clear oil in 99% yield (2.06 g) without any purification. All physical properties of this product were completely consistent with literature values [14] [15] or physical data of the commercially available compound.: IR (neat): 495, 720, 756, 815, 882, 1111, 1172, 1485, 1572, 1594, 1608, 1627, 2856, 2921, 3021, 3060 cm–1; 1H-NMR (CDCl3) δ (ppm): 2.33 (s, 3H, H1), 2.40 (s, 3H, H2), 6.89 (d, J = 7.80 Hz, 1H, H3), 7.03 - 7.11 (m, 1H, H4), 7.16 - 7.22 (m, 2H, H5 and H6), 7.26 (d, J = 7.92 Hz, 2H, H7), 7.79 (d, J = 7.92 Hz, 2H, H8), 8.30 (s, 1H, H9); 13C-NMR (CDCl3) δ (ppm): 17.69 (C1), 21.46 (C2), 117.56 (C3), 125.27 (C4), 126.52 (C5), 128.55 (C6), 129.30 (C7), 130.02 (C8), 131.61 (C9), 133.74 (C10), 141.50 (C11), 151.14 (C12), 159.23 (C13).

p-Tolualdehyde (1.20 g, 10.0 mmol) reacted with m-toluidine (1.07 g, 10 mmol) to give the corresponding pure compound (Compound 2) in 99% yield (2.06 g) as a colorless crystal in the same manner of procedure 1. All physical properties of this product were completely consistent with literature values [16] or physical data of the commercially available compound.: IR (neat): 479, 694, 774, 792, 816, 930, 1148, 1173, 1307, 1483, 1573, 1599, 1627, 2731, 2863, 2919, 3024 cm–1; 1H-NMR (CDCl3) δ (ppm): 2.39 (s, 3H, H1), 2.42 (s, 3H, H2), 7.00 - 7.05 (m, 3H, H3, H4 and H5), 7.24 - 7.30 (m, 1H, H6), 7.28 (d, J = 8.12 Hz, 2H, H7), 7.79 (d, J = 8.12 Hz, 2H, H8), 8.41 (s, 1H, H9); 13C-NMR (CDCl3) δ (ppm): 21.44 (C1), 21.66 (C2), 117.86 (C3), 121.66 (C4), 126.54 (C5), 128.79 (C6), 128.96 (C7), 129.52 (C8), 133.71 (C9), 138.96 (C10), 141.81 (C11), 152.25 (C12), 160.19 (C13).
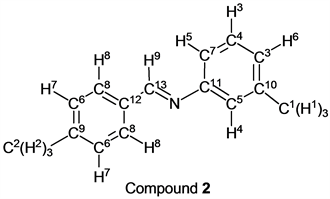
p-Tolualdehyde (1.20 g, 10.0 mmol) reacted with p-toluidine (1.07 g, 10 mmol) to give the corresponding pure compound (Compound 3) in 98% yield (2.05 g) as a colorless crystal in the same manner of procedure 1. All physical properties of this product were completely consistent with literature values [17] [18] [19] or physical data of the commercially available compound.: IR (neat): 467, 539, 718, 823, 885, 977, 1108, 1170, 1504, 1568, 1605, 1624, 2859, 2915, 3026 cm–1; 1H-NMR (CDCl3) δ (ppm): 2.36 (s, 3H, H1), 2.41 (s, 3H, H2), 7.13 (d, J = 8.20 Hz, 2H, H3), 7.19 (d, J = 8.20 Hz, 2H, H4), 7.27 (d, J = 7.96 Hz, 2H, H5), 7.78 (d, J = 7.96 Hz, 2H, H6), 8.42 (s, 1H, H7); 13C-NMR (CDCl3) δ (ppm): 21.04 (C1), 21.66 (C2), 120.83 (C3), 128.73 (C4), 129.52 (C5), 129.76 (C6), 133.79 (C7), 135.60 (C8), 141.69 (C9), 149.64 (C10), 159.65 (C11).
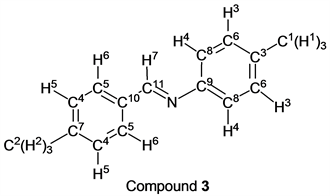
p-Anisaldehyde (1.36 g, 10.0 mmol) reacted with o-toluidine (1.07 g, 10 mmol) to give the corresponding pure compound (Compound 4) in 99% yield (2.24 g) as a colorless crystal in the same manner of procedure 1. All physical properties of this product were completely consistent with literature values [20] [21] [22] or physical data of the commercially available compound.: IR (neat): 450, 517, 724, 757, 832, 883, 1031, 1107, 1164, 1253, 1309, 1511, 1574, 1605, 2332, 2559, 2838, 2910, 2934, 2955, 3009, 3065 cm–1; 1H-NMR (CDCl3) δ (ppm): 2.35 (s, 3H, H1), 3.86 (s, 3H, H2), 6.89 - 6.91 (m, 1H, H3), 6.98 (d, J = 8.72 Hz, 2H, H4), 7.08 - 7.12 (m, 1H, H5), 7.18 - 7.29 (m, 2H, H6 and H7), 7.85 (d, J = 8.72 Hz, 2H, H8), 8.28 (s, 1H, H9); 13C-NMR (CDCl3) δ (ppm): 17.73 (C1), 55.26 (C2), 113.96 (C3), 117.61 (C4), 125.11 (C5), 126.53 (C6), 129.37 (C7), 130.02 (C8), 130.22 (C9), 131.60 (C10), 151.24 (C11), 158.56 (C12), 161.94 (C13).
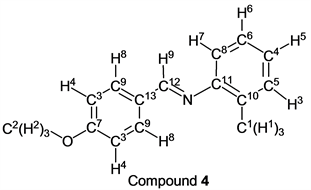
p-Anisaldehyde (1.36 g, 10.0 mmol) reacted with m-toluidine (1.07 g, 10 mmol) to give the corresponding pure compound (Compound 5) in 99% yield (2.24 g) as a colorless crystal in the same manner of procedure 1. All physical properties of this product were completely consistent with literature values [21] [23] or physical data of the commercially available compound.: IR (neat): 443, 530, 694, 792, 834, 933, 1024, 1105, 1165, 1246, 1309, 1419, 1457, 1508, 1604, 1621, 2554, 2839, 2910, 2935, 2966 cm–1; 1H-NMR (CDCl3) δ (ppm): 2.38 (s, 3H, H1), 3.87 (s, 3H, H2), 6.95 - 7.05 (m, 3H, H3, H4 and H5), 6.98 (d, J = 8.72 Hz, 2H, H6), 7.25 - 7.29 (m, 1H, H7), 7.84 (d, J = 8.72 Hz, 2H, H8), 8.39 (s, 1H, H9); 13C-NMR (CDCl3) δ (ppm): 21.45 (C1), 55.45 (C2), 114.18 (C3), 117.87 (C4), 121.66 (C5), 126.36 (C6), 128.96 (C7), 129.32 (C8), 130.49 (C9), 138.94 (C10), 152.37 (C11), 159.54 (C12), 162.19 (C13).
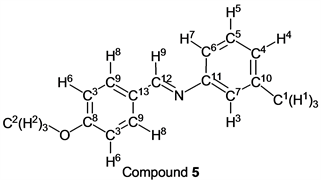
p-Anisaldehyde (1.36 g, 10.0 mmol) reacted with p-toluidine (1.07 g, 10 mmol) to give the corresponding pure compound (Compound 6) in 98% yield (2.21 g) as a colorless crystal in the same manner of procedure 1. All physical properties of this product were completely consistent with literature values [18] [24] [25] [26] [27] or physical data of the commercially available compound.: IR (neat): 505, 546, 590, 820, 838, 1025, 1105, 1166, 1249, 1420, 1508, 1570, 1604, 2555, 2841, 2876, 2971, 3005 cm–1; 1H-NMR (CDCl3) δ (ppm): 2.37 (s, 3H, H1), 3.87 (s, 3H, H2), 6.98 (d, J = 8.80 Hz, 2H, H3), 7.12 (d, J = 8.04 Hz, 2H, H4), 7.17 (d, J = 8.04 Hz, 2H, H5), 7.84 (d, J = 8.80 Hz, 2H, H6), 8.39 (s, 1H, H7); 13C-NMR (CDCl3) δ (ppm): 20.99 (C1), 55.42 (C2), 114.13 (C3), 120.76 (C4), 129.35 (C5), 129.71 (C6), 130.38 (C7), 135.35 (C8), 149.72 (C9), 159.00 (C10), 162.08 (C11).
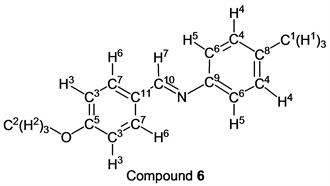
2.3. Typical Experimental Procedure 2 (Reaction of p-Tolualdehyde with Benzylamine)
To a stirring p-tolualdehyde (1.20 g, 10.0 mmol) was added dropwise benzylamine (1.07 g, 10.0 mmol) at 25˚C. After 2.0 hours, the reaction system was connected to a vacuum pump, the pressure was reduced to >0.1 mmHg (13.3 Pa) and stirred for 3.0 hours to give the desired pure compound (Compound 7) as a white solid in 97% yield (2.03 g) without any purification. All physical properties of this product were completely consistent with literature values [28] [29] or physical data of the commercially available compound.: IR (neat): 442, 505, 697, 733, 819, 865, 1028, 1173, 1307, 1377, 1453, 1496, 1606, 1648, 2837, 2920, 3027, 3061 cm–1; 1H-NMR (CDCl3) δ (ppm): 2.38 (s, 3H, H1), 4.80 (s, 2H, H2), 7.22 (d, J = 7.96 Hz, 2H, H3), 7.24 - 7.28 (m, 1H, H4), 7.32 - 7.36 (m, 4H, H5 and H6), 7.67 (d, J = 7.96 Hz, 2H, H7), 8.35 (s, 1H, H8); 13C-NMR (CDCl3) δ (ppm): 21.51 (C1), 65.02 (C2), 126.91 (C3), 127.94 (C4), 128.23 (C5), 128.45 (C6), 129.31 (C7), 133.51 (C8), 139.39 (C9), 141.04 (C10), 161.94 (C11).
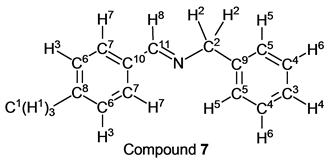
p-Anisaldehyde (1.36 g, 10.0 mmol) reacted with benzylamine (1.07 g, 10 mmol) to give the corresponding pure compound (Compound 8) in 100% (2.25 g) as a colorless crystal in the same manner of procedure 2. All physical properties of this product were completely consistent with literature values [30] [31] or physical data of the commercially available compound.: IR (neat): 462, 528, 697, 731, 1033, 1165, 1252, 1308, 1449, 1510, 1604, 1650, 2551, 2813, 2844, 2940, 2969, 2999, 3028 cm–1; 1H-NMR (CDCl3) δ (ppm): 3.84 (s, 3H, H1), 4.79 (s, 2H, H2), 6.93 (d, J = 8.80 Hz, 2H, H3), 7.23 - 7.29 (m, 1H, H4), 7.32 - 7.36 (m, 4H, H5 and H6), 7.73 (d, J = 8.80 Hz, 2H, H7), 8.32 (s, 1H, H8); 13C-NMR (CDCl3) δ (ppm): 55.35 (C1), 64.95 (C2), 113.96 (C3), 126.90 (C4), 127.94 (C5), 128.45 (C6), 129.06 (C7), 129.83 (C8), 139.52 (C9), 161.35 (C10), 161.67 (C11).
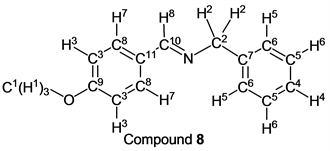
p-Fluorobenzaldehyde (1.24 g, 10.0 mmol) reacted with benzylamine (1.07 g, 10 mmol) to give the corresponding pure compound (Compound 9) in 99% (2.11 g) as a pale yellow clear oil in the same manner of procedure 2. All physical properties of this product were completely consistent with literature values [32] or physical data of the commercially available compound.: IR (neat): 470, 518, 698, 734, 837, 1151, 1230, 1293, 1454, 1508, 1602, 1644, 2841, 3030, 3063 cm–1; 1H-NMR (CDCl3) δ (ppm): 4.81 (s, 2H, H1), 7.09 (t, JHH = 8.76 Hz, JHF = 8.76 Hz, 2H, H2), 7.24 - 7.28 (m, 1H, H3), 7.32 - 7.37 (m, 4H, H4 and H5), 7.77 (dd, JHH = 8.76 Hz, JHF = 5.52 Hz, 2H, H6), 8.35 (s, 1H, H7); 13C-NMR (CDCl3) δ (ppm): 64.94 (C1), 115.68 (d, JCF = 21.78 Hz, C2), 127.04 (C3), 127.96 (C4), 128.51 (C5), 130.14 (d, JCF = 8.49 Hz, C6), 132.41 (d, JCF = 2.96 Hz, C7), 139.14 (C8), 160.47 (C9), 164.32 (d, JCF = 250.66 Hz, C10); 19F-NMR (CDCl3) δ (ppm): −109.86 (F1).
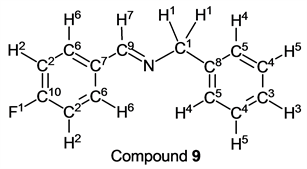
p-Chlorobenzaldehyde (1.41 g, 10.0 mmol) reacted with benzylamine (1.07 g, 10 mmol) to give the corresponding pure compound (Compound 10) in 99% (2.27 g) as a colorless crystal in the same manner of procedure 2. All physical properties of this product were completely consistent with literature values [33] [34] or physical data of the commercially available compound.: IR (neat): 462, 506, 701, 735, 821, 827, 859, 1012, 1044, 1088, 1340, 1371, 1450, 1491, 1593, 1645, 2802, 2818, 2852, 2874, 3030, 3062, 3084 cm–1; 1H-NMR (CDCl3) δ (ppm): 4.81 (s, 2H, H1), 7.24 - 7.29 (m, 1H, H2), 7.32 - 7.36 (m, 4H, H3 and H4), 7.38 (d, J = 8.52 Hz, 2H, H5), 7.71 (d, J = 8.52 Hz, 2H, H6), 8.34 (s, 1H, H7); 13C-NMR (CDCl3) δ (ppm): 64.99 (C1), 127.08 (C2), 127.97 (C3), 128.53 (C4), 128.86 (C5), 129.43 (C6), 134.57 (C7), 136.68 (C8), 138.99 (C9), 160.54 (C10).
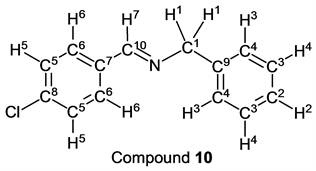
p-Bromobenzaldehyde (1.85 g, 10.0 mmol) reacted with benzylamine (1.07 g, 10 mmol) to give the corresponding pure compound (Compound 11) in 100% (2.74 g) as a colorless crystal in the same manner of procedure 2. All physical properties of this product were completely consistent with literature values [35] [36] or physical data of the commercially available compound.: IR (neat): 460, 500, 513, 698, 732, 857, 1009, 1044, 1068, 1295, 1340, 1370, 1450, 1484, 1588, 1644, 2802, 2851, 2874, 3028, 3060, 3082 cm–1; 1H-NMR (CDCl3) δ (ppm): 4.80 (s, 2H, H1), 7.24 - 7.29 (m, 1H, H2), 7.30 - 7.37 (m, 4H, H3 and H4), 7.54 (d, J = 8.48 Hz, 2H, H5), 7.64 (d, J = 8.48 Hz, 2H, H6), 8.33 (s, 1H, H7); 13C-NMR (CDCl3) δ (ppm): 65.00 (C1), 125.14 (C2), 127.08 (C3), 127.97 (C4), 128.52 (C5), 129.65 (C6), 131.81 (C7), 134.98 (C8), 138.95 (C9), 160.63 (C10).
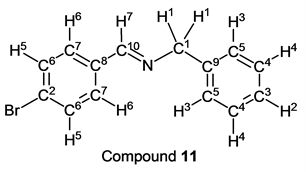
3. Results and Discussion
We found that target imines were obtained in excellent yields easily when aldehydes and amines were mixed without a solvent and a catalyst. Some results of reactions of p-tolualdehyde or p-anisaldehyde with various type toluidines were summarized in Table 1.
p-Tolualdehyde reacted with o-toluidine for 1.5 h without any solvent followed by the vacuum operation (3.0 h) to give the corresponding imine (Compound 1) in 99% yield (entry 1). In this reaction method, any purification, for example, a column chromatography with organic solvents and so on, of the target compound was not needed. The corresponding pure imine (by 1H-NMR analysis, purity: over 99.5%) was obtained. This methodology could be applied to the case of m-toluidine and p-toluidine. When p-tolualdehyde and m-toluidine were stirred under nitrogen for 1.5 h, the target compound (Compound 2) was obtained, quantitatively (entry 2) after the vacuum operation. We also found that p-tolualdehyde reacted with p-toluidine, in the same manner, to give the target imine (Compound 3) in excellent yield (entry 3). Interestingly, an aldehyde that was inert to a nucleophilic reaction could be also used as a starting material for this synthetic method. p-Anisaldehyde was inactive for the nucleophilic reaction because it had a strong electron-donating group (methoxy group) at p-position. When this type of reaction using p-anisaldehyde and o-toluidine as starting materials was carried out, the target compound (Compound 4) was obtained in 99% yield (entry 4). m-Toluidine and p-toluidine also reacted with p-anisaldehyde smoothly, and products (Compounds 5 and 6) were given in 99% yield and 98% yield (entries 5 and 6). It was reported that Compound 1 was obtained in the highest yield (96%) when formic acid, water, and ethanol were used as a catalyst and solvents in the previous study [15]. On the other hand, Compound 2 was given in 51% yield (the highest yield) by iron compounds-promoted C-C bond formation reactions [16]. These facts indicated that our method was more efficient for syntheses of Compounds 1 and 2 because our reactions gave these compounds in higher yields. In the case of the synthesis of Compound 3, it was obtained in 99% yield by the synthetic reaction using pyridine [17]. In contrast, our reaction gave Compound 3 in a similar yield without any solvent, perfectly. Compounds 4, 5 and 6 have already been synthesized by reactions using some solvents and some catalysts in 90% yield, 90% yield and 98% yield, respectively [20] [23] [26]. Our method was more suitable for syntheses of these imines than previous techniques in the viewpoints of yields of target compounds, solvent- and catalyst-free.
Some aldehydes were examined as starting materials for this imine formation and results were shown in Table 2. In this investigation, benzylamine was used as a nucleophile. Although this amine had a milder nucleophilicity than aromatic amines, it had been classified as a strong nucleophile generally.
When aromatic aldehydes having weak or strong electron-donating groups, such as methyl group and methoxy group, were employed as substrates, pure target imines (Compounds 7 and 8) were obtained in 97% yield and 100% yield (entries 1 and 2). This phenomenon indicated that the ability of electron-donation of a substituent at p-position of the aromatic aldehyde did not affect the yield of an imine at all. Halogenated aromatic aldehydes were also used as starting compounds in this synthetic method. The fluorinated imine derivative (Compound 9) was given in excellent yield by the reaction of p-fluorobenzaldehyde and benzylamine (entry 3). Furthermore, p-chlorobenzaldehyde and p-bromobenzaldehyde could be used as substrates in this reaction system to give target compounds (Compounds 10 and 11) in 99% yield and 100% yield (entries 4 and 5). In previous synthetic methods of Compounds 7, 8, 9, 10 and 11, these compounds were obtained in over 90% yields [28] [30] [32] [33] [36]. Meanwhile, our methods gave these compounds in excellent yields that were comparable to previous methods. Additionally, pure target products could be given without any solvent and any catalyst in our method.
Table 1. Solvent-and catalyst-free syntheses of imines.

Table 2. Effective syntheses of various benzylated imines.
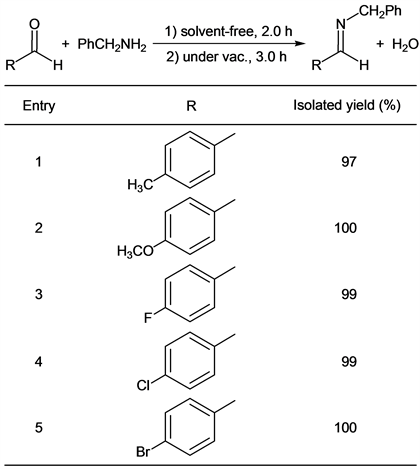
It was revealed that our methodology was superior to the previous one for the synthesis of an imine derivative, because of two reasons that were led from facts in Table 1 and Table 2. The first reason was an excellent yield of a target compound and the second reason was an easy technique under solvent- and catalyst-free conditions.
4. Conclusion
In this paper, we reported that perfect solvent- and catalyst-free syntheses of imine derivatives use the pressure reduction technique. This synthetic reaction proceeded under mild conditions, and desired imines were obtained in excellent yields. In this synthetic method, there were two important key-techniques. When aldehydes and amines were mixed exactly 1:1 (substance ratio) and reduced the pressure of the reaction pod under >0.1 mmHg at the end of this reaction, target imines were obtained, quantitatively. The scope and limitation of this synthetic method are now unknown. Further investigation is in progress.