The Influence of Water Hyacinth Floating Meadows on Limnological Characteristics in Shallow Subtropical Waters ()
1. Introduction
Aquatic macrophytes influence the quality of water bodies by providing organic matter and by influencing nutrient dynamics as well as the retention and transport of organic and inorganic silts [1] -[7] .
Eighty percents of the lakes and ponds of the lower Paraná floodplain are covered with floating plants, and the dominant aquatic plant species is Eichhornia crassipes (Mart.) Solms (water hyacinth) [4] [8] . Floating meadows, which may cover from <5% to 100% of the available surface of these lakes [1] [9] , interfere significantly with light penetration into the water and restrict photosynthesis due to increased sedimentation and shading of the water column, which leads to deoxygenation and has a detrimental impact on aquatic organisms, particularly fish [10] , zooplankton [11] and periphyton [12] . Other water quality effects include higher sedimentation rates within the plant’s complex root structure and the capture of inorganic phosphorus during floods [13] .
In these lakes, water circulation is reduced to a daily period of mixing, which occurs during the morning [3] . The floating meadows of water hyacinth produce a mean of 5.1 g∙m−2∙day−1 of organic matter [13] using a significant amount of nutrients, mainly nitrogen and phosphorus [1] [3] .
During the decomposition process, specifically through leaching and the mineralization of organic matter, floating meadows release nutrients and organic compounds that influence the chemistry of the surrounding waters [3] . This process depends on the oxygen concentration in the water [12] [14] ; thus, there is a feedback loop between the physical and chemical conditions of lakes and the growth of water hyacinth. The nitrate content of water is the most important external variable that influences the decay rates of E. crassipes in different floodplain lakes of the Paraná River [15] . The effects of aquatic vegetation on the environmental characteristics of floodplain lakes in tropical rivers remain poorly understood, despite the great scientific interest in understanding the dynamics of other communities. Most previous studies have been focused on the environmental factors that determine the distribution of aquatic plants [16] -[18] .
The aim of this study was to assess the influence of E. crassipes floating meadows on four limnological variables (temperature, dissolved oxygen, electrical conductivity and pH) in floodplain lakes of the Paraná River over the course of one year. The effects of vertical stratification and daily variation were studied in two experimental ponds: one with and one without E. crassipes cover.
We tested the following hypotheses: 1) E. crassipes cover determines the depletion of dissolved oxygen; 2) water temperature, electrical conductivity and pH differ between ponds with and without water hyacinth; and 3) limnological water conditions vary daily as a consequence of the cover provided by E. crassipes floating meadows.
2. Materials and Methods
2.1. Study Site
To study the effects of water hyacinth on the limnological characteristics of water, two natural lakes—El Puente and San Nicolás—were selected. These lakes are located on the west bank of the Paraná River within the Ramsar site in Chaco, Argentina. Because lakes situated on the river floodplain are regularly flooded, experiments with enclosures in floodplain lakes could only be performed over a short period of time due to frequent river overflow [3] . Thus, to prevent the mobility of E. crassipes floating meadows in one-year-long experiments, two experimental ponds located 12 km from the river course were used. These ponds, which were constructed of cement, were previously used in an experimental study [10] .
The lakes belong to a complex of several similar lakes and ponds (Figure 1) located parallel to, and approximately 2 km from, the river [19] . Typical floodplain lakes of this area are small (200 × 2000 m), shallow (0.4 - 2 m deep) and separated from the river by alluvial levees (20 m wide, 1 - 2 m high) occupied by gallery forests. As river water enters the floodplain lakes, dissolved inorganic nitrogen, especially NO3, increases to high levels. At a high water level, phosphate concentrations vary between 50 and 105 μg∙l−1 and show slight changes during and after the flood [3] [20] .
Lake San Nicolás (27˚27'S, 58˚55'W) connects to the Paraná River one to three times per year, and during this study, water hyacinth covered 70% - 90% of its surface and grew to a depth of 1.50 - 2.00 m.
Lake El Puente (26˚26'S, 58˚51'W) suffers more frequent long-lasting flooding events than Lake San Nicolás [19] , and water hyacinth covered 40% - 60% of its surface with a depth range similar to that observed in Lake San Nicolás.
Ponds A and B, located at 27˚28'S and 58˚44'W with dimensions of 10 m × 2.10 m and depths of 1.60 m, were filled with water from the Paraná River. The chemical conditions of these ponds were similar to those of the floodplain lakes. Organic matter on the bottom of both ponds was the result of E. crassipes decomposition.
Figure 1. Location of the sampling sites on the Paraná River floodplain.
Water lost due to evaporation and evapotranspiration was replaced with river water. Pond A was stocked to a surface coverage of 90% with water hyacinth plants with elongated leaves (large biotype), which occur in dense mats; in pond B, water hyacinth was absent.
2.2. Sampling
Sampling operations were performed for one year (from April 1998 to April 1999) on the natural lakes and the experimental ponds. Due to the movement of floating meadows and access limitations in the field area, the measurements of the floodplain lakes were performed once a month at 9 a.m. at a depth of 20 cm. The experimental ponds were sampled at 8 a.m. and at 2 p.m. at 3-day intervals, following a vertical profile of 0.10, 0.20, 0.50, 1.0 and 1.5 m depth.
Water temperature and oxygen concentration were measured with a YSI 54A polarographic probe, conductivity was recorded with a YSI 33 SCT device, and pH was measured with a WTW 330/SET-1 digital pH meter. Vertical profiles were recorded for the mentioned variables except pH, which was measured at 10 cm.
Air temperature records were obtained from the National Institute of Agricultural Technology (INTA) Colonia Benítez, which is located 5 km from the lakes.
2.3. Data Analysis
Water variables from both lakes, from both experimental ponds (A and B), and from ponds and natural lakes were compared with one-way ANOVA and post hoc LSD Fisher tests using InfoStat statistical software [21] . The data were log (x + 1) transformed if necessary to reduce heterogeneity of variances.
Variations in the water variable profiles (temperature, dissolved oxygen and electrical conductivity) between the ponds and between the floodplain lakes were tested using MANOVA at a significance level of p < 0.01 [21] .
3. Results
3.1. Limnological Conditions in Floodplain Lakes
Except for the fact that dissolved oxygen was significantly higher in Lake El Puente than in Lake San Nicolás (Table 1), we did not find limnological differences between the floodplain lakes. Lake El Puente connects more frequently with the Paraná River than Lake San Nicolás, and the dissolved oxygen content of these lakes varied between 2.6 and 6.3 mg∙L−1 and between 0 and 5 mg∙L−1, respectively (Figure 2(a)). Anoxic conditions were found during the summer due to the dense cover of E. crassipes and the high temperature.
No significant differences in temperature, electrical conductivity or pH were observed between the lakes during the study period (Table 1, Figures 2(b)-(d)). Minimum values were registered in June, when the Paraná River was in its high water period. The highest values of water temperature occurred in February (23.8˚C in Lake San Nicolás and 27˚C in Lake El Puente, Figure 2(b)) and were coincident with the maximum air temperature (Figure 3).
The maximum values of electrical conductivity (340 µS∙cm−1) and pH (8.23) were higher in Lake San Nicolás than in Lake El Puente (290 µS∙cm−1 and 7.98 units, Figure 2(c) and Figure 2(d)).
During the study period, the mean air temperature ranged between 9.3˚C in September and 31.4˚C in December (Figure 3).
The MANOVA results suggested that there were no significant differences between the floodplain lakes (F = 2.32; p < 0.1032).
3.2. Limnological Conditions in Experimental Ponds
The vertical profiles of the limnological variables at 8 a.m. and 2 p.m. were significantly different in both ponds (Table 1). During the study period, dissolved oxygen in pond A was always lower than that in pond B (below 4 mg∙L−1 and below 7 mg∙L−1 at 8 a.m. and 2 p.m. respectively), and anoxic conditions were noted at the bottom (Figure 4(a) and Figure 5(a)).

Figure 3. Mean daily variations of air temperature at the study area.
Differences in water temperature between pond A (with water hyacinth cover) and Pond B (without waterhyacinth cover) ranged between 2.1˚C and 3.6˚C at 8 a.m. and between 0.1˚C and 6.4˚C at 2 p.m. (Figure 4(b) and Figure 5(b)). The highest values were found at the water surface. In both ponds, the temperature reached its minimum at 8 a.m. and 2 p.m. in June (Figure 4(b) and Figure 5(b)), while the maximum values were recorded at 2 p.m. in February and March (pond A and pond B, respectively).
Electrical conductivity (Figure 4(c) and Figure 5(c)) was always lower in pond A than in pond B; the maximum values were recorded in January in both ponds (550 µS∙cm−1 in pond A and 820 µS∙cm−1 in pond B) at 8 a.m. and 2 p.m. (Figure 4(c) and Figure 5(c)).
Due to the influence of the cover plants, the water remained more acidic in pond A than in pond B (Figure 6(a)), with pH values ranging between 6.39 and 7.71 at 8 a.m. and between 6.55 and 7.65 at 2 p.m. (Figure 6(b)). This effect was more prominent in the spring and summer.
The MANOVA results suggested a significant difference (p < 0.0001) between ponds with and without water hyacinth, both at 8 a.m. (F= 185.54) and at 2 p.m. (F = 225.49).
3.3. Comparisons between Lakes and Ponds
The differences between the lakes (San Nicolás and El Puente) and the conditions of both ponds were analyzed by comparing the measurements carried out at a depth of 0.2 m at 8 a.m.
There were no significant differences in temperature and pH values between the lakes and pond A (Table 1). Dissolved oxygen was significantly lower (maximum of 2.15 mg∙L−1) and electrical conductivity was significantly higher (between 150 and 318 µS∙cm−1) in pond A than in both lakes (Table 1) due to the river overflow in these natural environments.
Dissolved oxygen, electrical conductivity and pH were significantly higher in pond B (without E. crassipes) than in the lakes (Table 1). The water temperatures were similar during the study period in the floodplain lakes and pond B, and no significant differences were found (Table 1). However, pond B registered the maximum absolute value (28.8˚C, Figure 2(b) and
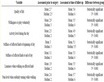
Figure 4(b)).
4. Discussion
Our results suggest that a dense cover (70% - 90%) of floating meadows composed of E. crassipes produced Table 1. Mean values and standard error of limnological variables of the floodplain lakes and ponds during the study period. Letters different indicate means statistically different (Fisher’s test, p ≤ 0.05).
(a)
(b)
(c)
Figure 4. Variability of dissolved oxygen (a) temperature (b) and electrical conductivity (c) values in pond A (with E. crassipes) and pond B (without E. crassipes) at 8 a.m.
changes in dissolved oxygen content, showing a variability pattern that differed from the pattern observed in lakes with submerged vegetation [22] . This plant succeeds in the occupation of both floodplain lakes with different river connectivity [4] [19] and ponds. Generally, less connected lakes have a denser cover of floating meadows than more connected lakes [20] . Under these conditions (less than a 20% cover), oxygen depletion was not found due to water circulation and diffusion of O2 from the air into the water. When E. crassipes plants occupy the entire water surface, they stop the horizontal circulation of water [3] , and during the summer, anoxic conditions were produced. In addition, the decreased oxygen concentration varied directly with the increase in temperature. Thus, we partially accepted the first hypothesis.
The most commonly documented effects of floating meadows are lower dissolved oxygen concentrations [7] [8] [23] [24] . During the decomposition of plant debris in a floodplain lake with a dense cover of E. crassipes, the oxygen concentration remained near zero [15] . Oxygen deficiency caused by aquatic vegetation causes fish mortality in floodplain lakes and in the main course of some tropical South American rivers [25] . Due to light interference, water hyacinth meadows clearly restricted phytoplankton growth [26] [27] and periphyton production [10] .
When comparing floodplain lakes that differ in the extent of water hyacinth cover, the current study found significant differences only in dissolved oxygen concentration. In contrast, other studies in subtropical lakes characterized by oversaturation of dissolved oxygen and dominated by submerged plants and phytoplankton showed that water hyacinth had only small effects on temperature and electrical conductivity [28] .
In the experimental ponds, a dense cover of E. crassipes reduced the water temperature up to 6.4˚C, which was noticeable in the summer and in the afternoon. In the floodplain lake with less E. crassipes cover (El Puente), the highest absolute value of temperature occurred in summer and was 3.2˚C above the maximum value recorded in the floodplain lake, which had greater water hyacinth coverage. The thermal damping effect produced by plants was negligible only in the winter. Differences in electrical conductivity between ponds with and
(a)
(b)
(c)
Figure 5. Variability of dissolved oxygen (a) temperature (b) and electrical conductivity (c) values in pond A (with E. crassipes) and pond B (with E. crassipes) at 2 p.m.
(a)
(b)
Figure 6. Variability of pH units at 8 a.m. (a) and 2 p.m. (b).
without E. crassipes indicated electrolyte capture by the plants. In the summer, CO2 release during plant decomposition creates a reductive environment that decreases the water pH in the vegetated area.
We did not find changes in dissolved oxygen concentration, electrical conductivity or pH between the morning and the afternoon. This pattern differs from the one observed in environments with predominantly submerged plants, where high photosynthetic and respiration rates during the day and night yield large changes in dissolved oxygen and CO2 [22] [25] . Consequently, we rejected the third hypothesis.
These results suggest that it is important to include the thermal damping effect produced by floating meadows as a variable in models used to assess the effects of global climate change on wetlands. These floating meadows cover tens of square kilometers of floodplains, such as those of the Paraguay, Paraná and Orinoco Rivers. Our results indicate that water hyacinth produces a strong damping effect on the heating of water bodies, and these results are the basis for the development of spatial models to express results on an experimental scale. Our results show that when water hyacinth covers the entire surface of shallow lakes, it can produce a thermal difference of 2˚C - 6˚C during the summer. Moreover, our results may assist in the development of cause-consequence models by demonstrating the relevance of the effect of thermal damping produced by floating meadows. This thermal damping may have indirect effects on other communities because massive fish kills occur due to extreme temperatures and insufficient dissolved oxygen in water [25] [29] .
Acknowledgements
We thank Chet Van Duzer for him valuable suggestions and to our manuscript. María do Carmo Calijuri also contributed important comments for the discussion of the results. We thank the anonymous reviewers who provide useful comments on the manuscript. This study was supported by Projects PIP 11220100100486; PIP 11420100100215; PICT 2077-2008 (CONICET) and 2011Q001 SGCYT (UNNE).