Increasing the Efficiency and Level of Environmental Safety of Pro-Environmental City Heat Supply Technologies by Low Power Nuclear Plants ()
1. Introduction
In the modern world, the issue of energy consumption is becoming increasingly important. The increase in energy demand is the result of the development of industry and IT technologies, as well as an increase in population and efforts to reduce carbon emissions to ensure decarbonization of the energy sector, etc. At the same time, the exhaustibility of fossil energy resources and the climate heating of the planet have led to restrictions on the use of alternative energy sources. At the same time, under current conditions, the solution of energy and environmental problems on a global scale cannot be achieved by 2035 without nuclear energy [1] . The main task of further development of nuclear energy is to ensure environmental safety of the population under any internal and external conditions of operation of nuclear power plants (NPPs), including emergencies, spent fuel and radioactive waste management. Such environmentally safe development of nuclear power in the XXI century is closely related to the introduction of small nuclear power plants (SNPPs) [2] based on small modular reactors (SMRs). The most proven technologies for small nuclear power plants are SMRs with pressurized water, which are characterized by their designers as having a relatively high level of environmental safety. Their passive safety performance and geographical independence from the availability of cooling water sources allow the placement of SMRs in close proximity to heat supply consumers [3] [4] . At the same time, the use of the ASWR heat generation mode for heat supply of large cities with heating of network water with steam taken from the turbine allows saving fuel due to higher efficiency [5] . NPP-based district heating systems have already been established in Slovakia at Bohunice NPP, in Finland at Loviisa NPP, and at Beznau NPP in Switzerland.
2. Literature Review and Problem Statement
The relevance of small modular reactor (SMR) technology is evidenced by a number of factors: they can be used to generate basic electricity, heat for satellite cities, as maneuverable capacity for green energy, for electricity generation, desalination of drinking water, industrial-scale hydrogen production, and other applications of process heat [6] [7] .
The prospects for global nuclear power are associated with the implementation of the American SMR projects NuScale, SMR-160, and mPower [8] [9] [10] .
Compared to high-capacity nuclear reactors (more than 700 MW) [11] , SMRs may have higher environmental safety performance, the ability to maneuver power, and better meet the needs of the energy market. However, papers [12] [13] emphasize the need for an additional set of theoretical and bench-scale experimental studies to improve the reliability of the expected indicators of technological and environmental safety, as well as studies of effective heat transfer from the surface of fuel elements, and to ensure reliable calculation of thermal and hydrodynamic processes in turbulent coolant flows. It is also pointed out that it is necessary to physically substantiate adequate ideas about the nature of the emergency mode of impaired heat transfer, which can unpredictably occur on the surface of fuel elements even under the condition of continuous cooling.
The creation of efficient heat supply schemes is central to the list of urgent scientific and technical problems that are directly related to the priorities of ensuring operational and environmental safety and reliability of use for cities [14] [15] . In a number of European countries, this issue has long been recognized as a priority [16] . Paper [17] presents data on the experience of operating district heating systems based on operating NPPs in Switzerland and Slovakia and demonstrates their economic and environmental efficiency. At the same time, municipal heat supply from NPPs makes it possible to replace organic fuels for thermal power plants and thermal boilers and thereby reduce environmentally hazardous gas and aerosol emissions into the atmosphere, including greenhouse gas CO2. For example, the Beznau NPP has saved about 20,000 tons of liquid fuel per year thanks to the use of high-efficiency technology. In terms of the annual amount of environmentally hazardous gas pollution emitted into the environment, this amounts to: 50,000 tons of CO2, 100 tons of SO2, and 50 tons of NOx. The study also revealed the fact that various issues related to municipal heat supply from nuclear power plants remain unresearched [18] .
All known NPP heat supply projects envisaged schemes for independent connection of heat consumers to the heating network with a total thermal load of 430 MW. Design temperature in the supply heat pipe of the transit circuit of the heating networks was 140˚C and 60˚C in the return heat pipeline.
3. Purpose and Objectives of the Study
The aim of the study is to increase the efficiency of heat supply by improving the scheme. In practice, this will reduce the amount of fossil fuel consumption and increase the efficiency of heat supply to a settlement from a small nuclear power plant at any distance.
To achieve this goal, the following tasks were set:
· to analyze the possibility of using condensing turbines for heat supply to cities;
· to propose a scheme with the use of backpressure HPTs and heating of network water with hot steam to increase the heat supply capacity;
· to determine the efficiency of using a backpressure heat pump and a heat pump for the district heating system of a settlement from a small nuclear power plant.
4. Materials and Methods of the Study
The object of the study is to determine the feasibility of using the heat recovery mode by small nuclear power plants for urban heat supply with heating of network water with steam taken from the turbine.
Thermodynamic methods and methods of heat and mass transfer theory were used to analyze the efficiency of thermal processes and operating modes. The adequacy of the methods, the reliability of the proposed theoretical solutions, methods, and conclusions were assessed by comparing them with experimental operating data.
The methodological basis was based on regulatory documents, the experience of scientists, project documentation, and adapted methods of experimental research on operating turbines at various nuclear power plants.
5. Results of the Study of Small Nuclear Power Plants for Heat Supply
5.1. Analysis of the Possibility of Using Condensing Turbines for Municipal Heat Supply
Let’s consider the possibilities of SMR-160 for heat supply. Based on the previously mentioned ratio between the electric and thermal power of a condensing turbine, we obtain that with an electric power of 160 MW, the possible thermal power will be 32 MW (160 × 200/1000). It follows that SMRs, which by definition have an electric capacity of up to 300 MW, are not advisable to use for heat supply of large cities using the known technology. Relatively low thermal capacity is also shown by the results of the analysis of the thermal scheme of the second circuit of the NewScale ASMP [19] [20] , where heat supply for desalination is provided by heat recovery from the turbine.
We cannot ignore the rather advanced and expensive thermal scheme of the turbine unit presented in [21] , which can be effectively used for turbines with relatively high power. For the turbines that were planned to be used to equip the NewScale SMR, turbines from transport power plants that have been tested and have sufficient operating experience are more suitable. These turbines have one low pressure heater (LPH), two high pressure heaters (HPH), and a separator [22] .
5.2. Application of the Scheme with the Use of a High-Pressure Heater to Increase the Heat Supply Capacity
The initial parameters (P0 = 0.4 MPa) of turbine units with PWRs provide an efficiency of 30%. Using the above characteristics of SMR-160, the calculated efficiency is equal to Nel/Qreact = 160/525 = 0.3047. This means that there can be no additional heat production. This is necessary, for example, in the KLT-40S plant with a turbine electric power of 35 MW, the reactor power is 150 MW, which exceeds the power required for the turbine 35/0.30 = 117 MW. This makes it possible to generate a relatively large amount of heat for the consumer: 25 Gcal/hour = 29 MW.
If the SMR-160 is used as a nuclear thermal power plant (NTP), then with a thermal capacity of 525 MW, it can successfully provide heat to a large city district in NTP mode. But here the question of economics arises. In the AST configuration, the plant will operate only during the heating season, which makes it uneconomical. To ensure year-round operation, the ASHP scheme must have equipment for both modes: condensing for electricity generation in summer and thermal for heat supply in winter (Figure 1).
In summer, the ASHP operates in condensing mode: the main two-cylinder turbine is used. In winter, it is proposed to switch to the heat recovery mode: the low-pressure cylinder is turned off and all the steam from the exhaust of the high-pressure cylinder is sent to the heat recovery system and to the heat recovery unit (HRU). It is thermodynamically advantageous to heat water in two stages: first with selected steam and then with hot steam.
5.3. Determination of the Efficiency of Using the Backpressure Mode and a Heat Pump from a Small Nuclear Power Plant
To determine the possible thermal power of the heat consumer, a mathematical model of the thermal scheme in the heat recovery mode was calculated (Figure 2) with the following initial data
· reactor (steam generator) power 525 MW;
· parameters of acute steam P0 = 3.4 MPa; t0 = 285˚C;
· feed water temperature 170˚С;
· pressure in the deaerator 1 atm;
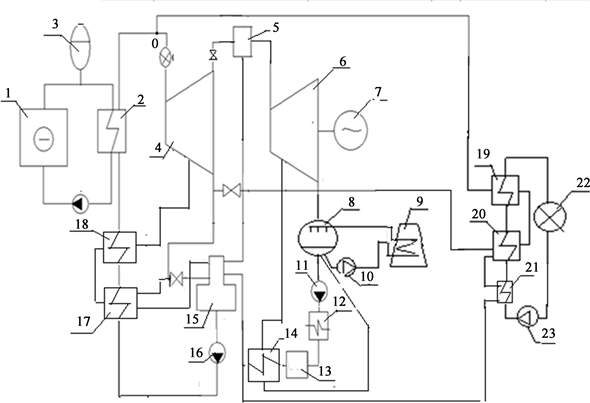
1—reactor; 2—steam generator; 3—pressure compensator; 4—high pressure cylinder of the steam turbine; 5—separator; 6—low pressure cylinder (LPC); 7—electric generator; 8—mixing condenser; 9—dry cooling tower; 10—circulation pump; 11—condensate pump; 12—ejector cooler; 13—desalination plant; 14—low pressure heater (LPH); 15—deaerator; 16—feed pump; 17—high pressure heater (HPH) No. 1; 18—HPH-2; 19—network water heater (NWH-2); 20—NWH-1; 21—drainage cooler; 22—heat consumer; 23—network pum.
Figure 1. Connection scheme for small nuclear power plants to operate in condensing mode in summer and in heating mode in winter.
· direct network water temperature 130˚С;
· return network water temperature 70˚С;
· steam pressure at the centralized steam temperature of 0.357 MPa.
According to the calculation methodology, the heat and power plant was initially calculated at any heat consumer capacity. As a result of the calculation, at a 50 MW TFC capacity, the following were obtained: steam consumption for the network heater D2 = 13.014 kg/s; D7 = 9.44 kg/s; ratio of the hot steam consumption to the steam consumption for PMW-1 D2/D7 = 1.3785
According to the methodology for calculating thermal schemes of turbine units [23] , temperature, pressure, and enthalpy are first determined at the nodal points of the scheme. From the material and energy balance of the steam generator, the steam consumption from GHG and feedwater is determined:
(1)
where Qt—is the heat output of the steam generator; h0, hw—is the enthalpy of acute steam and feed water, respectively.
Then, as a result of calculating the material and energy balance equations for the sequentially of the HRSG-2, HRSG-1, and deaerator, the steam consumption for these elements of the scheme is determined: D3, D4, D6, and D9. In turn:
(2)
where D7 = D9/2.3785 = 83.7 kg/s; D2 = 115.4 kg/s.
The power of the heat consumer will be determined from the proportion: D7 = 9.44 kg/s corresponds to a power of 50 MW; D7 = 83.7 kg/s will provide power X. Hence, the proposed scheme with a reactor power of 525 MW will provide a heat output of 442.5 MW in the heat treatment mode.
Table 1 shows the parameters at all nodal points of the scheme of Figure 2. From these data, we will determine the electric power of the ASMP in the heat treatment mode:
(3)
Let’s estimate the maximum heat supply capacity for SMR-160 SMRs using a heat pump. The calculation of a heat pump for NPP conditions is given in [24] . From these results, it can be concluded that at a condensation temperature of 32.9˚C in the condenser and water heating to 65˚C, COP = 5.365. In SMR-160, the turbine condenser capacity is equal:
(4)
In other words, the evaporator capacity for a heat source is 365 MW. At the same time for a heat pump:
(5)
(6)
![]()
Table 1. Parameters of the working body at the nodal points of the scheme according to Figure 2.
(7)
(8)
Thus, the use of a heat pump with a steam turbine condenser as a low-potential energy source will generate 448.62 MW of heat for the heat consumer and Nturb − Ncomp = 160 − 83.62 = 76.38 MW of electricity.
Table 2 shows the results of calculations of the considered variants of the ASHP thermal scheme, which show that the first variant (condensation mode with heat recovery) loses due to the inability to provide a sufficiently large heat output to the consumer. The next scheme (a) with the low-pressure cylinder (LPC) disconnection and (b) using a heat pump) is almost identical in terms of heat supply capacity. However, the scheme with the use of The scheme using CNTs has twice the electrical power and therefore, at first glance, it may have an advantage.
It should be noted that when using option a, the network water has a temperature of 130˚С, and when using the CNT—65˚С. This difference can be taken into account when calculating the exergy efficiency [25] .
![]()
Figure 2. Scheme of operation of low-capacity nuclear power plants in the heat recovery mode.
![]()
Table 2. Comparison of different schemes of using SMR-160 with SMR-160 in terms of electricity and heat generation.
(9)
where Nel—the electric power; Eq—the thermal power exergy; Qr—the reactor thermal power
From the results shown in Table 2, the exergy efficiency for the variant using HFCs is 1.33% (abs.) higher than the use of heating oil. To increase the heat supply capacity, an improved scheme (Figure 2) with the use of HPW in the backpressure mode and heating of of the network water with hot steam. In this mode of operation, the SMR-160 ASMP with reactor power of 525 MW provides a heat consumer with a capacity of 442.5 MW and an electric power of 36.1 MW. The use of a heat pump with an evaporator which serves as an evaporator of the turbine condenser. This mode of operation allows to produce 448.62 MW of thermal energy for the heat consumer and 76.38 MW of electric power edit text.
6. Discussion of the Results of the Study of Small Nuclear Power Plants for Heat Supply
Implementation of SMR NPPs envisages, in particular, the possibility of their construction near populated urbanized areas, and in this case, the problem of ensuring their nuclear and environmental safety is extremely important.
The proposed scheme for improving the efficiency of heat supply by small nuclear power plants was calculated at a reactor capacity of 525 MW, which will provide 442.5 MW of heat in the heat supply mode. The parameters were calculated at all nodal points of the system (Figure 2) and based on the results obtained, they were determined in the heat supply mode.
For example, let us consider a thermal power plant (TPP) or a nuclear power plant (NPP) as a priority and evaluate the capabilities of NPPs used today in Ukraine. It should be noted that the heat supply to NPP satellite cities is carried out in the heat recovery mode: the network water is heated by condensation of steam taken from the turbine. At the same time, with a turbine electric power of 1000 MW, the thermal capacity of the heat recovery unit of a serial K-1000-5.8/50 turbine is 200 MW. At the same time, the operation of a condensing turbine has restrictions on the minimum steam flow to the condenser. Therefore, it is impossible to extract large amounts of steam from the condensing turbine for heat supply. This means that 1.5 NPP units with VVER-1000 reactors would have to be used to supply only one district of Odesa, which is absolutely unacceptable. In the 1980s, it was planned to build the Odesa NPP, where K-450/500-5.8 turbines were to be installed, which had an increased steam extraction for heat supply. In the combined heat and power mode, they were supposed to produce 450 MW of electricity in winter and 500 MW in summer.
In addition to nuclear power plants, which usually have turbines for electricity generation and a heat recovery unit, it is possible to use nuclear thermal power plants (NTPs) for heat supply, which are designed only for heat production. This allows to reduce the parameters of the coolant and thus increase safety. But in this case, the advantage of combined heat and power generation is lost. In addition, the economic performance of ASTs that will operate only in winter will be relatively worse.
Small modular reactors can be used to build ASHPs, and this will make it possible to generate not only electricity but also heat. This is also related to the global trend of “0 waste”.
One of the biggest advantages of using small nuclear power plants is the ability to reduce the negative impact on the environment.
7. Conclusions
1) As a result of the research, it can be stated that the possibility of using SMR for urban heat supply has power limitations.
2) To increase the heat supply capacity, a scheme with the use of EHWs in the backpressure mode and the heating of network water with hot steam is proposed. In this mode of operation, an SMR-160 NPP with a reactor capacity of 525 MW provides a heat consumer with a capacity of 442.5 MW and an electric power of 36.1 MW. A heat pump is used, the evaporator of which is the turbine condenser. This mode of operation allows generating 448.62 MW of heat for the heat consumer and 76.38 MW of electric power.
3) As a result of comparing the two means of heat supply, it was concluded that the pressure mode is preferable to the backpressure mode, for which the energy efficiency is 1.33% (abs.) higher than the efficiency of the scheme using a heat pump. At the same time, in the backpressure mode, the temperature of the direct network water was 130˚C, while when using the heat pump, the temperature of the direct network water was 65˚C.
4) Replacing coal heat sources at TPPs with low-capacity nuclear power plants can ensure the production of electricity and heat at TPPs without burning fossil hydrocarbon fuels, which will contribute to the process of decarbonization of domestic and global energy to overcome the environmental and energy crisis, and will also become a tool for maintaining climate security.