An ideal degradable biomaterial for bone regeneration is expected to be not only degradation controllable but also bioactive [6]. In general, as an implant material, only its surface contacts with the host tissue directly, thus this portion of the material plays a central role in determining its degradation resistance, biocompatibility and bioactivity [10]. The surface modifying methods are attractive technologies in gaining suitable coatings to improve magnesium implant bioactivity and protect it from fast in vivo corrosion and degradation [11-13]. Of all surface coatings, the calcium-phosphate coating is one of major coatings that have been studied extensively for modifying the surface of implanted devices, and have been successfully applied to the surface modification of Ti and its alloys in order to promote direct attachment of the surrounding hard tissue and to suppress the release of corrosion products into the human body [14]. At the same time, the compounds containing calcium and phosphate have also been studied to use for the formation of bioactive coatings and or for inhibiting magnesium alloys fast corrosion [15-19].
In recent years, an organic molecule, which is phytic acid (PA), is gaining increased interest because of the similarity in composition to phosphate and good biocompatibility [20]. The previous works have been reported about the application of PA conversion coating or corrosion resistant inhibitor for the paint or pigment systems on magnesium alloys [21-23]. But rare works reported the bioactivity of PA conversion coating on the magnesium alloys.
In this study, AZ31 magnesium alloy was modified with PA solution through immersion method, which is expected to prepare the PA coating on magnesium alloy and improve the surface corrosion resistance as well as the surface bioactivity. The in vitro corrosion properties of the AZ31 magnesium alloys with PA coatings were evaluated by electrochemical corrosion tests, the in vitro biocompatibility and the surface bioactivity were assessed by short-term tests using the human osteosarcoma MG-63.
2. Materials and Methods
2.1. Sample Preparation
Commercial AZ31 magnesium alloys were provided by the National Engineering Research Center for Magnesium Alloy of Chongqing University. For the PA modification, plate samples with a dimension of 10 mm in diameter and 3 mm in thickness were cut from the magnesium alloy bars, all samples were mechanically polished by polishing machine (UNIPOL-800, Sheng yang Kejing Instrument Co. Ltd.) with sand paper no.1000, then ultrasonically washed in acetone for 10 min, followed by washing with absolute ethanol and distilled water in turn, finally dried with warm air.
Surface modification of magnesium alloys was carried out in PA solution. The samples were immersed in a 15 mg/ml PA solution (pH 6.0) for 10 min in order to obtain a PA surface coating. The PA solutions were prepared by dissolving PA (purity > 70%, purchased from Chongqing Medicines Co. Ltd.), the pH value of the solution was adjusted by adding sodium hydroxide solution (prepared by analytical grade sodium hydroxide and distilled water) as our previous report [22]. After immersion, the samples were thoroughly washed using running distilled water, dried in warm air.
2.2. Surface Morphologies Observation
The surface morphologies of magnesium alloys treated with PA solutions were observed using scanning electron microscopy (S-3400N,Hitachi, Japan). Energy dispersive X-ray spectroscopy (EDS, Thermo Electron Corporation) was used to analyze the chemical composition of the treated magnesium alloy surface. The relative quantification was done by fixing the acceleration voltages and data acquisition conditions.
2.3. In Vitro Corrosion Test
To evaluate the effect for corrosion property of magnesium alloys through PA treatment, the potentiodynamic polarization measurement in Hank’s solution was carried out to comparatively investigate the corrosion behavior of the bare alloy to the alloy with the surface coating. A three-electrode cell was used for electrochemical measurements. The counter electrode was made of platinum and the reference electrode was saturated calomel electrode (SCE). The exposed area of the working electrode (treated or untreated AZ31 magnesium alloy samples with PA solutions) to the solution was 0.785 cm2. All the measurements were carried out on an electro-chemical workstation (CS2350, CorrTest, China) at the temperature of 25˚C. The test milieu was Hank’s solution.
2.4. Cell Culture and Proliferative Analysis
Biocompatibility of the PA modified AZ31 was examined using immature osteoblast-like cell line MG-63 which was purchased from Shanghai Institute of Biochemistry and Cell Biology (Shanghai, China). The osteoblasts were cultured in Dulbecco’ s modified Eagle’s medium (DMEM) (Invitrogen Life Tech., Shanghai, China) containing 10% fetal bovine serum (Sigma), 1% PSF (100×, 10,000 U/ml Penicillin, 10,000 mg/ml Streptomycin and 25 mg/ml Fungizones) (Invitrogen Life Tech., Shanghai, China) under a humidified 5% CO2 air atmosphere at 37˚C. Cells were seeded on the top surface of each metal material (placed in a 24-well-plate) at a concentration of 10,000 cells/well.
A MTT test kit (C0009; Beyondtime, China) was used for cell proliferation analysis. The protocol was as the followings: PA modified or unmodified AZ31 were placed on the bottom of the wells of 24-well plates (3 well for each treatment), and cells were plated on the modified surface and cultured for 48 h, then 10ul MTT solution was added to each well and cultured for another 4 h, followed by 100 ul Formanzan per well and the cells were incubated at 37˚C for 4 h. The medium was removed and dimethyl sulfoxide (DMSO) was added to dissolve the formazan crystals. Finally, 200 ul solution from each well was transferred to 96-well plate and the absorbance was measured at 570 nm with a common ELISA reader.
Cell visualization was carried out by the following as the previous report [24] with slight modifications. The metal materials were washed with PBS (phosphate buffered saline, 10 mmol/L; pH 7.4), and blocked in 2% normal goat serum for 30 min at room temperature. Then incubated over night at 4˚C with the α-Tubulin primary polyclonal antiserum (sc-5546, Santa Cruz, USA) diluted 1:200 with Antibody Diluent (S3022, Dako Inc., Glostrup, Denmark). After several washes with PBS, the metal materials were incubated with AlexaFluor 568® goat anti-rabbit IgG (1:600; A-11011, USA) for 1 h, and counterstained with Dapi (H1200, Molecular Probes; USA) then examined under Olympus microscope (BX60, Japan).
Data of MTT analysis were showed as mean ± S.E., then analyzed with SPSS software (version 13.0; IBM, Chicago, IL) and χ2 was employed to compare the differences between modified and unmodified materials, a level of p < 0.05 was considered to be statistical significant.
3. Results
3.1. Microstructure of Surface Treatment Magnesium Alloy
The surface morphology and the EDS result of AZ31 magnesium alloy treated with PA solution were shown in Figure 1. Compared to the untreated AZ31 magnesium alloy, there was an obvious conversion coating formed on the treated magnesium alloy surface, its result of surface EDS analysis (as shown in Figure 1(a1)) indicated that the chemical elemental compositions were mainly Mg, Al, P, C, O and a small amount of Zn. The corresponding elemental compositions (Figure 1(b1)) of untreated AZ31 magnesium alloy were only Mg, Al, and Zn.
3.2. Corrosion Resistance of Treated Magnesium Alloys
The electrochemical polarization curves of the PA treatment AZ31 magnesium alloys in Hank’s solution were shown in Figure 2. The bare AZ31 magnesium alloy was set as control. As seen from Figure 2, the corrosion potential of the bare alloy was about −1.50 V. In contrast, the corrosion potential of treated magnesium alloy was a little positive, shifting to about −1.46 V. It was noted that the corrosion current density of the treated magnesium alloy was 2.193 × 10−3 mA/cm2, a little decrease than that of untreated alloy (2.547 × 10−3 mA/cm2). The results were summarized in Table 1.
3.3. Cell Viability/Proliferation
α-Tubulin immunocytochemistry was used to visualize the cytoskeleton and Dapi was used to visualize the nuclei of MG-63 cells, the results showed that MG-63 cells
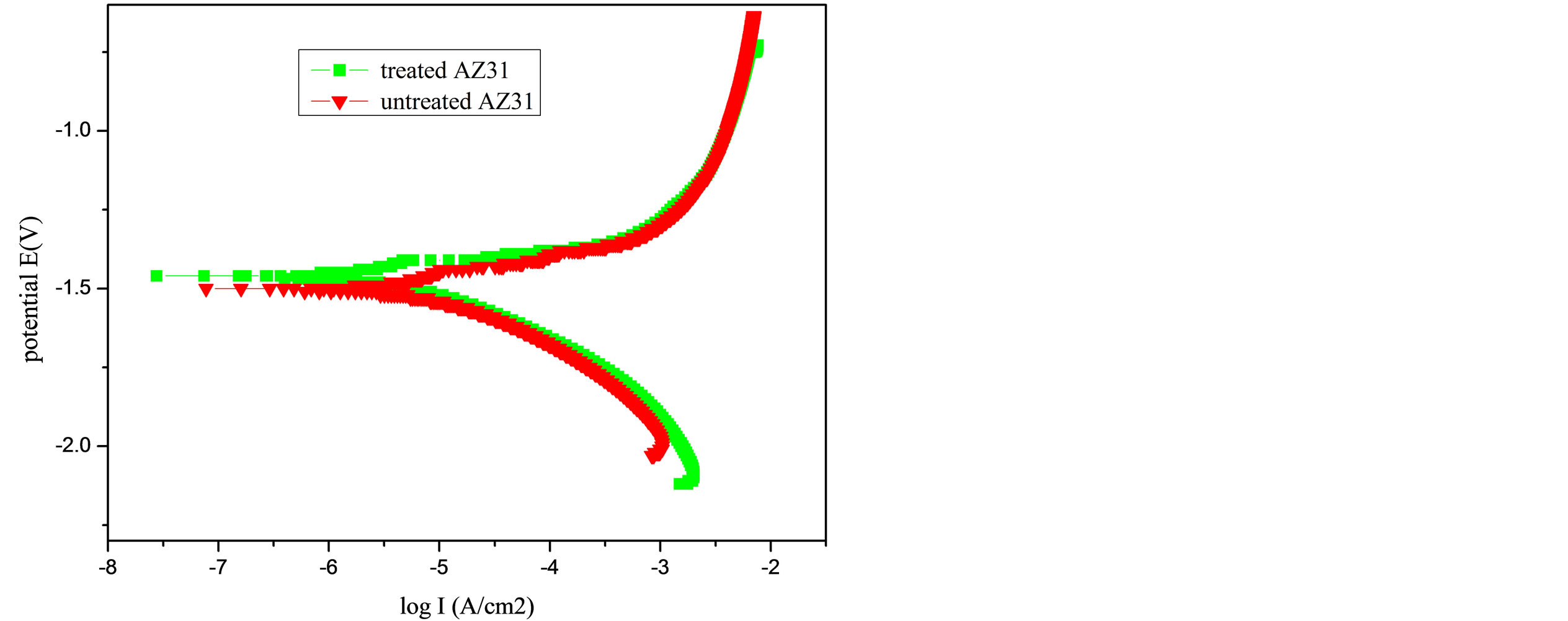
Figure 2. Potentiodynamic polarization curves of the PA treatment AZ31 magnesium alloys and the bare AZ31 control sample in Hank’s solution.

Table 1. Fitting results of polarization curves.
grew well on the metal surface as shown in Figure 3, either treated or untreated by phytic acid. The classic MTT analysis was employed to test the potential cytotoxicity of PA on the proliferation of MG-63 cells, the results revealed that cell proliferation was dramatically induced by PA modification when compared to the unmodified controls as shown in Figure 4.
4. Discussion
4.1. The Surface Coating and Corrosion Behavior
The Figure 1(a) showed that the coating layer was formed, and the EDS analysis (Figure 1(a1)) result indicated that the chemical elemental compositions of the coatings were mainly Mg and a small amount of Al, P, C, O, Zn. It is well known that PA (C6H18O24P6) consists of 24 oxygen atoms, 12 hydroxyl groups and 6 phosphate carboxyl groups [25-27]. It could deprotonate according to the equilibrium (1) (the completely deprotonated form is denoted in this work by Phy12−):
(1)
Due to its high density of negatively charged phosphate groups, PA, in its various deprotonated forms, has the ability to bind or chelate multivalent cations by coordinate bonds through reaction with paired oxo-dianions of the phosphoryl groups [25]. So, compared to the control (Figure 1(b1)), the three EDS signals of carbonPA Con
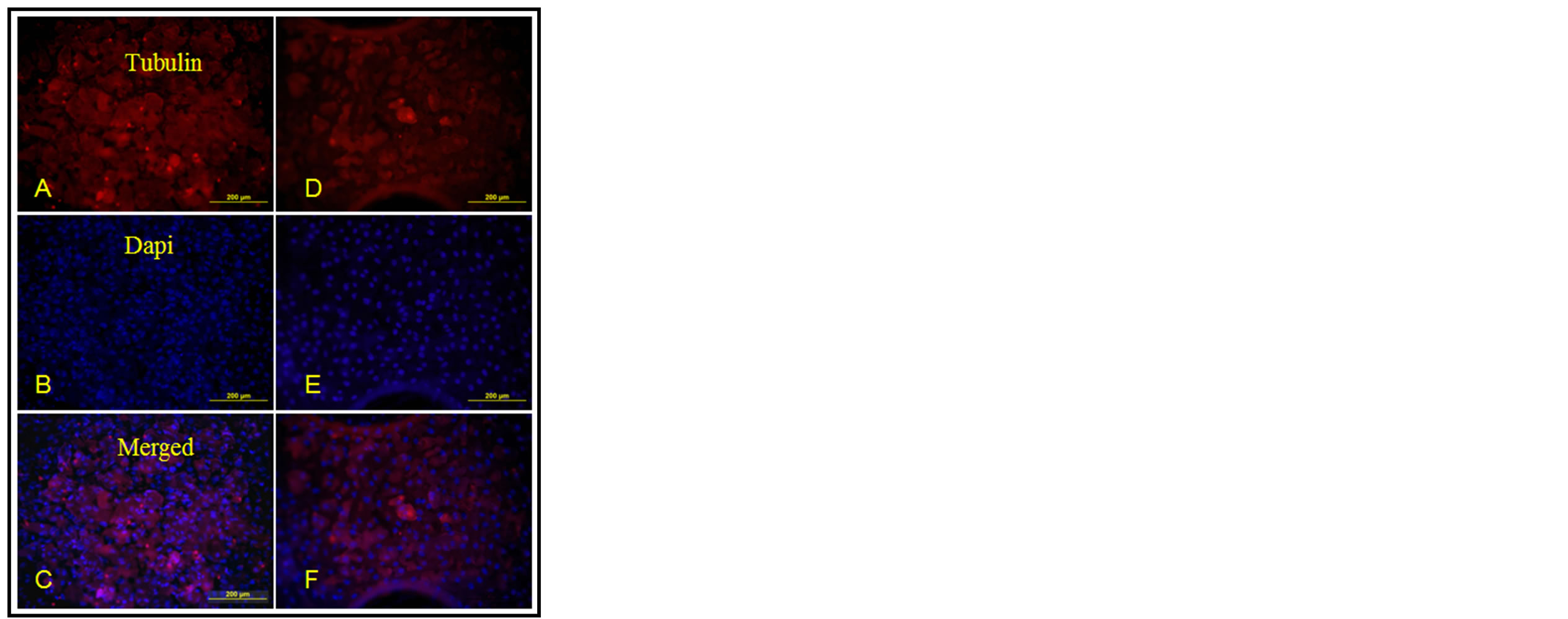
Figure 3. The results of the cytoskeleton and the nuclei of MG-63 cells on modified magnesium alloys ((A)-(C)) and the bare magnesium alloys ((D)-(E)).
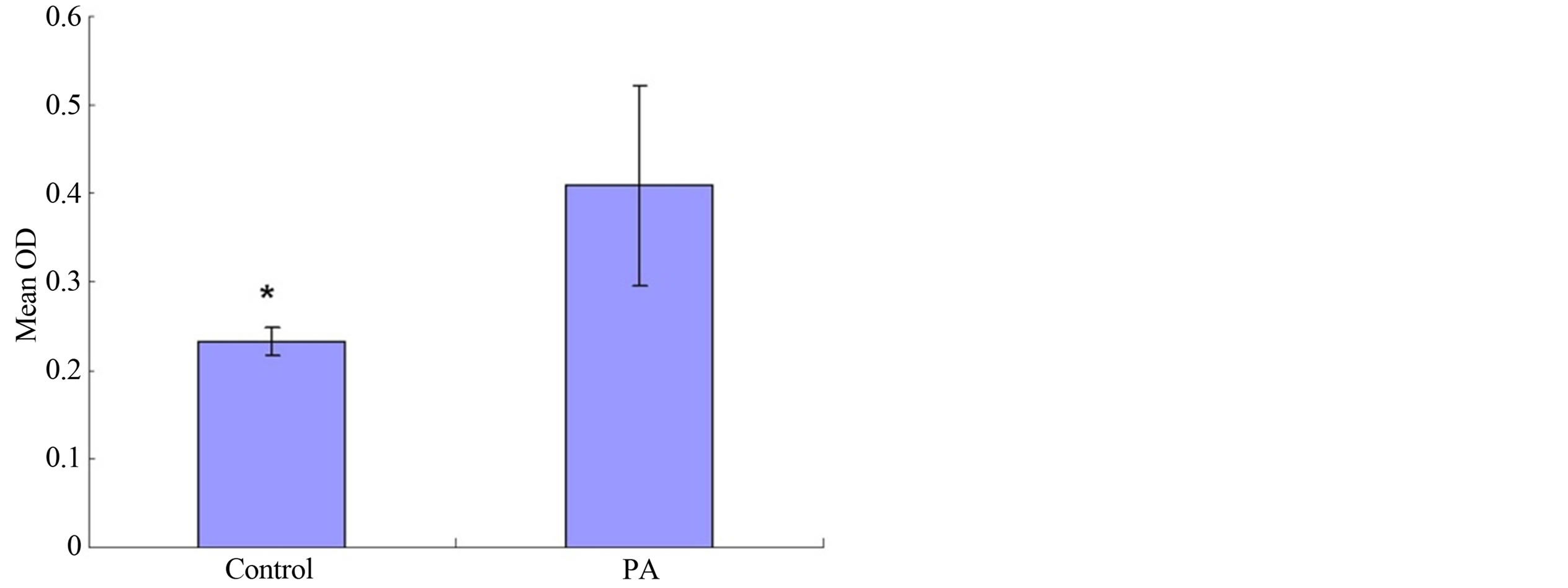
Figure 4. The results of the proliferation of MG-63 cells on PA modification magnesium alloys and the unmodified controls in MTT test.
oxygen and phosphorus suggested the presence of PA on the surface of AZ31 magnesium alloys. In other words, the PA conversion coating formed on the surface by chelating of metal cations (such as Al3+, Zn2+ and Mg2+) with PA and formation insoluble complexes [22,25].
Figure 2 presented the potentiodynamic polarization curves for the treated specimen and untreated control specimen. Compared with that of the control, the corrosion potential Ecorr was shifted positively about 0.04 V from −1.46 V to −1.50 V, and the corrosion current density icorr decreased from 2.193 × 10−3 mA/cm2 to 2.547 × 10−3 mA/cm2. Thus, after the PA treatment, the corrosion resistance of AZ31 magnesium alloy was slightly improved, the magnesium alloy also remained in an active state and further protection was necessary to prevent corrosion.
In aforementioned sections, the formed PA conversion coating showed a limited improvement to the corrosion resistance of AZ31 magnesium alloy. This finiteness may be explained by the nature of coating formation. According previous studies, Mg, Zn and Al atoms of the AZ31 magnesium alloy surface were oxidized into Mg2+, Zn2+ and Al3+ in acidic solution, then reversibly chelated with PA into different complexes (showed by MH(i-n)Phy, M is Mg2+, Zn2+ or Al3+) and deposited on the surface. All the complexes have the similar structure with phosphate [22,25,27], but there are different chemical composition and properties [25,27]. AlH(i-3)Phy compound has the higher stability and the lower solubility than those of other compounds ZnH(i-2)Phy and MgH(i-2)Phy [25]. It is right the stability and solubility difference of compounds (MH(i-n)Phy) as well as the amount difference of metals in alloy that result in heterogeneity of the PA coating, and affect the corrosion resistance further improvement. A schematic diagram (shown in Figure 5) may be a better description.
4.2. The Biocompatibility and Bioactivity of Surface Coating
The cytoskeleton and the nuclei of MG-63 cells visua-


Figure 5. Schematic illustration of formation PA coating on magnesium alloy surface: (a) the reaction between substrate and PA; (b) the formation and deposition of complexes (MH(i-n)Phy, M is Mg2+, Zn2+ or Al3+).
lized by α-Tubulin immunocytochemistry and Dapi staining showed that MG-63 cells grew well on the metal surface (Figure 3) either treated or untreated by phytic acid. On the other hand, the results of the classic MTT analysis revealed that cell proliferation was dramatically induced by PA modification when compared to the unmodified controls (Figure 4).
For biomaterials application, the surface bioactivity is mainly controlled by the surface properties. The chemical properties of the biomaterials play a very important role in good surface bioactivity. In order to improve the surface biocompatibility, various calcium phosphate coatings including octacalcium phosphate and hydroxyapatite have been successfully applied to magnesium alloys [28], because these compounds contain the same chemical composition or structure as the mineral composition of natural bone and the release of Ca2+ and PO43- during hydrolysis which can be utilized in the course of forming new bone [29]. The previous studies have indicated that the formation of PA conversion coating on AZ magnesium alloy surface is due to the chelating of Mg2+, Zn2+ and Al3+ with PA by coordinate bonds and deposition [21,22,25]. At the same time, the conversion coating may also slowly release metal ions (such as Mg2+, Zn2+ etc.) by reversibly [21,25]. Mg2+ is known to be active in cell adhesion mechanisms, and it is necessary for calcium incorporation [29]. It has already shown that Mg2+ substituted tricalcium phosphate could stimulate cell proliferation and the synthesis and secretion of collagenase [30,31]. Zn2+ also plays important roles in controlling the function of osteoblasts and increasing osteoblast adhesion and the alkaline phosphatase activity of bone cells [5]. The cytocompatibility of calcium phosphate/collagen composites doped with Zn2+ is acceptable and the composites can be considered as a promising biomaterial that has the potentials of promoting bone formation [29,32]. In addition, Zn2+ and Mg2+ in the coating may promote osteogenesis due to their stimulatory effects on osteoblastic cell proliferation and bone formation [33,34]. It has been reported that osteoblast-like MG63 cells are attached to and spread completely on a calcium zinc magnesium phosphate (CaZnMgP) matrix and this matrix appears to be comparable to the control group (hydroxyapatite matrix) [35,36]. Therefore, divalent cations such as Ca2+, Zn2+ and Mg2+ in the Ca-P layer also promote cell growth and differentiation [35-37]. According to our previous study, the PA coatings on AZ magnesium alloys surface have the similar chemical composition or structure with various phosphates, hydroxyapatite, and the natural bone. Hence, it could be speculated that it is such a similar structure that promote cell growth and proliferation, this similar chemical composition or structure may be the nature of the biological activity caused by PA treatment AZ31 magnesium alloys.
5. Conclusion
In this study, the surface PA coating was successfully prepared on AZ31 magnesium alloy by immersion modification method. But the corrosion resistance of AZ31 alloy was not significantly improved by PA modification. Its corrosion potential positive shifted only about 0.04V (from −1.50V to −1.46V), and the corrosion current decreased also only 0.354 mA/cm2 (from 2.547 × 10−3 mA/cm2 to 2.193 × 10−3 mA/cm2). However, the coating exhibited similar chemical composition or structure to that of various phosphates, hydroxyapatite and the natural bone. It could promote cell growth and proliferation; improve the surface bioactivity of magnesium alloy significantly, which provided a novel alternative for speeding up the early bone response synchronously to magnesium alloys implants.
Acknowledgements
The authors acknowledge the financial support provided by the Scientific and Technological Research Project of CQ CSTC (No. 2010AB5116).
NOTES