Consolidation Properties of Compacted Lateritic Soil Treated with Rice Husk Ash ()
One dimensional laboratory consolidation test was conducted on compacted lateritic soils treated with up to 16% rice husk ash (RHA), to assess its consolidation properties. Specimens were prepared at three different moulding water contents (2% dry of optimum, optimum moisture content and 2% wet of optimum) and compacted using the British Standard Light compactive effort. Preliminary tests on soils showed improved index properties with an increase in liquid limit (LL), an increase in plastic limits (PL) with a resulting decrease in plasticity index (PI). Preconsolidation pressure increased with RHA content, it also decreased before increasing with increased moulding water content. Reductions in compression index (Cc) and Swell Index (Cs) with increased RHA content were recorded. Cc and Cs generally decreased before increasing with increased moulding water content. The coefficient of volume compressibility (Mv) decreased and increased with higher RHA content; they were also affected by the soil particle state with increasing pressure. The coefficient of consolidation (Cv) showed no observable trend with increased RHA content but generally increased with higher consolidation pressure on the dry and wet side of optimum compacted states.
1. Introduction
The consolidation of compressible soils is of concern to engineers engaged in the design / construction of foundations, embankments, bridge abutments, earth dams, fills etc. Consolidation deals with the response of soil systems to imposed load and predicts stresses and displacement of the loaded soil as a function of space and time. The theory of consolidation since its introduction by Terzaghi in 1923 has formed the foundation of modern geotechnical engineering where the interaction of soil and water dominates. Although consolidation is used for estimating settlements, it has also played key roles in the design/construction of civil engineering infrastructures [1].
Lateritic soils are the most common reddish colour weathered pedogenic surface deposits occurring in the tropical and subtropical regions of the world. They constitute the most common materials for the construction of earth dams, highways, embankments, airfields as well as foundation materials to support structures in these areas [2].
Their chemical composition and morphological characteristics are influenced by the level of weathering of which the parent material has been subjected to [2]. It is therefore almost impossible to execute any construction work in Nigeria without the use of lateritic soils [3]. The consolidation characteristics of most residual laterite soils appear to depend upon the nature of the soil, the position of the sample in the profile and the characteristics of the material deposit [2].
Evaluation of the consolidation characteristics of residual laterite and other tropically weathered clay on the basis of Terzaghi’s theory of consolidation has been found to be useful in predicting the settlement of structures [4,5]. It was reported [6] that the decrease of the void ratio with applied normal pressure in residual clays follows the same law which governs the consolidation phenomenon for sedimentary clays.
Rice milling generates the product known as rice husk which surrounds the paddy grain. During the milling of paddy, about 78% is received as rice, broken rice and bran. The remaining 22% is received as husk. This husk is used in the rice mills to generate steam used in the parboiling process. The husk contains about 75% organic volatile matter, leaving 25% to be converted into ash during the firing process. Rice husk ash (RHA) contains around 85% - 90% amorphous silica. So for every 1000 kg of paddy milled, about 220 kg (22%) of husk is produced and when the husk is burnt in the boilers, about 55 kg of RHA is generated. It is estimated that about 108 tones of RHA is produced annually worldwide [7]. This is a waste causing damage to the land and the surrounding area in which it is dumped. In the urban and rural areas of Nigeria, local milling is done by women. They mainly use firewood as heat source and as such 100% of the rice husk from the mill is a waste.
Rice husk ash (RHA) is a carbon neutral green product obtained from the husk of rice; an agro-industrial waste. RHA is obtained from the combustion of the husk at a temperature range between 600°C and 800°C for about 12 - 24 hours in excess air. The most striking thing about RHA is the high silica content, as well as the free lime content. The pozzolanic activity of the ash is best obtained in the amorphous phase. To realize this, the above range of temperature and firing length are established especially for commercial production quantity [8].
Rice husk ash (RHA) is a pozzolanic materials which would react in the presence of moisture to yield cementatious products [9]. In developing countries like Nigeria, proper utilization of this agro-industrial wastes has not been given due attention. The rice husk thereby constitutes an environmental nuisance as they form refuse heaps in the areas where they are disposed; hence the need for this investigation. There is a growing demand for pozzolanic materials in the construction industry today; as it is economical for an industrial or agro-industrial waste material like rice husk ash to be used as an additive to improve the engineering properties of lateritic soils.
There exist little data in literature concerning the consolidation properties or behaviour of compacted lateritic soil treated with rice husk ash when subjected to time dependent one dimensional compression. This work aims at understanding the consolidation characteristics of compacted lateritic soil treated with rice husk ash. A better understanding of these properties will enhance the usage of the material in geotechnical engineering works in places where they are abundant.
2. Materials and Methods of Testing
2.1. Materials
2.1.1. Soil
The soil used is a naturally reddish-brown lateritic soil obtained from a borrow pit in Shika area of Zaria (Latitude 11˚15'N and Longitude 7˚45'E), Nigeria. A study of the geological and soil maps of Nigeria [10,11] show that the samples taken belong to the group of ferruginous tropical soil derived from acid igneous and metamorphic rocks. Previous studies on soils from this area have been shown to contain kaolinite as the dominant clay mineral [3]. The soil is classified as A-7-6 (10) according to AASHTO soil classification system [12] and low plasticity clay (CL) according to the Unified Soil Classification System [13].
2.1.2. Rice Husk Ash
The rice husk ash (RHA) used in this study was obtained from Kura local government of Kano State along ZariaKano express way (latitude 11˚46'N and longitude 8˚25'E). The sample was obtained from a local rice milling factory in the neighbourhood. It was burnt under normal atmospheric temperature and pressure (open burning) to obtain the ash. The rice husk ash was sieved through BS sieve No. 200 and the fractions passing through the sieve were used throughout the tests. The sieved ash was immediately stored in air tight containers to avoid pre-hydration during storage or when left in open air. The ash was used to treat the lateritic soil by mixing it in different percentages. The ash treatments considered were 0%, 4%, 8%, 12% and 16% by dry weight of soil used, to give five different soil-rice husk ash mixtures with 0% as the natural soil. Oxide composition of the Bagasse ash used in this study was obtained through a Compact Energy Dispersive X-ray Spectrometer Method (Mini Pal), designed for elementary analysis of a wide range of samples. The test was carried out at Centre for Energy Research Technology (CERT), Ahmadu Bello University Zaria, Nigeria. The Oxide Composition is as given in Table 1.

Table 1. Chemical Composition of Rice Husk Ash Used
2.2. Methods
2.2.1. Index properties
Laboratory tests were conducted to determine the index properties of the natural soil and soil-rice husk ash mixtures in accordance with British Standards [14].
2.2.2. Compaction
British standard Light compactive effort was used. Air dried soil samples passing through British Standard sieve with 4.76 mm aperture mixed with 0%, 4%, 8%, 12% and 16% rice husk ash by weight of dry soil were used. British Standard Light effort was carried out in accordance with British Standard [14].
2.2.3. Consolidation Test
One dimensional consolidation test was carried out in accordance with British Standard [14] and as described by Head [15]. Lateritic soil-rice husk ash mixtures compacted at 2% dry of optimum, at optimum moisture content, and 2% wet of optimum simulating the variation in moisture that might occur in the field. These specimens were cored into the oedometer ring and placed in the consolidometer setup. Pressure increment was 100 kN/ m2, 200 kN/m2, 400 kN/m2 and 800 kN/m2 during the loading stage and unloaded up to 200 kN/m2. Compression readings were recorded between 10 sec and 24 hours during the loading stage for each incremental load. The Taylor method (Square Root of Time Method) was used to analyze experimental results. The pre-consolidation pressure was obtained from the void ratio-pressure curve using the procedure proposed by Casagrande [16].
3. Discussion of Test Results
3.1. Index Properties
The Atterberg limit results show improved index properties with an increase in liquid limit (LL), an increase in plastic limits (PL) with a resulting decrease in plasticity index (PI). The liquid limit increased from 35% to 42% with increase in RHA content from 0% to 16%. This trend was attributed to the excess RHA quantity requiring more water to mix the soil-RHA mix to fluid [17]. Similar results were obtained by other researchers [18-21] when fly ash and RHA was used.
The plastic limit increased from 21% to 31% with increase in RHA content from 0% to 16%. Similar results were obtained by Muntohar [22]. Previous studies [23] using lime showed that the plastic limit of lateritic soils increased with higher lime contents. The plasticity index decreased from 15.7% to 10.7% with increase in RHA content from 0 to 16%. This trend may be attributed to the replacement of the soil particles by RHA fines with consequent reduction in clay content and plasticity index. The plasticity of a soil decreases as the amount of clay fraction decreases and vice versa [24]. The decrease in plasticity index shows that the engineering properties of the soils were improved. This is in agreement with the works of other researchers [17-21,25].
3.2. Compaction Characteristics
The effect of rice husk ash content on the maximum dry density (MDD) and optimum moisture content (OMC) of the lateritic soil-rice husk ash mixtures is shown in Figure 1 With increased rice husk ash treatment for up to 16%, the MDD reduced from 1.76 to 1.43 Mg/m3. This is not unconnected to the initial simultaneous flocculation and agglomeration of clay particles caused by cation exchange leading to increase in volume and decrease in dry density. Also this is probably due to the comparatively low specific gravity value 2.25 of the rice husk ash compared to that of soil which is 2.65.
The OMC increased with rice husk ash treatment for up to 16% with values ranging from 18.0% to 24.2%. This trend is due to the increased fines mainly from rice husk ash which decreased the quantity of free silt and clay fraction and forming coarser materials with larger surface areas (this process needs water to take place). This implies also that more water was needed for the hydration of the rice husk ash. Similar results were reported [17].
3.3. Consolidation Characteristics
The consolidation characteristics of the various soil-rice husk ash mixtures compacted at different moulding water contents were observed through the pre-consolidation pressure, compression index, coefficient of volume compressibility, and coefficient of consolidation; these are discussed under the effect of the rice husk ash content and effect of the moulding water content, and effect of pressure where applicable.
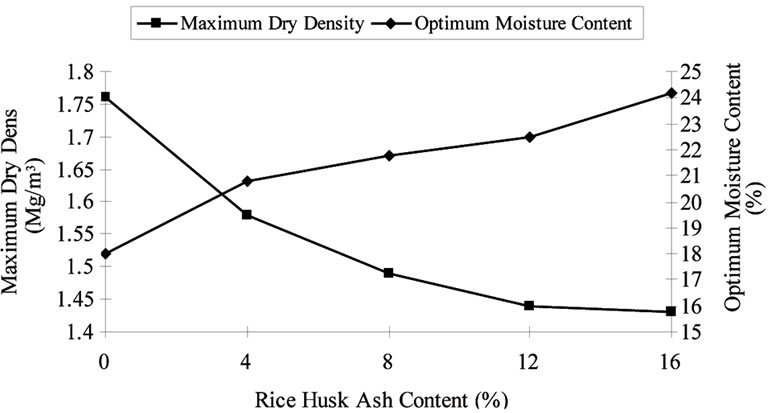
Figure 1. Variation of maximum dry density and optimum moisture content with rice husk ash content.
3.3.1. Pre-Consolidation Pressure
3.3.1.1. Effect of Rice Husk Ash Content
The variation of pre-consolidation pressure with RHA content is shown in Figure 2. The trend shows that the pre-consolidation pressure increased from 420 to 630 kN/m2, 165 to 420 kN/m2 and 162 to 242 kN/m2 with increasing RHA content at the dry side, optimum and wet sides of optimum moisture content, respectively. The increasing RHA content changes the soil matrix with increasing fines, making it easier for the soil to be compacted. This increases the penetration by the compaction rammer on the soil surface resulting in closer alignment of particles along the failure surface. This could also probably be as a result of the increased pozzolanic reaction with increased RHA treatment which results in the formation of calcium silicate hydrates and increased strength. Osinubi and Eberemu [26] reported increased unconfined compressive strength for up to 8% treatment with another pozzolan bagasse ash. The engineering implication of this result is that the soil is able to withstand increased pressure without settling at higher rice husk ash contents.
3.3.1.2. Effect of water content relative to optimum
The variation of pre-consolidation pressure with moulding water content relative to optimum is shown in Figure 3. The trend shows that pre-consolidation pressure generally decreases with higher moulding water content between

Figure 2. Variation of pre-consolidation pressure with rice husk ash content
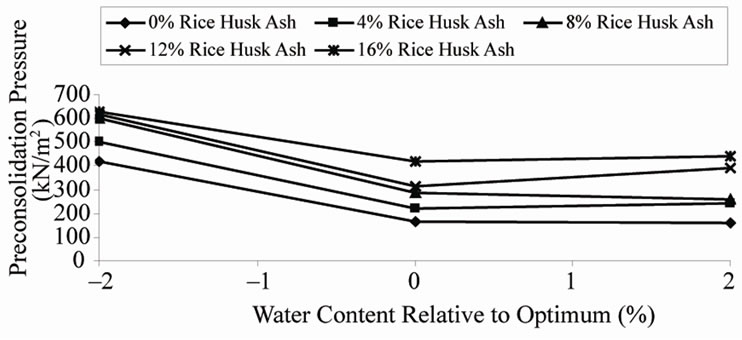
Figure 3. Variation of pre-consolidation pressure with water content relative to optimum.
(–2%) dry and 2% wet of optimum, except between OMC and 2% wet of OMC for 4 and 12% RHA content where a slight increase occurred. The highest value of 690 kN/m2 obtained at 16% RHA content on the dry side of optimum to 162 kN/m2 obtained at 0% RHA on the wet side of optimum moisture content. This may be attributed to particle rearrangement as the moisture content increases. Compaction under higher moulding water contents results in soils devoid of macro pores. The increasing water will help in deflocculating the particle structure reducing voids [27]; hence the arrangement of individual particles influenced by moulding water content will affect the pre-consolidation pressure. The slight increase as the moisture content increases to (+2%) wet may be attributed to the excess moisture causing the particles to slide; hence requiring greater effort to compact. The significance of this is that the soil is able to withstand greater overburden pressure without settling on the dry side.
3.3.2. Compression Index
3.3.2.1. Effect of Rice Husk Ash Content
The variation of compression index with RHA content is shown in Figure 4. The trend shows that the compression index (Cc) generally decreased with increasing RHA for up to 16% treatment. Cc ranged from 0.189 - 0.365, 0.085 - 0.201 and 0.136 - 0.223 at the dry side (–2%), optimum moisture and wet side (+2%) of optimum moisture content respectively with increasing RHA content. This decrease in compression index could be as a result of increased formation of pozzolanic products within the pore spaces from physico-chemical changes [26] which leads to a reduction in compression index. Muntohar [22] observed that the addition of lime and rice husk ash reduces the compressibility of remoulded clays. When the rice husk ash content exceeds the quantity required for the soil-ash reaction, they will be filled between the voids of the soil. A more compact state of the soil is probably attained. Anagnostopoulos [28] also observed
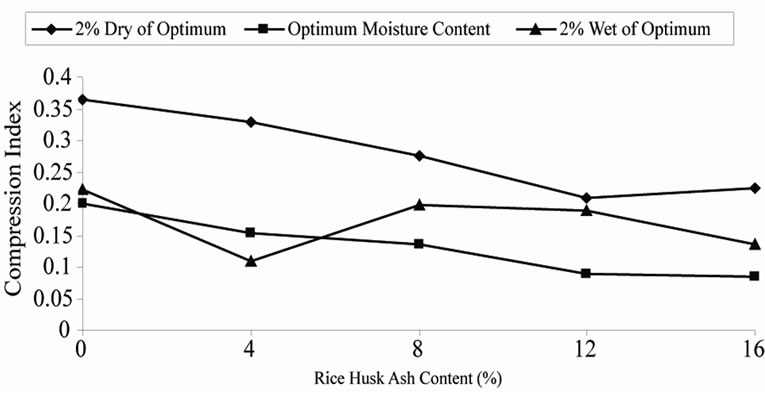
Figure 4. Variation of compression index with rice husk ash content.
a decrease in the compression index of soft soils with increasing cement ratio. Kazemian and Huat [29] observed a decrease in the compression index of fibrous tropical peat reinforced with cement column. The settlement of soils is directly related to the compression index which implies that RHA can be used to reduce the settlement of soils.
3.3.2.2. Effect of water content relative to optimum
The variation of compression index with moulding water content relative to optimum is shown in Figure 5. The trend generally shows that compression index decreases with higher moisture contents from the dry side up to optimum and increases between the optimum and the wet side of optimum. At the optimum moulding water contents, compaction results in soils devoid of macro pores. The increasing water from the dry side to optimum will help in deflocculating the particle structure reducing voids [27]. This means that samples compacted as the water content increased up to optimum contain less voids and hence the reduction in compression index. This implies that a more compact state of soil is attained at optimum moisture content and will compress less when loaded; showing that the compactive index is affected by the particle state of the soil fabric.
3.3.3. Swell Index
3.3.3.1. Effect of Rice Husk Ash Content
The variation of swell index (Cs) with increasing rice husk ash content is shown in Figure 6. The swell index generally decreased with increased RHA content. It ranged from 0.023 - 0.036, 0.016 - 0.043 and 0.037 - 0.049 at the dry side, optimum moisture and wet side of optimum moisture content respectively with higher RHA content. Previous research work [28] showed that the swell index of soft soils was reduced with increasing cement ratio.
3.3.3.2. Effect of Water Content Relative to Optimum
The variation of swell index with water content relative

Figure 5. Variation of compression index with water content relative to optimum.
to optimum is shown in Figure 7. The swell index increases and decreases depending on the RHA treatment as the moulding water content relative to optimum increases from (–2%) dry to optimum and increased slightly on (+2%) wet side. This results show that the swell index is influenced by the particle state of the soil fabric which is controlled by the moulding water content. A more compact state of soil is attained at optimum moisture content and the samples at optimum moisture contents will swell less when unloaded.
3.3.4. Coefficient of Volume Compressibility, (Mv)
The variation of coefficient of volume compressibility with RHA content is shown in Figure 8. At the optimum moisture content the values of Mv ranged from 0.097- 0.485, 0.026 - 0.377, 0.027- 0.255 and 0.019 - 0.042 at 100, 200, 400 and 800 kN/m2 loadings respectively. Previous research work [29] showed that the coefficient of volume compressibility is gradually decreased by increasing the consolidation pressure and cement ratio. It was also observed [22] that the addition of lime and RHA had a reducing effect on the compressibility of remoulded soils.
Generally on the dry side and optimum the Mv decreased up to 4% RHA treatment beyond which it started to increase; on the wet side it decreases up to 8% treatment. This probably could be due to pozzolanic nature

Figure 6. Variation of swell index with rice husk ash content.

Figure 7. Variation of swell index with water content relative to optimum.
(a)
(b)
(c)
Figure 8. Variation of coefficient of volume compressibility with rice husk ash content for (a) 2% dry of optimum; (b) optimum moisture content; (c) 2% wet of optimum.
and pozzolanic reaction taking place. Beyond 8% treatment, the soil matrix is changed and Mv begins to increase.
Effect of Pressure The variation of coefficient of volume compressibility (Mv) with pressure is shown in Figure 9. The trend shows that on the dry side of optimum, Mv decreases between 100 and 200 kN/m² before it increases. This can be attributed to the macro pores contained in the soil at the dry state (2% dry). At the optimum (OMC) and wet of optimum (2% wet), the coefficient of volume compressibility generally decreased with increased pressure. The increasing water contents contained in the soil helps in deflocculating the particle structure and hence reducing voids. This arrangement of individual particles influenced by increasing moulding water content results in smaller interclod voids [27] and hence reduced coefficients of compressibility under loading. Similar results were also obtained by Kazemian and Huat [29].
(a)
(b)
(c)
Figure 9. Variation of coefficient of volume compressibility with rice husk ash content for (a) 2% dry of optimum; (b) optimum moisture content; (c) 2% wet of optimum.
3.3.5. Coefficient of Consolidation (Cv)
The variation of coefficient of consolidation with RHA content is shown in Figure 10. No trend was observed on Cv with increased RHA treatment. This result is not in agreement with the results of other researchers [30,31]. Kazemian and Huat [29] observed that in fibrous reinforced tropical peat treated with cement, there was decrease in Cv with increased cement ratio.
Effect of Pressure The variation of coefficient of consolidation with pressure is shown in Figure 11. The trend shows that coefficient of consolidation generally increases with higher consolidation pressure on the dry and wet side of optimum. As the consolidation pressure increases beyond the preconsolidation pressure the soil will settle faster. This could also be attributed to the consolidation being
(a)
(b)
(c)
Figure 10. Variation of coefficient of consolidation with rice husk ash content for (a) 2% dry of optimum; (b) optimum moisture content; (c) 2% wet of optimum.
controlled by mechanical effects (physical properties of mineral particles). This is in agreement with other works [31].
4. Conclusions
A reddish brown lateritic soil was treated with up to 16% rice husk ash. Treated specimens were, compacted using standard proctor effort at different moulding water contents (2% dry of optimum, optimum moisture content and 2% wet of optimum) and subjected to one dimensional consolidation test; to assess their consolidation characteristics.
Index tests showed improved index properties with an increase in liquid limit (LL), an increase in plastic limits (PL) with a resulting decrease in plasticity index (PI); with increased RHA treatment. Compaction results revealed a decreasing maximum dry density (MDD) and increasing optimum moisture content (OMC) for up to 16% RHA content.
(a)
(b)
(c)
Figure 11. Variation of coefficient of consolidation with pressure for (a) 2% dry of optimum; (b) optimum moisture content; (c) 2% wet of optimum.
The pre-consolidation pressure increased irrespective of the moulding water content with increasing RHA content for up to 16% treatment. The preconsolidation pressure decreases with higher moulding water content relative to optimum between 2% dry of optimum and optimum; it slightly increases at 2% wet of optimum. The compression index decreased steadily with higher RHA contents for up to 16% treatment, irrespective of the moulding water content. The compression index also decreased with higher moulding water content.
The Swell Index generally decreases with higher RHA contents for up to 16% treatment irrespective of the moulding water content. The swell index also decreases with higher moulding water content relative to optimum between 2% dry of optimum and optimum moisture content. It slightly increased between the OMC and 2% wet of optimum.
The Coefficient of Volume Compressibility (MV) decreases with increasing RHA content for up to 4% treatment on specimens prepared at 2% dry and optimum moisture content. It also decreased for up to 8% RHA treatment for specimens prepared at 2% wet of optimum. The variation of coefficient of volume compressibility (Mv) with pressure shows that on the dry side of optimum, Mv decreases between 100 and 200 kN/m² before it increases. At the optimum (OMC) and wet of optimum (2% wet), the coefficient of volume compressibility generally decreased with increased pressure, showing that it is influenced by the particle state of the soil.
The Coefficient of Consolidation (Cv) showed no observable trend with increase in RHA content. This implies that increasing RHA content did not have a unique impact on the time rate of settlement. The coefficient of consolidation (Cv) generally increases with higher consolidation pressure. These findings show that compacted lateritic soil treated with RHA will be suitable for reclaiming lowlying marginal land for foundation works and for fills in embankment works.
5. Acknowledgements
I wish to acknowledge the effort of Ovye John Akyengo of the department of Civil Engineering Ahmadu Bello University Zaria, for his contribution towards the success of this work.
[1] R. L. Schiffman, V. Pane and R. E. Gibson, “The Theory of One-dimensional Consolidation of Saturated Clays. IV. An Overview of Non-linear Finite Strain sedimentation and Consolidation,” Proceedings, ASCE Symposium on Sedimentation/Consolidation Models, San Francisco, 1984, pp. 1-29.
[2] M. D. Gidigasu, “Laterite Soil Engineering Pedo-Genesis and Engineering Principles,” Amsterdam Elsevier Scientific, New York, 1976, p. 554.
[3] K. J. Osinubi, “Permeability of Lime Treated Lateritic Soil,” Journal of Transportation Engineering, Vol. 124, No. 5, 1998, pp. 465-469. doi:10.1061/(ASCE)0733-947X(1998)124:5(465)
[4] D. W. Lamb, “Decomposed Laterite as Fill Material with Particular to Earth Dam Construction,” Proceedings of Symposia Hong Kong Soils, Hong Kong Group, Hong Kong, 1962, pp. 57-71.
[5] W. R. Macchechnie, “Some Consolidation Characteristics of a Residual Mica Schist,” Proceedings of the Regional Conference for Africa―Soil Mechanics and Foundation Engineering, Vol. 44, No. 2, 1967, pp. 135-139.
[6] M. Vargas, “Correlation between Angle of Internal Friction and Angle of Shearing Resistance in Consolidated Quick Triaxial Compression Test on Residual Clays,” Proceedings of the 3rd International Conference on Soil Mechanics and Foundation Engineering, Zurich, Vol. 1, 1953, pp. 72-75.
[7] E. B. Oyetola and M. Abdullahi, “The Use of Rice Husk Ash in Low-Cost Sandcrete Block Production,” Department of Civil Engineering, Federal University of Technology, Minna, 2006.
[8] M. M. Tashima, C. A. R. Silva, J. L. Akasaki and M. B Barbosa, “The Possibility of Adding the Rice Husk Ash (RHA) to the Concrete,” Proceedings of the 2004 International RILEM Conference on the Use of Recycled Materials in Building and Structures, Barcelona,8-11 November 2004, pp. 778-786.
[9] http://www.ricehuskash.com accessed, 3 August 2010.
[10] F. A. Akintola, “Geology and Geomorphology,” Nigeria in Maps, In: K. M. Barbour, J. S. Oguntoyinbo, J. O. C. Onyemelukwe and J. C. Nwafor, Eds., Hodder and Stoughton, London, 1982, P. 209. ISBN: 0841907633.
[11] O. Areola, “Soils,” Nigeria in Maps, In: K. M. Barbour, J. S. Oguntoyinbo, J. O. C. Onyemelukwe and J. C. Nwafor. Eds., Hodder and Stoughton, London, 1982, p. 209, ISBN: 0841-907633.
[12] American Association of State Highway and Transportation Officials, “Standard specification for transportation materials and methods of sampling and testing,” 14th Edition, Washington, D.C, 1986.
[13] American Society for Testing and Materials, “Annual Book of ASTM Standards,” Philadephia, Vol. 4-8, 1992.
[14] British Standard Institute, “Methods of testing soils for civil engineering purposes,” BS 1377, London, 1990.
[15] K. H. Head, “Manual of Soil Laboratory Testing,” Permeability, Shear Strength and Compressibility Tests, Pentech Press, London, 1994, p. 440.
[16] A. Casagrande, “The Determination of the Pre-Consolidation Load and Its Practical Significance,” Proceedings of the 1st International Conference on Soil Mechanics, Harvard, Vol. 3, 1936.
[17] F. O. Okafor and U. N. Okonkwo, “Effect of Rice Husk Ash on Some Geotechnical Properties of Lateric Soil,” Leonardo Electronic Journal of Practices and Technologies, No. 15, 2009, pp. 67-74
[18] G. Ferguson, “Use of Self-Cementing Fly Ashes as a Soil Stabilization Agent,” Fly Ash for Soil Improvement, Geotechnical Special Publication, New York, 1993, pp. 1-14.
[19] P. G. Nicholson and V. Kashyap, “Fly-Ash Stabilization of Tropical Hawaiian Soils,” In: D. Kevan, Fly-Ash for Soil Improvement, Sharp. Geotechnical Special Publication, Opelika, 1993, pp.15-29.
[20] E. Coka, “Use of Class C Fly Ashes for the Stabilization of an Expansive Soil,” Journal of Geotechnical and Geoenvironmental Engineering, Vol. 127, No. 7, 2001, pp. 568-573. doi:10.1061/(ASCE)1090-0241(2001)127:7(568)
[21] A. O. Eberemu, A. A. Amadi and K. J. Osinubi, “The Use of Compacted Tropical Clay Treated with Rice Husk Ash as a Suitable Hydraulic Barrier Material in Waste Containment Applications,” Accepted for presentation at the ‘International Conference on Waste Management in Developing Countries and Transient Economies Mauritius, Africa’, 5-9 September 2011.
[22] A. S. Muntohar, “Utilization of Uncontrolled Burnt Rice Husk Ash in Soil Improvement,” Dimensi Tekiksipil, Vol. 4, No. 2, 2002, pp. 100-105.
[23] S. Ola, “Geotechnical Properties and Behavior of Some Stabilized Nigerian Lateritic Soils,” Quarterly Journal of Engineering Geology and Hydrogeology, Vol. 11. No.2. 1978, pp. 145-160. doi:10.1144/GSL.QJEG.1978.011.02.04
[24] K. R. Arora, “Soil Mechanics and Foundation Engineering,” Standard Publishers Distributors, Delhi, 2008.
[25] A. O. Eberemu, A. A. Amadi and J. Sule, “Desiccation Effect on Compacted Tropical Clay Treated with Rice Husk Ash,” In: Jie Han and Daniel E. Alzamora, Eds., Advances in Geotechnical Engineering, Geotechnical Special Publication, Opelika, 2011, pp. 1192-1201.
[26] K. J. Osinubi and A. O. Eberemu, “Effect of Bagasse Ash on the Strength of Stabilized Lateritic Soil,” Proceedings of 5th Nigerian Material Congress, Abuja, 15-18 November 2006, pp. 214-220.
[27] J. K. Mitchell, “Fundamental of Soil Behaviour,” John Wiley and Sons, Inc., New York, 1976, p. 422.
[28] A. C. Anagnostopoulos and E. I. Stavridakis, “Influence of Sand Content on Cement and Durability of Cement-Acrylic Resin Treated Soil,” Electronic Journal of Geotechnical Engineering, Vol. 8, Bundle D, 2003.
[29] S. Kazemian and B. B. K. Huat, “Compressibility Characteristics of Fibrous Tropical Peat with Reinforced with Cement Column,” Electronic Journal of Geotechnical Engineering. Vol. 14, Bundle C, 2009, pp 1-13.
[30] R. E. Olson and G. Mesri, "Mechanisms Controlling the Compressibility of Clays," Journal of the Soil Mechanics and Foundations Division, Vol. 96, No. 6, 1970, pp. 1863‑1878.
[31] R. G. Robinson and M. M, Allam “Effect of Clay Mineralogy on the Coefficient of Consolidation,” Clay and Clay minerals, Vol.,46, No. 5, 1998, pp. 596-600