The Potential Bioeffects of Extremely Low Frequency Electromagnetic Fields on Melatonin Levels & Related Oxidative Stress in Electric Utility Workers Exposed to 132 kV Substation ()
1. Introduction
There is wide spread apprehension regarding the bioeffects of Extremely Low Frequency Electromagnetic Fields (ELF-EMFs). The conclusions drawn from various studies are controversial issues with conflicting reports. The role of melatonin in pathophysiology of occupational exposure to ELF-EMFs is not well established [1-4]. However, altered patterns or suppression of melatonin have been reported to coincide with sleep disorders, jetlag, depression, stress, reproductive activities and some cancers particularly breast cancers, melanoma, colon cancer, lung cancer and leukemia [5,6].
The ubiquitous excessive exposure of electromagnetic fields (EMFs) is posing a health concern due to melatonin disruption [7]. The potential effects of EMFs on human health by ELF-EMFs emitted from power lines and domestic wiring remain to be investigated. Some reports believe the monitoring of health risks on human population exposed to ELF-EMFs as a high priority research need [8].
There have been several epidemiological studies of the possible health effects associated with environmental exposure to Electromagnetic fields, emitted by power cables and electric substations. However, certain investigations have provided sufficient mechanistic information relevant to the assessment that melatonin suppression may be responsible for various biological effects [9].
Based on the epidemiological association between residential exposure to ELF-EMFs and childhood leukemia, the International Agency for Research on Cancer (IARC) classified ELF-EMFs as a “possible human carcinogen” [10]. Hence, to rule out the controversy in this study, the subjects exposed to EMFs from occupational environs of high voltage substations were investigated.
2. Materials and Methods
The male electric utility workers (n = 142) from high voltage substations operating at 132 kV were considered for the study. These subjects were involved in maintenance and installation of powerline distribution at electricity transmission network in Hyderabad, India. They are professionally categorized as linemen, casual labor and hotliners (live-line workers). Detailed questionnaire for designation, duration of exposure in years, frequency of exposure in hours/day and vicinity from substation were noted. The healthy control population (n = 151) was chosen with age, gender and socioeconomic status to match with controls.
2.1. Proforma/Questionnaire
Age, gender, diet, smoking, exercise and recent infection were used as criteria for the selection of both exposed and control populations. Detailed questionnaire on work characteristics, psychological perceived stress and nonspecific symptoms experienced by exposed populations were gathered by 94% of participants Questionnaire on subjective symptoms related to EMFs exposure included: Self-assessment of non-specific symptoms such as headache, dizziness, tinnitus, visual impairments and sleep disturbances.
2.2. Sample Collection
After taking informed consent, 5 ml of peripheral blood was collected in the morning between 9 AM to 10 AM from each of the 142 exposed subjects and 151 controls by venepuncture into a sterile disposable syringe. The whole blood was placed in ice to prevent exogenous damage and processed in the laboratory within an hour of collection to study the following parameters.
2.3. Lipid Peroxidation-Thiobarbituric Acid Reactive Species (TBARS) Assay
Malondialdehyde [MDA] is the stable end product of lipid peroxidation and an efficient parameter for lipid oxidative damage [11]. Formation of MDA was assessed by quantification of thiobarbituric acid reactive species (TBARS). The protein free plasma obtained after centrifugation was treated with thiobarbituric acid (0.33%) in boiling water bath for 30 minutes to form an 1:2 adduct which is measured at 535 nm with spectrophotometer (Schimadzu UV-160A, Cat No:204-04550-01, Made in Japan). MDA standards were prepared by using 1,1,3,3- tetraethoxy propane. The concentration of MDA equivalents in nmol/ml in samples were interpolated from the standard curve.
2.4. Estimation of Serum Nitric Oxide
As NO is an unstable compound, it is rapidly converted to nitrates and nitrites in the body, hence their concentration is parallel to NO levels. Total production of Nitric Oxide (NO) was determined by assaying for nitrite and nitrate. Nitrate and nitrite concentrations were then estimated by the Griess method [12]. In this method nitrate is first reduced to nitrite which is treated with sulfanilamide and N1-napthyl-ethylene diamine. The coloured compound is formed after which its characteristic absorption spectrum was determined on spectrophotometry. The optical densities of the samples were then obtained on a spectrophotometer (Schimadzu UV-160A, Cat No: 204-04550-01, Made in Japan) at 540 nm.
2.5. Enzymatic Determination of Superoxide Dismutase
Superoxide Dismutase (SOD) activity was estimated [13]. One unit of SOD is that which causes a 50% inhibition of the rate of reduction of 2-(4-iodophenyl)-3-(4-nitrophenol)-5-phenyltetrazolium chloride (INT) under the conditions of the assay. This assay was linear from 30% to 60% inhibition. The within-run CV for SOD of 1185 U/g haemoglobin in erythrocyte suspension was 4.1% (n = 5).
2.6. Enzymatic Determination of Glutathione Peroxidase
The activity of Glutathione Peroxidase (GpX) was determined by the modified method [14]. 1 unit of glutathione peroxidase will form 1.0 µmol NADP+ from NADPH per min at pH 8.0 at 25˚C. Enzyme units were defined as the number of micromoles of NADPH oxidised per minute. Results were interpreted as International units per unit per gram of Hb (U/g Hb). The within-run CV for GpX of 7.4 U/g hemoglobin in erythrocyte suspension was 4.8% (n = 5).
2.7. Enzymatic Determination of Catalase
The catalase assay method defined [15] and modified [16] was used in this study. Thus the activity of catalase, a cellular antioxidant enzyme, present in biological sample was estimated by measuring its ability to decompose hydrogen peroxide formed due to metabolic reactions into water and oxygen. Hydrogen peroxide has absorbance at 240 nm and the decrease in optical density (OD) is an indicator of the decomposition of hydrogen peroxide by catalase. The decomposition of the substrate (H2O2) was recorded spectrophotometrically (Schimadzu UV340) at 240 nm for 60 s and 30 s time interval. Catalase activity was expressed as K/g hemoglobin.
2.8. Melatonin Assay (Direct Radio Immuno Assay)
Plasma Melatonin was quantitatively measured by Direct Radioimmuno Assay (RIA) [17]. The amount of 125Ilabelled antigen bound to the antibody is inversely proportional to the analyte concentration of the sample. Standard melatonin (1000 pg/l)- LDN GmbH & Co. KG, Nordhorn, Germany was used in this assay. When the system is in equilibrium, the antibody bound radioactivity is precipitated with a second antibody in the presence of polyethylene glycol. The precipitate was counted in a gamma counter.
3. Statistical Analysis
Descriptive statistics were applied for calculating the distribution of various characteristics. The test samples were coded during processing and decoded at the time of statistical analysis. For statistical evaluation, observations on each parameter for each group were pooled and mean ± SD (SE) was calculated. Student’s t-test (paired and unpaired comparisons), one way Analysis of Variance (ANOVA) after log transformation were performed to evaluate various differences among the exposed group. The latest significant difference (LSD) procedure for multiple comparisons was applied when three or more groups were compared.
An unpaired students t-test and one way ANOVA were applied to determine the significance of various biochemical changes (levels of MDA, NO, Catalase, SOD, GpX) between different categories of exposed subjects and their respective controls. Uniand multivariate logistic regression for quantitative risk assessment of symptoms in relation to exposure level, after adjusting for confounders. Multiple regression analysis was done to study the effects of confounding factors. Statistical software SPSS 16 (South Asia Pvt. Ltd., Bangalore, India) was used to carry out statistical analysis.
4. Results
The demographic details of the occupationally exposed high voltage substation (132 kV) workers (ELF-EMFs exposed) and control group are shown in Table 1. As indicated, the age distributions of the entire population

Table 1. Demographic characteristics of the occupationally exposed high voltage substation (132 kV) exposed and control.
were similar and comparable between groups. The socioeconomic status and lifestyle factors were matched between exposed groups and control. Most of the subjects in the exposed group and control belonged to low and middle socioeconomic status.
4.1. Analysis by Exposure Characteristics
The sources of exposure include high voltage substation (132 kV). The electric utility workers are exposed for a mean (SD) duration of 9.27 (7.23) years. The workers are exposed to field intensities at an average of 9 h per day. The linemen, casual labor and hotliners (live-line workers), had variations in their exposure characteristics.
4.2. Results of Oxidant and Antioxidant Status
Table 2 shows the results of TBARS assay in the ELFEMFs exposed and control groups. The mean MDA concentration (nmoles/ml) of the ELF-EMFs exposed subjects showed significant increase in MDA levels when compared with controls. The levels of serum nitric oxide, determined as nitrites or nitrates showed an increased level of serum NO in the exposed groups (p = 0.033). The anti-oxidative enzyme, erythrocyte catalase activity was estimated in controls and exposed. ELF-EMFs exposed group was found to have significant altered catalase levels when compared to controls (see Table 2).
The effect of duration of work in years in electric utility workers was significantly high in MDA, NO and catalase levels among the subgroups above 12 years of exposure (see Table 3). Frequency of daily exposure in hours had no significant effect in oxidative stress parameters among the sub-groups (see Table 4). The oxidative stress markers showed no relationship with exposure variables as assessed from regression analysis. The
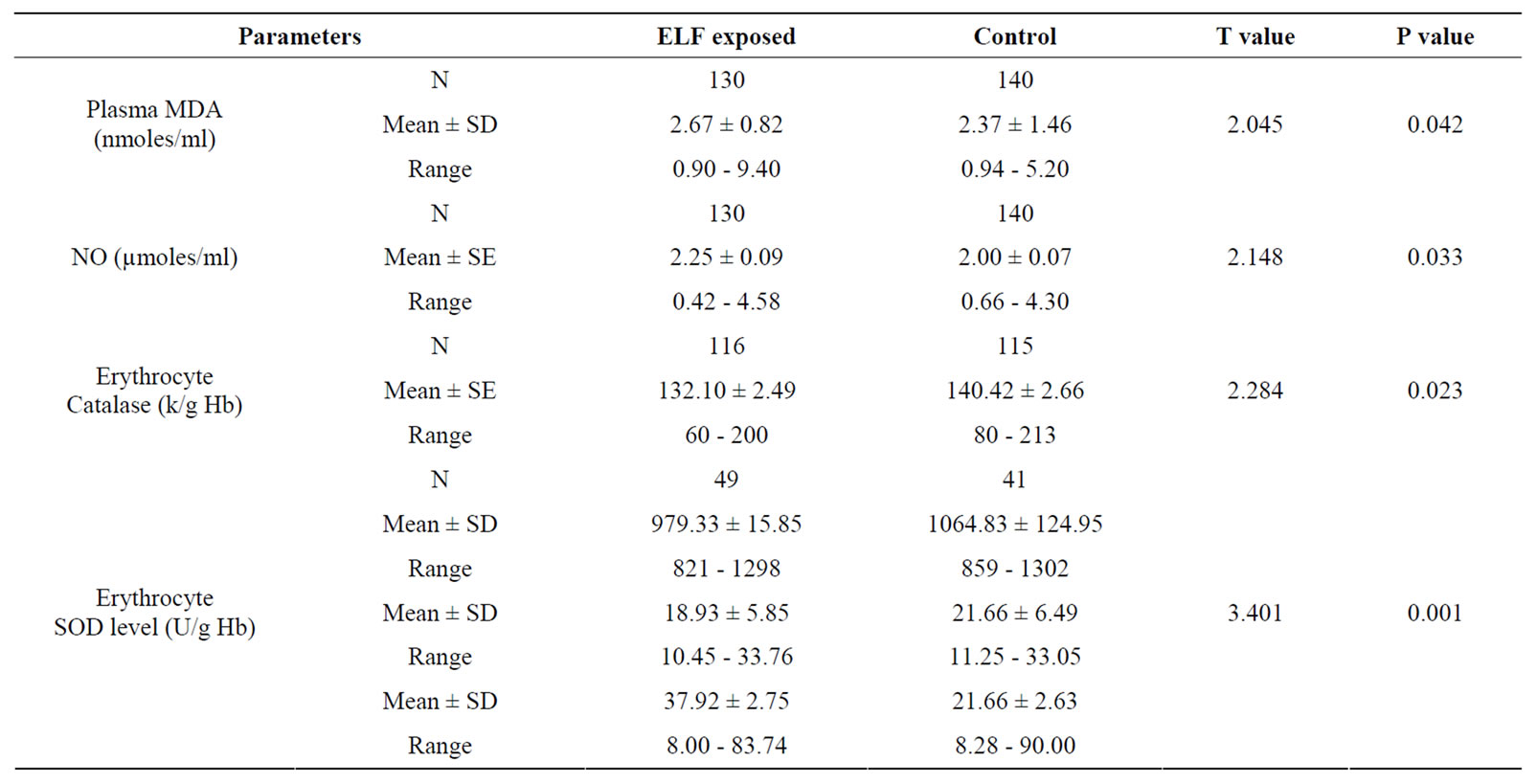
Table 2. Results of TBARS assay in the ELF exposed compared with control group.
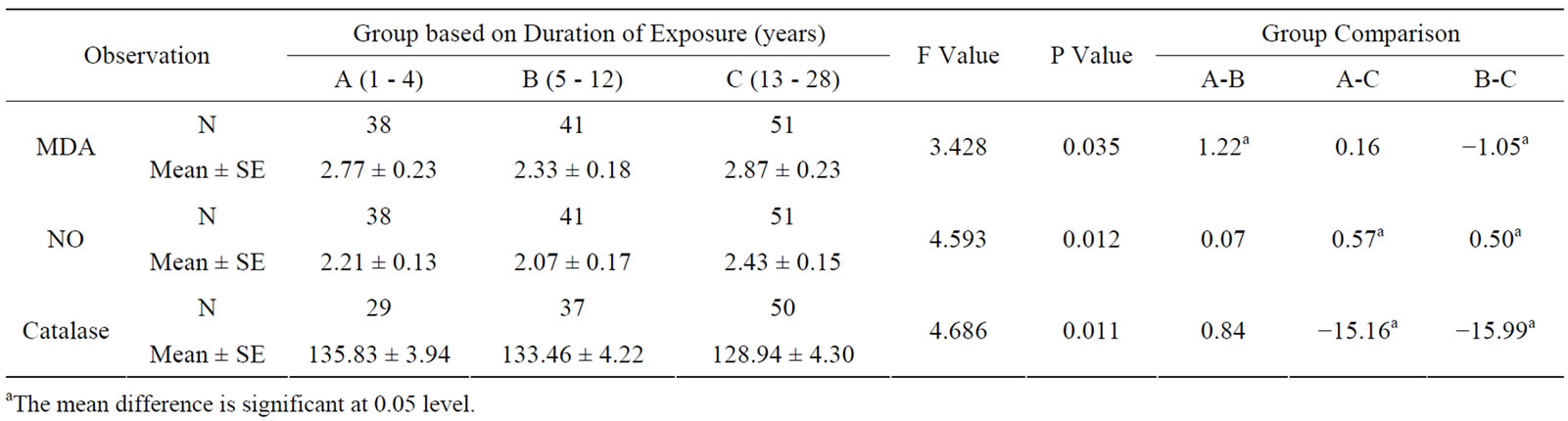
Table 3. Oxidative stress in relation to duration (years) of occupationally exposed electric utility workers.

Table 4. Oxidative stress in relation to frequency of exposure (hours/day) to occupational ELF-EMFs of electric utility workers.
high exposure group of hotliners had significantly different oxidative stress parameter levels compared to other sub-groups (see Table 5). The maintenance workers had comparatively a higher range of MDA levels when compared with the low exposed sub-group.
The antioxidative enzyme levels of erythrocyte SOD and GpX were assessed in the exposed and controls. The activity level of SOD in ELF-EMFs exposed group was found to be significantly low (p < 0.001) when compared with normal controls (Table 2, Figures 1 and 2). The
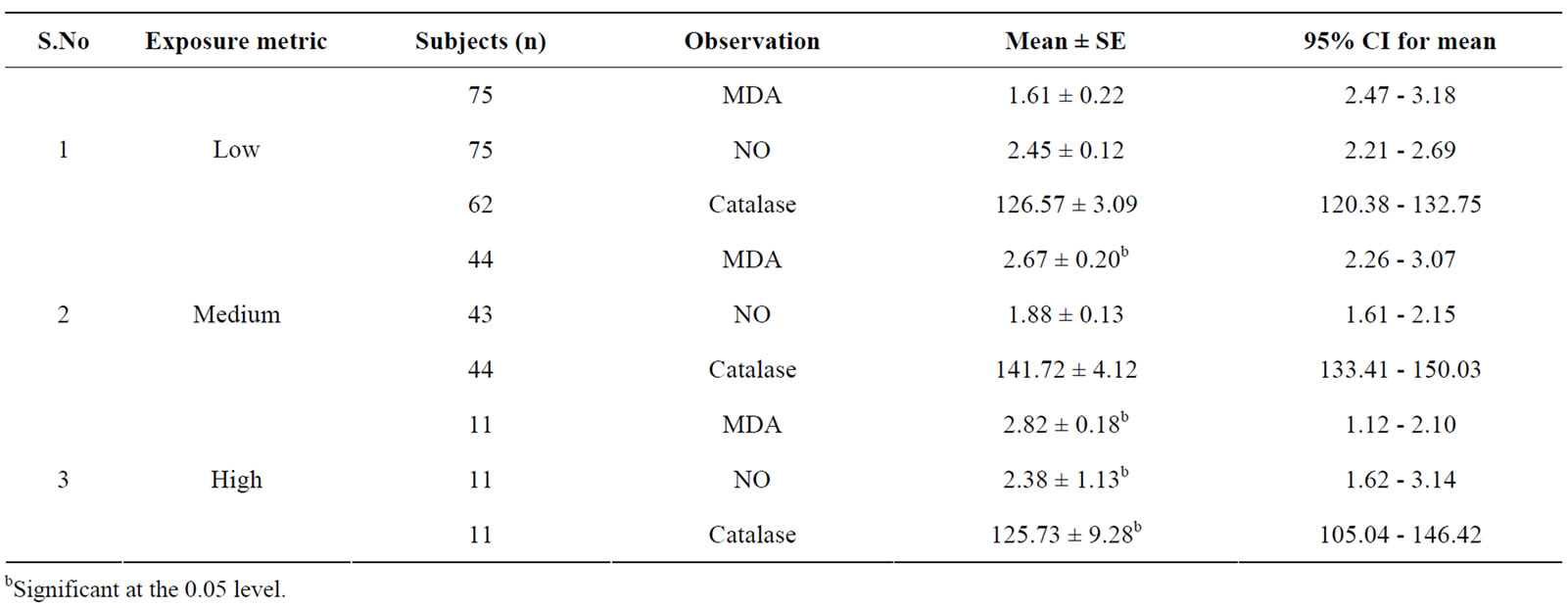
Table 5. Oxidative stress in relation to exposure metric of occupationally exposed electric utility workers.
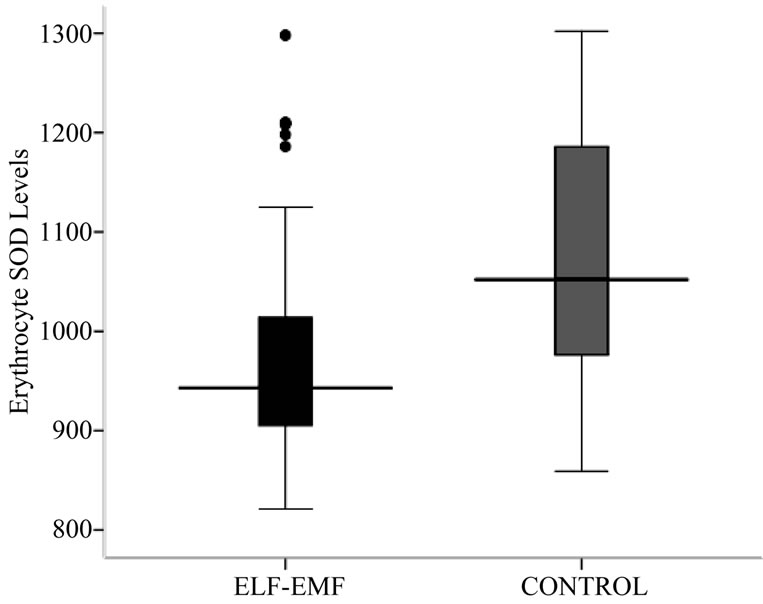
Figure 1. Box-plot showing Erythrocyte SOD (U/g Hb) concentration.

Figure 2. Box-plot showing Erythrocyte SOD (U/g Hb) concentration.
mean ± SD of SOD concentration in high exposed group (953.67 ± 49.85) was found to be significantly lesser from low exposed (1108.10 ± 78.50) and medium exposed (1115.30 ± 68.06). The mean difference of SOD concentration between the sub-groups of low and medium exposed was also significant. The regression analysis showed no relation of SOD concentration with exposure durations (R2 = 0.01, p = 0.529) and frequency of exposure (R2 = 0.001, p = 0.884) (Table 2, Figures 1 and 2).
As in Table 2 and Figure 2, a significantly low (p < 0.05) activity level of GpX in exposed group was noted when compared with control group. The mean ± SD GpX concentration of high exposed group was found to be significantly lesser from low exposed and medium exposed. The mean difference of GpX concentration between the sub-groups of low and medium exposed was also significant. The regression analysis showed no relation between GpX concentration with the exposure durations (R2 = 0.002, p = 0.843) and frequency of exposure (R2 = 0.026, p = 0.818).
Tables 6 and 7 indicate the results of ANOVA in sub groups based on duration of exposure in years and hours per day respectively. It was observed that there is a significant increase (p = 0.05) proportionate to the duration of exposure both in years and hours.
Table 8 outlines that the melatonin levels did not differ markedly between the exposed and controls. The concentration of the high exposed (19.60 ± 9.65) was significantly lesser from those of low (36.27 ± 15.00) and medium exposed (31.94 ± 21.82) subjects. The mean values ranged between 8.0 to 90.74 pg/ml (Figures 3 and 4). The mean concentration of melatonin did not differ significantly with differences in age group (see Figure 5). Univariate analysis of variance showed the significant changes in melatonin levels in sub-groups based on dura

Table 6. Results of ANOVA in sub-groups based on duration of exposure.

Table 7. Results of ANOVA in sub-groups based on duration of exposure.

Table 8. Melatonin levels (pg/ml) in exposed and control subjects.
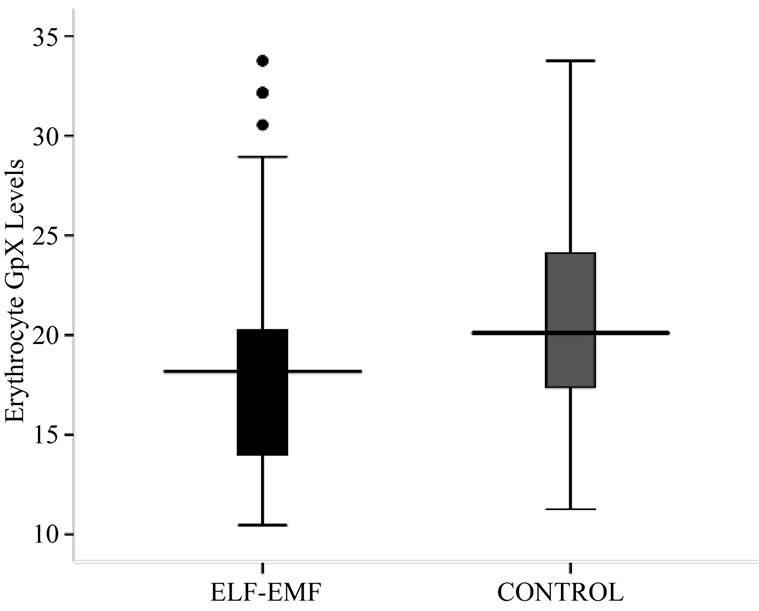
Figure 2. Box-plot showing Erythrocyte SOD (U/g Hb) concentration.
tion and frequency of exposure. Increasing duration of exposure was found to be significantly related to the suppression of melatonin (F = 3.490, p = 0.069). The regression analysis showed no relation between melatonin concentrations with frequency of exposure (see Figure 6).
5. Discussion
The subjects exposed to specific non-ionizing radiations

Figure 3. Distribution of circulating melatonin concentration (pg/ml) in substation workers.

Figure 4. Effect of magnetic field exposure levels in melatonin (pg/ml) concentration.

Figure 5. Linear trend of melatonin levels with increasing duration of exposure (years).
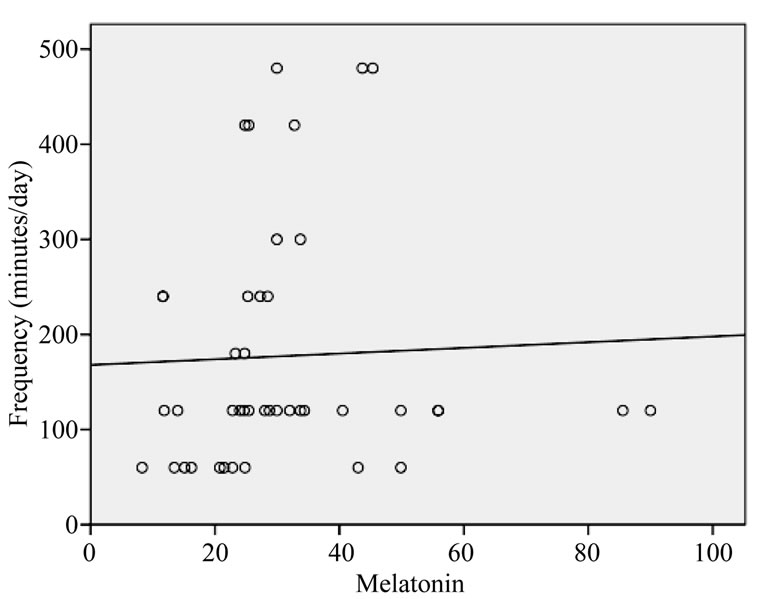
Figure 6. Linear trend of melatonin levels with increasing frequency of exposure (minutes/day).
were screened while care was taken to exclude subjects majorly exposed to other occupational/environmental agents such as chemicals and pollutants. The present study has shown the possible bioeffects of EMFs on subjects occupationally exposed to high voltage substation.
5.1. Bioeffects on Occupational Exposure to ELF-EMFs
The hypothesis that chronic exposure to residential and or occupational ELF-EMFs may cause adverse health hazards has led to a great deal of research. Epidemiological reports particularly on increased risk of childhood leukemia, breast cancer and neurological disorders had been a subject of National and International reviews [18]. There has been a steady increase in investigations examining the genetic effects of EMF exposure in in vitro experimental designs and in vivo animal models. Howeververy few epidemiological studies have directly evaluated the cause of such bioeffects. As studies on genotoxicity are highly relevant in evaluation of carcinogenicity, the present study is focused on such an issue in occupational settings of ELF-ELFs exposure. Moreover, in India hardly such studies are conducted to evaluate the oxidative stress effects of magnetic fields on high voltage substation workers. Nonetheless, it has been suggested that EMFs may induce cell specific activation processes, which in turn activate signal transduction pathways causing excessive reactive oxygen species (ROS) formation/ cell cycle disturbances. These disturbances are supposed to have the potential to disrupt DNA integrity [19].
5.2. ELF Effect on Oxidant-Antioxidant Balance
The MDA levels in erythrocytes of ELF exposed subjects suggest a statistical significance in lipid peroxidation (LPO) activity. Owing to the great number of biological actions of free radicals, the LPO activity could have an important implication in the comprehension of the biological stress response to ELF-EMFs. Oxidative DNA damage and LPO activity in exposure to ELF-ELFs was reported in certain in vivo experimentations and animal models. A time-dependent significant higher TBARS levels (p < 0.001) and induced DNA damage (p < 0.05) in the exposure group as an effect of ELF magnetic fields (50 Hz, 0.97 mT) is observed [20].
Glutathione peroxidase (P < 0.001) and superoxide dismutase (P < 0.007) showed a decrease, while an increase in catalase (P < 0.005) was observed. Malondialdehyde (P < 0.003) showed an increase. Erythrocyte MDA and glutathione (GSH) contents, can through some light on the role of melatonin as an antioxidant and its effect on oxidative stress [21].
The total nitrite concentration was statistically higher in the substation workers. In support to the findings of the study, statistically higher concentration of total nitrite in samples from volunteers exposed to magnetic field than in pre-magnetic field samples were reported [22]. Altered nitric oxide production in prolonged ELF-EMFs exposure at intensities of exposure limits, determined by International Commission on Non-Ionizing Radiation Protection (ICNIRP) for public and occupational exposure [23]. Few studies have reported the role and involvement of nitric oxide in magnetic field effects [24- 26]. Negative results on nitric oxide levels are shown in other studies [27]. It is also shown that nitric oxide synthase is supposed to be stimulated by the EMF exposure [28-30]. This compound can be involved in the stimulation of lipid peroxidation indirectly. This hypothesis could also explain the increased TBARS production in our exposed group.
Altered SOD and GpX activities showed the exposure effects of ELF-EMFs. However, no significant changes in catalase levels could be noted. A diminution in the activity of SOD and GpX were proven by ZwirkaKorczala et al. [31]. The results indicated a relation between exposure to ELF-EMFs and oxidative stress. Some studies have shown increased oxidative-stress in human fibroblasts under ELF-EMFs exposure [32], mammalian cultured cells and human erythrocytes [33]. In support to our results, altered production of superoxide dismutase activities are noted in magnetic field exposure studies [25].
Furthermore, few studies have focused on the involvement of oxidative stress in the action mechanism of EMF exposure [34]. Exposition to electric fields (50 Hz) of Sprague-Dawley rats did not change the antioxidant activity and lipid peroxide level of unstressed animals but decreased the plasma peroxide level in stressed rats [35,36]. Recently, Zwirska-Korczala and colleagues [31] using preadipocytes, showed a diminution in the activity of superoxide dismutase after 24 h and 48 h of EMF exposition (180 - 195 Hz). The early increase in the TBARS concentration observed in our study could be the result of the reduction in the activity of antioxidant enzymes or/and the increase of free radical production.
Since free radicals are able to damage many biomolecules, including DNA, enzymes, lipids and proteins, we can hypothesise that modulation in antioxidative enzyme activities observed in our study can be related to overproduction of oxidants NO and MDA under ELFEMFs exposure. However, the precise mechanisms involved in the effect on oxidative stress need further investigations.
Nevertheless, the altered oxidant-antioxidant imbalance was highly pronounced in live-line workers. Oxidative damage was evident in this sub-group of electric utility workers by 1) increase in malondialdehyde (MDA) and nitric oxide (NO) levels, 2) decrease in superoxide dismutase (SOD) and glutathione peroxidase (GpX) activities and 3) suppressed melatonin levels.
5.3. ELF-EMFs Effect on Neuroendocrine Hormone and Stress Parameters
The effect of ELF-EMFs on occupational exposure over the production of pineal gland hormone, melatonin was evaluated. The mean melatonin concentration of the exposed was significantly different from those of healthy male individuals. The high exposed workers have suppressed melatonin levels against the low exposed and control group.
Very few studies are carried out on melatonin secretion in occupationally ELF-EMFs exposed subjects. Some authors observed an effect in electric workers in substations with 3-phase conductors, but not in substations with 1-phase [37]. Findings from studies on occupational ELF-EMFs exposure to influence melatonin secretion have also shown negative results [38-41]. A decrease in melatonin secretion was observed in subgroups of the population exposed to high-power lines [42].
When the data was analysed by different exposure characteristics, differences were noted in sub-groups employed as live-line workers. In support to our data, the consequence of duration and intensity dependent exposure effect of ELF-EMF on melatonin levels is reported [41]. Another occupational study showed reduced 6-OHMS in a group of female garment industry workers [32]. Similar trend in melatonin concentration was seen in the sub-group of workers exposed to different levels of ELF-EMFs. Some results [39] showed decrease in melatonin with increasing age.
We propose the role of melatonin as an antioxidant to explain the biological effects of EMFs. The rationale behind our study is that the increased free radical production in response to magnetic field exposure might result in suppressed circulating melatonin levels. The suppression of melatonin with extended free radical lifetime may also enhance DNA damage [6,43]. Even in female workers exposed to magnetic fields, Nocturnal 6-hydroxy melatonin sulfate levels were suppressed [44]. This hypothesis could have an important implication for the possible health effects associated with exposure to ELF magnetic fields in the public and occupational environments.
5.4. Relevance of Electromagnetic Field Exposure Indices
For the duration estimates, we systematically used the number of years of exposure, which are expected to provide better estimates of the duration of long-term exposure. Furthermore, intensity is estimated separately from daily exposure or working hours per day. The results from our earlier report on cytogenetic assays suggested the induction of DNA damage probably due to genotoxic action of ELF-EMFs [6]. This genotoxic effect may also increase on co-exposure of occupational magnetic field densities with other environmental mutagen.
Men employed as live-line workers, may be at risk, with indications of increased incidences of cytogenetic damage and stress. Thus the live-line workers in high voltage substation were identified as vulnerable groups. The live-line workers are positioned in direct contact (bare hand) with the live components. In practice liveline work is potentially very hazardous and involves high risk. Though the safeties of workers are ensured, the impact of their routine procedures in maintaining live-lines might result in adverse stress effects.
5.5. Magnetic Field Intensities in 132 kV Substation
The magnetic field measured in the present study represents the prevailing field strength in high voltage (132 kV) substations at Hyderabad, India. The mean occupational magnetic field exposure in the 132 kV substation was 3.5 µT (SD ± 2.01), while environmental non-occupational exposure was 0.0015 µT (SD ± 0.001). The range of ELF-MF measured in the present study was consistent with the levels observed in other occupational studies [45]. The magnetic field intensities represent the prevailing exposure levels in high voltage (132 kV) substations of Hyderabad, India.
6. Conclusions
The findings that ELF-EMFs may influence melatonin levels and oxidative stress have been an issue of debate for over two decades. In our study, the biological relevance of melatonin and related oxidative stress is well documented. The present study provides an insight to look into the possible bioeffects of electromagnetic exposure of ELF-EMFs in the occupational environments. The study can be applicable to consider melatonin and oxidative stress as biomarkers.
This investigation is also important for the safety measures to be taken by the personnel working in substations. The proper evaluation of the EMFs in such environments allows mapping the levels around the electromagnetic devices. It would help to choose tools with low emission of magnetic fields, to design accurately and correctly the working areas and to minimize the exposures. Thus, this study of bioeffects on humans exposed to the vast expanding electromagnetic pollution may be noteworthy from public health perspective.
7. Acknowledgements
The authors express heartfelt thanks to the University Grants Commission (UGC) for funding this Major Research project.