Heavy Metal Remediation in Agoè Nyivé Landfill Soils: Enhancing Stability through Organic Amendments ()
1. Introduction
In developing countries (DCs), burying waste in landfills often does not provide the necessary guarantees to avoid the pollution of water, soil and air [1] . In addition, some sites were set up without those in charge of understanding the problems they could cause, other very sophisticated sites required heavy investments without valid reasons [2] .
The establishment of landfills in developing countries must be carried out according to certain rules and provisions which make it possible to avoid negative impacts on the environment, which amounts to controlling fermentation phenomena by controlling the nature and flow of buried waste and liquid and gas flows.
Solid waste landfilled is very rarely inert. Numerous physicochemical and biological reactions occur not only between the waste and the receiving environment (soil, geological bedrock, groundwater, etc.), but also within this waste itself [3] [4] . Attempts at solutions proposed or solutions currently being implemented are limited only to the burying or filling of lowlands (uncontrolled landfills) on the outskirts of cities while the population of these cities continues to increase [4] [5] . Landfilling has the advantage of eliminating large volumes at reasonable costs [6] , but very often in developing countries such as Togo, no prior study is carried out in relation to the pollution of soils and groundwater from landfills, notably the old final landfill of Agoè Nyivé in Lomé.
The old final landfill of the city of Lomé, the capital of Togo, is located in the Agoè Nyivé district. This landfill was a former sand and gravel quarry exploited by the indigenous population before 1990, and was not developed before the waste was buried. It was closed in 2018 due to saturation. Several studies have shown that uncontrolled landfills have a negative impact on the environment. The observation is that the waste which, in the past, was essentially organic (livestock effluents, food waste, plant residues, etc.), now contains a very heterogeneous range of products (sewage sludge, green waste, food waste, livestock and industrial effluents, paper, plastic and metal packaging, etc.) [7] [8] . This waste poses many ecological problems and therefore requires prior treatment. The studies were carried out to limit or even reduce the impact of trace metal elements on the environment, specifically focusing on stabilization a process which reduces the dangerous potential and the leachability of materials (waste, soil, etc.) by converting its contaminants into less soluble, mobile or toxic forms [9] .
The primary aim of this study is to investigate the retention of heavy metals by introducing cattle manure into soil samples obtained from the final Agoè Nyivé landfill in Lomé, Togo. The focus is on demonstrating the capability of trapping or stabilizing heavy metals within organic-rich soil following the application of manure. The cattle manure was chosen for this study because of its availability at the Agronomic Center of the University of Lomé and the fact that much work has shown the capacity of manure to retain heavy metals in soils by adsorption.
2. Materials and Methods
2.1. Sampling
The samples are collected at the final landfill site in the city of Lomé. This landfill has an area of approximately 6 ha with an average depth of 6 m. The samples were taken during the rainy season. Only the parts covered with waste and accessible were sampled. Samples were taken at the surface and at a depth at 6 different points. At the sampling points, the age of buried waste ranges from 1 to 5 years. These samples were taken using the drilling truck. The pipes used to drill are made of steel and have a diameter of approximately 100 mm. The samples were taken from the same locations as those taken at the surface [10] (Photo 1).
Composite samples are formed by grouping samples from each sampling point (surface and depth samples) into one sample.
The cattle manure which was used as an amendment was collected from the agronomic center of the University of Lomé. For this purpose, 50 g of each sample are amended with 20% manure, i.e. a ratio of 1/5. The amendment was carried out for six months. Then the samples were reduced to powder with a particle size of <2 mm before carrying out the analyses.
2.2. Determination of Physicochemical Parameters
The pH and electrical conductivity (EC) were measured on a suspension of 10 g of sample in 25 ml of distilled water. After 30 minutes of stirring using a magnetic stirrer, the suspension was left to stand for two (2) hours before carrying out the measurements. The devices used are the CRISON pH 25 type electrode pH meter and a WTW brand LF 330/SET conductivity meter [10] .
The technique used to determine the total organic carbon (TOC) content in the samples is that of Walkley and Black [2] [11] [12] . This technique is based on the oxidation of organic carbon by excess potassium dichromate (K2Cr2O7) in the presence of sulfuric acid at 95% concentration.
The content of organic matter (OM) was determined by the loss on ignition method through calcination in a Nabertherm electric oven controller P 320 (MB1) than heat 30˚C - 3000˚C, of 10 g of samples at 550˚C for four hours. Before calcination, the sample is placed in an oven at 105˚C until constant weight in order to eliminate free water [4] [13] .
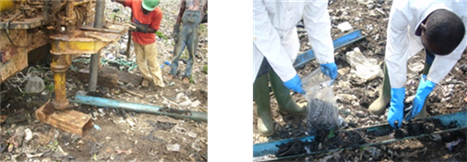
Photo 1. Taking soil samples at the final Agoè Nyivé landfill.
The cation exchange capacity (CEC) of a soil is the total quantity of cations that this soil can adsorb on its complex and exchange with the surrounding solution under well-defined pH conditions. The cation exchange capacity of the samples was determined by the Metson method using ammonium acetate at pH = 7 [11] [14] .
Total nitrogen (NTK) is determined by the Kjeldahl method [2] [11] . The nitrogenous organic matter in the sample is mineralized by hot concentrated sulfuric acid in the presence of a catalyst. The nitrogen in mineral and organic compounds is transformed into ammonium sulfate during the oxidative attack. In a nitrogen distiller, the ammonium ion is then displaced by 40% sodium hydroxide and entrained in steam then fixed with 2% boric acid and finally titrated with 95% sulfuric acid at 0.02 N in the presence of the colored indicator helianthin.
The device used for infrared (IR) is a Bruker Fourier transform infrared spectrometer of the VERTEX 70 type. The measurements were carried out in diffuse reflection. The samples were packaged in the form of pellets diluted in KBr. The fine sample is ground in KBr (0.099 g of KBr and supplemented with the sample to 0.100 g) to mitigate energy losses. The radiation penetrates inside the sample, undergoes reflection, refraction, diffusion and absorption effects and is then reemitted in all directions of a hemispherical space. For this study, the absorption spectra were carried out in the mid-infrared region, corresponding to wavenumbers (ν = 1/λ) between 400 cm−1 and 4000 cm−1) [10] .
The device used for X-ray fluorescence is the Pioneer S4 X-ray fluorescence spectrometer. This device was used to do elemental analysis of soil samples from the final landfill. The samples were subjected to intense X-ray radiation, which has the effect of ionizing the atoms by ejecting the electrons located on the layers close to the nucleus. The elements were expressed as oxide [10] .
The content of heavy metals was determined using flame atomic absorption spectrometry (SAA) after hot mineralization of the samples with aqua regia (1/3 HNO at 69% for 2/3 HCl at 36% - 38%). The mineralization was carried out in Pyrex Matras previously rinsed with 10% nitric acid; the samples were heated to 200˚C until the acid evaporated. 25 ml to 50 ml of demineralized water are then added to the mineralized residues and then filtered through a flat membrane with a porosity of 0.45 μm. The metal concentrations were then measured using a Thermo Electron type flame atomic absorption spectrophotometer with a variable detection limit depending on the elements measured in mg/l (Pb: 0.01; Cd: 0.0005; Ni: 0.004; Cu: 0.001; Zn: 0.0008) [2] [15] .
For the sequential Extraction of heavy metals, the application of the procedure of BCR (The community Bureau of Reference) aims to allocate each pollutant according to three fractions (to which one can add a fourth unextracted called residual fraction) which are characteristic of the mode and the fixing intensity of each pollutant on the compartments of the soil [4] [15] [16] .
In sequential BCR extraction, the soils samples were treated with a chain of reagents and determined the elemental concentration into fractions, linked with different mineralogical phases. The different fractions of the sequential extraction are: The exchangeable and acid-soluble fraction (F1); the reducible fraction (F2); the oxidizable fraction (F3) and the residual fraction (F4). The Scheme shows the different steps of the extraction [15] (Scheme 1).
2.3. Statistical Processing
The mean value of each treatment, as well as the corresponding variance and standard deviation, were calculated taking into account the three repetitions carried out. The data thus obtained were subject to statistical analysis with the Assistat Software Version 7.6 Beta (2012). Analysis of variance (ANOVA) was performed. In cases where significant differences were found (p < 0.05), means were separated using the Tukey test (p < 0.05) [4] .
![]()
Scheme 1. Different steps of the sequential extraction.
3. Results and Discussions
3.1. Contents of Physicochemical Parameters
Several physicochemical parameters have an influence on the mobility of heavy metals in the soil. These parameters were determined on composite samples that did not undergo any treatment (UCS), manure samples (MS) and composite samples treated with manure (MTCS).
The pH constitutes a factor whose role is crucial for the mobility of metal ions, because it influences the number of negative charges that can be put into solution [16] . At the end of the manure treatment (T = 6 months), the pH of the samples increases and is around 8.80. This optimum pH obtained at the end of the treatment is favorable to the retention of the heavy metals studied since most of them can become mobile at acidic pH. The study has shown that an acidic pH leads to the dissolution of metal salts, the dissolution of retention phases, the desorption of cations and the adsorption of anions [9] .
The electrical conductivity significantly increased during the treatment. This shows that the manure provides enough mineral salts to the samples, since the electrical conductivity (EC) of the manure is 8975 µS/cm, much higher than that of the untreated samples which is 1444 µS/cm (Table 1). However, the addition of manure made it possible to increase the EC of the samples, hence a strong presence of mineral salts which can facilitate adsorption by ion exchange with metal ions; because the more conductive a solution is, the more it is rich in mineral salts.
The ability of a material to retain cations by physical adsorption is called cation exchange capacity. The cation exchange capacity in the samples with manure addition evolved after six months of treatment. This is due to the action of manure which has a high exchange capacity (42.48 meq/100g). The variation in pH during the treatment could also lead to this increase since, the higher the pH, the more the CEC increases and the more the quantity of cations adsorbed increases. The increase in the CEC following the treatment of composite samples with manure could, in fact, allow the reduction of the mobility of metals because the retention of heavy metals becomes significant with the increase in the CEC.
![]()
Table 1. Physico-chemical parameters of composite, manure and composite samples treated by manure.
UCS = Untreated Composite Sample; MS = Manure Sample; MTCS= Manure Treated Composite Sample.
The analysis results show that the treatment of the samples with manure (43.62% organic matter content) allowed their enrichment in organic matter. Organic matter increased from 11.32% ± 0.57% to 25% ± 0.56% after treatment with manure (Table 1). Given that organic matter participates effectively in the retention of metallic species which can be in exchangeable form, the manure amendment will then make it possible to retain more metallic elements in the soil. Organic matter, through humic substances, can also fix metal ions by complexation and efficiently chemisorb metal ions with a high degree of selectivity thus forming strong ionic or covalent bonds [9] .
The C/N ratio of treated samples decreased compared to untreated samples. This would be due to a greater contribution of nitrogen by manure compared to carbon. This low C/N value (1.31) in the samples after six months of treatment highlights significant microbial activity in these samples, thus promoting the availability of mineralized organic matter.
3.2. Infrared Spectroscopy and X-Ray Fluorescence
Infrared spectroscopy can be used as a mineralogical analysis tool. It is also applied to solids with very complex structures such as shales, coals, humic acids, and other sediments. This technique makes it possible to characterize the functional groups present (OH, COOH, C=O, C-H, C=C bonds, etc.) and to monitor any modifications that they may undergo.
Infrared spectroscopy analysis of composite samples from the landfill reveals the presence of a small clay fraction within the waste. The bands observed at 3698, 3677 and 3621 cm−1 and between 1100 and 500 cm−1 confirm this hypothesis. Only three relatively intense bands characterize the waste. They are located at 3423, 1632 and 1433 cm−1. The band at 1433 cm−1 (Figure 1) is probably that of carbonates.
![]()
Figure 1. Infrared spectra of samples from the final discharge.
We also noted that the band located at 1086 cm−1 corresponds to the Si-O vibration of the clay contained in the waste. The deformation bands appear at 538 cm−1 for Si-O-Al vibrations, at 470 cm−1 for Si-O-Mg vibrations and at approximately 428 cm−1 (Figure 1) for Si-O-Si vibrations.
X-ray fluorescence is both a qualitative and quantitative analysis. The results show the presence of chlorine, oxides of silicon, aluminum, iron, titanium, manganese, sodium, calcium, sulfur and potassium. Other oxides such as magnesium, copper, zinc, nickel oxides, etc., were detected but given their low content, they were not represented in the figure. The presence of all these elements can be explained by the diversity of waste buried at the landfill [5] . The analysis reveals a strong presence of silicon oxide with a rate of 50% in all samples (Figure 2). This demonstrates a strong presence of sand in the landfill samples. The presence of magnesium (MgO), sodium (Na2O), potassium (K2O) and calcium (CaO) oxides confirms (Figure 2) the alkaline pH found in the samples, since these oxides promote an increase in the pH of a medium [17] . Likewise, the presence of oxides in the soil plays an important role because they have a particular affinity for certain heavy metals (Cu, Zn, Ni, Pb, etc.) which they can adsorb in large quantities [9] . Metal oxides can be good adsorbents that can increase the retention capacity of trace metal elements in a soil [18] [19] .
3.3. Total Heavy Metal Levels
Lead is toxic and is one of the least mobile metallic elements in the ground. It is frequently found in household waste in developing countries because of its use as a constituent element of batteries [20] . The results obtained show that its content in the samples is 130 mg/kg. This fairly high content would be due to the presence of organic matter which is not negligible and to an alkaline pH which can promote the retention of metals; because high pH often induces the formation of precipitated species which can limit the solubility and bioavailability of all ionic species [21] . The lead content in the samples exceeds the thresholds recommended by the AFNOR NF U 44-041 standard (Table 2).
![]()
Figure 2. Determination of the elemental composition of samples.
![]()
Table 2. Total heavy metal content of composite soil sample in mg/kg of dry matter.
Cadmium is very toxic in all its forms (solid, vapor, salts, organic compound). In soil samples from the landfill, the cadmium content is 3.83 mg/kg and exceeds the recommended standard.
The copper content is 230 mg/kg. This value exceeds the AFNOR standard and this is partly due to the basic pH found in the samples which would have favored the retention of copper in the soil matrix, since copper becomes very mobile from pH = 5 [1] .
Among the heavy metals studied, zinc has very high concentrations in the landfill soil. This is linked to its presence in various items used around the world. This content is 700 mg/kg (Table 2). It is well above the thresholds recommended by the AFNOR NF U 44-041 standard. Certain components of waste sources of the presence of zinc found during the physical characterization of waste intended to be buried in the landfill [5] , such as metals, categories of paper, cardboard, textiles, sanitary textiles (baby care cream based on zinc oxide), special waste (batteries, aerosols) would have led to a high presence of zinc in the landfill soil. This strong presence of zinc in the soil can also be linked to the alkaline pH of the samples; because generally, the acidic pH leads to the dissolution of metal salts, the dissolution of retention phases, the desorption of cations and the adsorption of anions [22] .
Determining the total concentration of a potentially toxic element provides little information on its actual toxicity [23] . Any metal, regardless of its minimal content or not, must be taken into account in relation to the danger it represents to the environment since the toxicity of an element is directly linked to the chemical form in which it is present and therefore to its speciation.
3.4. Evolution of Heavy Metal Content in Manure-Treated Samples
3.4.1. Distribution of Lead in the Different Fractions
After six months of treatment, the distribution of lead in the different fractions of the soil shows that it is mainly present in the oxidizable (F3) and residual (F4) fractions (Figure 4). This would be due to the addition of manure which would have caused the concentration of Pb in these different fractions of the soil. In these fractions, heavy metals are difficult to mobilize because they are firmly bound. In household waste, it is associated with the residual fraction [24] . To evaluate the mobility of lead in the samples following the treatment, we added the percentage contents of the F1 and F2 fractions and calculated the average. For this purpose, the mobilizable portion of lead in the samples after six months of treatment with manure is estimated at 14.39%. This proportion decreased compared to untreated samples which is approximately 78.06%. The treatment of composite samples with manure allowed the concentration of lead in the oxidizable and residual fractions, thus leading to the reduction of its mobility.
3.4.2. Distribution of Cadmium in the Different Fractions
The cadmium contents relative to the exchangeable and acid-soluble (F1) and reducible (F2) fractions are low (Figure 4). Cadmium is very present in the oxidizable fraction and moderately present in the residual fraction. We note that there is a significant change in the distribution of cadmium in the different fractions after six months of amendment (Figure 4) compared to the unamended samples (Figure 3). The mobilizable portion of cadmium is estimated at 11.52%. This shows that the oxidizable and residual fractions retain most of the cadmium in the samples at the end of the amendment. This accumulation of cadmium in the two fractions (F3 and F4) is due to the contribution of manure since, as listed previously, it has been shown that cadmium concentrates more in the exchangeable and carbonated fraction of soils [25] . This migration of cadmium from one fraction to another with the addition of manure was facilitated by its high mobility and its ease to have into exchange connections with several fractions of the soil.
3.4.3. Distribution of Copper in the Different Fractions
The results of sequential copper extraction in samples treated for six months are very remarkable. The copper contents relating to the exchangeable and acid-soluble and reducible fractions are almost zero (Figure 4). The distribution of copper is made only in the oxidizable and residual fractions. We notice that copper is more present in the oxidizable fraction. This is due to its great affinity towards this fraction [26] [27] . On the other hand, the migration of copper in the soil is also linked to the formation of organometallic compounds. Therefore, the complexation of copper by organic matter can explain the proportions of copper extracted. The addition of cattle manure, very rich in organic matter, allowed a very large accumulation of copper in the oxidizable fraction, thus reducing its mobility. The Cu contents in fractions F1 and F2 being zero, we can however say that the amendment has made it possible to considerably reduce the risks of copper migration towards the depths.
![]()
Figure 3. Distribution of heavy metals in the different fractions of untreated soil.
![]()
Figure 4. Distribution of heavy metals in the different soil fractions treated with manure.
3.4.4. Distribution of Zinc in the Different Fractions
Sequential extraction of samples treated with manure shows that zinc is mainly linked to fractions F1 and F2 (Figure 4). This is due to the fact that zinc was strongly adsorbed by iron, manganese and aluminum oxides, which would surely have specific adsorption sites where the metal ion would have attached [28] . The potentially mobilizable portion is estimated at 80.58%. This portion has decreased since it was estimated at 91.15% (Figure 3). All these rates are very important for estimating the risks of immediate pollution.
3.4.5. Estimation of the Evolution of the Mobility of Heavy Metals before and after Treatment
The metals present in the soil matrix behave differently depending on their position in the different fractions. In certain compartments of the soil, such as the oxidizable and residual fractions, the metals are solidly bound, which makes them difficult to mobilize. In other fractions such as exchangeable and acid-soluble (F1) and reducible (F2), metals are weakly adsorbed and therefore can easily be mobilized. However, we quantified the portion of mobilizable and poorly mobilizable metals contained in fractions F1 and F2 respectively. Figure 5 shows the variation in the mobilizable fraction of heavy metals in the manure-amended samples.
After three months of manure amendment (T1), the mobility of heavy metals decreases except that of zinc (Figure 5). This can be explained by the fact that the manure, with its high organic matter content (43.62%), would have led to the trapping of metals in the oxidizable fraction (linked to organic matter). The mobility of zinc has not decreased because, it is an element that has a high affinity to the exchangeable and acid-soluble fraction and generally its mobile fraction varies from 1 to 20% of its total content in the soil [29] [30] . After six months of amendment (T2), the mobility of all the metals studied decreases. This may be linked to the increase in the organic matter content at time T2 and therefore accentuated the phenomenon of metals being trapped in the organic matter. Copper having a great affinity towards organic matter [27] , we note that it is completely concentrated in the oxidizable and residual fractions, thus making its
![]()
Figure 5. Evolution of the mobilizable fraction of metals in the treated samples. T0 = 0 day; T1 = 3 months; T2 = 6 months.
mobility almost zero. We can indeed say that, the higher the organic matter content, the more the metals will be concentrated in the organic phase and will thus lead to a reduction in their mobility.
4. Conclusions
To reduce the risks of pollution from landfills on the environment, and as part of achieving the Sustainable Development Goals (SDGs), relating to the protection of the environment in general and natural resources in particular, it is essential to seek solutions to various pollutions. A heavy metal trapping study using the manure was carried out on soil samples from the household waste landfill. The treatment of soil samples with manure is followed from zero (0) to six (6) months. The addition of manure made it possible to increase the levels of certain physicochemical parameters such as pH, electrical conductivity (EC), organic matter (OM), cation exchange capacity (CEC) which have elsewhere an influence on the retention of heavy metals. On average, the pH went from 8.4 before adding manure to 8.8 at the end of the treatment. The electrical conductivity, organic matter and cation exchange capacity saw their contents increase at the end of the treatment compared to the samples before addition of manure. We also note the concentration of metals (Pb, Cd and Cu) in the oxidizable and residual fractions, thus allowing the reduction of their mobility, hence reducing the risk of pollution of these elements to the environment and especially to the water table. For zinc, although the treatment led to a reduction in the potentially mobilizable portion, the risk of immediate pollution remains significant since it is estimated at 80.58% after six months of treatment. The order of mobility of heavy metals is as follows: Zn > Pb > Cd > Cu.
The study carried out could continue beyond 6 months in order to better assess the long-term stability of heavy metals in amended soils.