The Damaging Effects of Abstracting the Deep Aquifers’ Groundwater in Jordan-Quality Constraints ()
1. Introduction and Background Information
Jordan is a part of the Levant, lying east of the northern extension of the Great African Rift Valley, East of the Lower Jordan River, the Dead Sea, and Wadi Araba with a total area of about 89.000 km2 (Figure 1). The prevailing climate is semi-arid and precipitation occurs during the winter months October to April with annual rates of 30 mm/yr. in the south gradually increasing to about 550 mm/yr, in the northwestern highlands of the country. Precipitation decreases rapidly from the eastern mountains of the Jordan Rift Valley westwards towards to the Jordan Valley and eastward towards the eastern borders of the country (DoM, n.d.) . The potential evaporation rates range from 4.000 mm/yr. in the southeastern parts to 1.600 mm/yr. in the high mountains in the northwestern parts of the country. The surface and groundwater resources of the country sum up to around 900 MCM/yr., which indicates water scarcity in a country of more than 10 million people (Salameh et al., 2018; BGR & MWI, 2019; BGR, NRA & MoWI, 1977) .
The Geology of Jordan consists of a series of sedimentary rocks covering a granitic basement cropping out in the southwest of the country and dipping towards the north. The main rocks of the Pre-Cambrian through Lower Cretaceous ages consist of clastics with missing rocks of Devonian, Carboniferous, and Lower Permian (Bandel & Salameh, 2013; Bender, 1968; Burdon, 1959) (Figure 2 and Table 1). In north Jordan, mainly clastic rocks of the Upper Permian, Triassic, Jurassic, and Lower Cretaceous ages cover Silurian and older rocks and form with them one interconnected clastic rocks aquifer complex. Calcareous rocks of the Upper Cretaceous and Tertiary ages build a series of aquifers and badly developed aquifers that overlay in north Jordan the lower aquifer complex.
The groundwater in the country is recharged directly by precipitation along the highlands and, especially along wadi courses in rain poor areas east of the highlands.
The lower aquifer complex receives recharge along its outcrops in the southernmost part of the country and from down percolation of groundwater from the overlying calcareous aquifers in the northern areas (Lloyd & Pin, 1990; Lloyd, 1990; Parker, 1970) . Hence, the groundwater in the lower aquifer complex in the south is fresh, but in areas where it receives down percolating leakages from the calcareous aquifers, or Triassic and Jurassic rocks containing evaporate
![]()
Figure 1. Map of Jordan (Fanack water 2022).
residues, its quality changes, and the groundwater becomes brackish or even saline (Salameh & Hammouri, 2008) .
The groundwater resources, in almost all aquifers and areas in Jordan, are over-drafted resulting in groundwater level declines, mobilization of brackish and saltwater bodies, depletion of all types of springs, and changing flow patterns (Brückner et al., 2021; Salameh & Shteiwi, 2019; Salameh et al., 2018; Salameh, 2008) . The current work will discuss the sources of salinities in the groundwater of the different aquifers and areas to illustrate the effects of extracting the deep aquifer groundwater on the qualities of the fresh groundwater
Table 1. General stratigraphic column of rock formations in Jordan; rock types, ages and thicknesses.
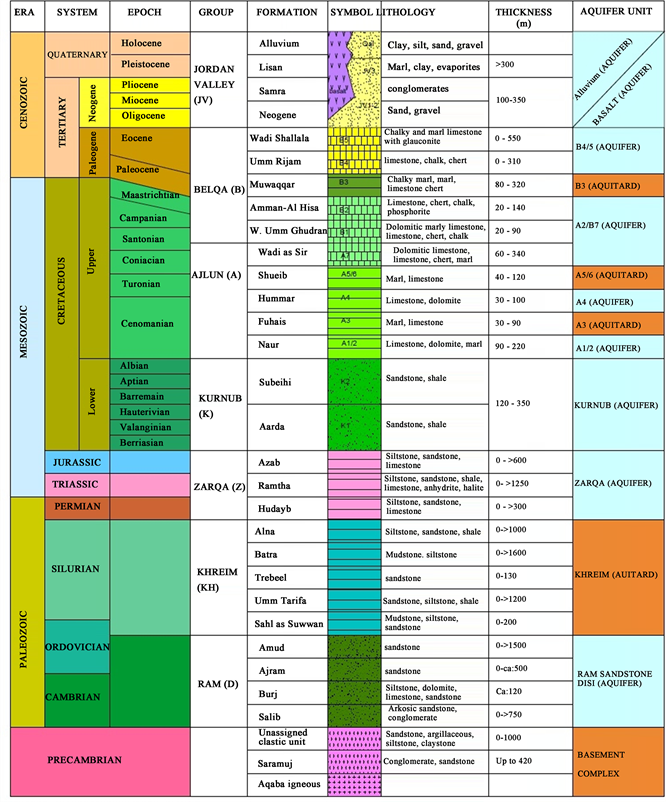
bodies overlying them.
Some of the previous studies about the groundwater of Jordan indicated the dangers these water resources are exposed to. The Ministry of Water and Irrigation monitors groundwater levels and the change in the composition through its expanded network of groundwater observation wells in Jordan and provides that information to researchers through its open files access. In addition, the Ministry publishes annual reports on the trends of groundwater levels and chemistry (MWI, 2016 & 2017) . This information is a major source for any work carried out on the development of the groundwater resources in Jordan. MWI and BGR (BGR & MWI, 2019) have studied in several reports the situation and trends of the groundwater resources in the different basins in Jordan. Salameh (2021) studied the hydrogeological consequences of extracting the deep groundwater in Jordan and concluded that the extraction of the deep saline groundwater will enhance the down percolation of the overlying fresh water into the deep aquifer where that water becomes salinized. Breulmann et al. (2020) studied the vulnerable water resources in Jordan and concluded that the groundwater resources are overexploited and the situation may worsen with time.
This article analyses the sources of salinity in the deep groundwater of the country in order to illustrate the damaging effects of extracting that groundwater on the groundwater stocks, quality, thermal water, and water strategic reserves of the country.
2. Methodology
The required geological and hydrogeological information for the current work was obtained from Salameh, 2021; Bandel & Salameh, 2013; BGR, 1997; Ibrahim, 1993; Bender, 1968; Van Den Boom & Sawwan, 1966; Burdon, 1959 . For this study, the country is subdivided into 3 hydrogeological provinces related to the vertical interconnectedness of aquifer series based on available geological and hydrogeological information (Salameh, 2021; Bandel & Salameh, 2013; Bender, 1968; Burdon, 1959) . The chemical and physical analyses originate from own analyses using US Standard Methods for Water and Waste Water Analyses and German DIN procedures, from the open files of the Water Authority of Jordan, National Petroleum Company, and many Ph.D. and M.Sc. theses supervised by the first author (Salameh). Different programs and software have been used to classify the analytical results, calculate saturation indices and ionic ratios, and establish diagrams such as Piper, Durov, and others.
3. Geology and Hydrogeology of Hydrogeochemical Provinces
3.1. The Deep Sandstone Aquifer System
The deep sandstone aquifer system extends from the granitic basement in the south and southwest in Aqaba and Wadi Araba Mountains where it crops out and dips to the north and northeast to the borders of Jordan with Syria, Iraq, and Saudi Arabia. It can be hydro-geochemically subdivided into 4 provinces, namely (Figure 1).
3.1.1. Province 1: Disi Mudawwara Area
This area of southern Jordan is composed of clastic rocks of Precambrian, Cambrian, Ordovician, and Silurian rocks overlying the granitic basement. They form one interconnected aquifer system (The deep aquifer system) having one groundwater table inclined in a northerly direction (BGR, NRA, & MoWI, 1977; Lloyd, 1990) (Figure 2 and Figure 3, Table 1 and Table 2).
3.1.2. Province 2a: Ras en Naqab to Mujib-Zarqa Ma’in Area
In the area extending from Ras en Naqab to Mujib-Zarqa Ma’in, calcareous rocks of Upper Cretaceous and Tertiary ages overlay the deep clastic aquifer system, but the hydraulic interconnectedness of the clastic and calcareous rocks persists due to semi-aquifer nature of the calcareous and sandy rocks, which are generally composed of sandy limestone, limestone, calcareous sand, dolomite or chalk (Table 3). Only in the eastern Plateau areas thick bituminous marls of most Upper Cretaceous and most Lower Tertiary ages build an extended surficial aquiclude. Chalk marls of the Eocene Rijam Formation, basalts and recent fluviatile overlie the bituminous marls and cover the earth surface to build a local surficial aquifer (Salameh, 2021) .
![]()
Figure 2. Groundwater flow lines with regional water salinities in the deep sandstone aquifer system.
![]()
Figure 3. Piper plot of the groundwater in Disi-Mudawwara area.
3.1.3. Province 2b: Mujib-Zarqa Ma’in to Zarqa River
In the area to the north of Wadi Mujib-Wadi Zarqa Ma’in, Late Permian and Triassic sand- and siltstones intercalate between the deep sandstone aquifer system and the Lower Cretaceous sandstones (Table 4, Figure 4). They increase in thickness in a northeasterly direction, but the rock series from the granitic basement complex up to the Uppermost Cretaceous-Tertiary bituminous marls remains hydraulically interconnected (although in some areas the rocks are hydraulically weekly interconnected), especially because the area is strongly faulted and fractured along E-W and N-S striking weakness zones. The Late Permian-Jurassic rocks contain some evaporate residues, which dissolve in the water reaching them, either from the limited recharge along their restricted outcrops or from the neighboring aquifers.
At a few kilometers to the north of the Dead Sea, Jurassic rocks intercalate into the rock series and increase in thickness northward reaching around 440 m in Zarqa River area (Figure 4). They build, on a scale of about 1 × 1 km, very
![]()
Table 3. Geologic column in the area North of Ras en Naqab to the Latitude of Wadi Mujib (The southern part of Area 2), (Based on NRA 2016 ).
![]()
Table 4. Geologic column of the area of Wadi Mujib Zarqa Ma’in to Zarqa River (The northern part of Area 2), (Based on NRA 2016 ).
![]()
Figure 4. Durov plot of the Groundwater in Disi-Mudawwara area.
limited local aquicludes within the whole rock series, which overlies the granitic basement complex. The Jurassic rocks contain evaporate deposits, which are mainly composed of anhydrite, gypsum, and halite residues giving the groundwater its high salinity. The overlying Cretaceous and Tertiary rocks, in this area, resemble in their composition and set-up those of province 2a: Ras en Naqab to Mujib-Zarqa Ma’in area.
3.1.4. Province 3: Deep Aquifer of the Plateau Area
The deep aquifer here is composed of the rock series overlying the granitic basement from the Precambrian through the Lower Cretaceous ages, generally composed of clastic sediments. This deep aquifer of the Plateau area receives almost no direct recharge. After all, it does not crop out in this area because it is covered, in its southern parts, south of the Mujib River area, by the thick series of Upper Cretaceous and Tertiary calcareous rocks (Figure 5). To the north of Mujib River area, gradually Late Permian, Triassic, and Jurassic rocks intercalate between the Ordovician-Silurian clastics and the Lower Cretaceous sandstones. The thickness of the aquifer reaches up to 2 - 3 km.
3.2. Upper Cretaceous Aquifer/Aquiclude Series of Nodular and Echinoidal Limestone Formations (Na’ur, Fuheis, Hummar and Shueib Formations, A1-6, Figure 2)
This series of rocks is composed of limestone, dolomite, marl, chalk, and shale beds of more than 300 m thickness. The series contains thick beds of fractured and karstified limestone building local aquifers, such as Hummar Formation or parts of Na’ur and Fuheis Formations. The Series as a whole build a poor aquifer, but on a regional scale, it hydraulically connects the overlying aquifers (Massive Limestone and Silicified Limestone Units (A7/B2)) with the underlying deep sandstone aquifer complex (Lower Cretaceous and older clastic rocks). The series contains gypsum layers of a few meters in thickness and other evaporates’ residues (Bender, 1968) .
3.3. Massive Limestone-Silicified Limestone Aquifer System (Wadi Sir and Amman Formations, A7/B2, Figure 2)
This composite aquifer system consists of a series of thick, massive limestone overlain by phosphatic rocks and cherts with a thickness of 200 - 300 m and very high porosity and permeability. It receives direct recharge from precipitation and flood runoff. Its water is generally of low salinity and is a main source of drinking and irrigation water in Jordan.
3.4. Bituminous Marl Aquiclude (Muwaqqar Formation, B3, Figure 2)
The Muwaqqar formation is found in the area of the Plateau extending from Ras en Naqab Escarpment to north Jordan and east of the Highlands to the eastern borders of the country. To the north of the northern latitude of the Dead Sea to around 25 km south of the Yarmouk River the Bituminous Marl Formation has been eroded and is only found in patches. The formation builds an aquitard of up to 300 m in thickness and in Jafr Depression it reached around 800 m (NRA, 2016) . The Formation confines the underlying aquifers and separates them from the overlying surficial composite aquifer of Rijam and Shallala Formations together with basalts and recent clastic sediments.
3.5. Surficial Aquifer System: The Chalk Marl Formations; B4/5 (Rijam and Shallala), the Basalt aquifer, and the Recent Sediments of the Highlands (Figure 2)
The Rijam and Shallala Chalk Marl Formations cover large parts of the highlands of Jordan to the east of the High Mountains. They overlay the thick aquiclude Bituminous Marl Formation. Rijam and Shallala build in Jordan's local surficial aquifers and receive direct recharge from precipitation and flood flows along their outcrops. In some areas such as Jafr, Azraq, and Mafraq these Formations are covered by basalts and/or recent sediments. The groundwater in the composite aquifers is generally fresh, except in playa environments, where evaporation has left behind salty grounds.
4. Hydrochemistry
The Deep Sandstone Aquifer System
· Province 1: Disi Mudawwara to Ras en Naqab area。
· Provinces 2: Southern part: Ras en Naqab to Mujib-Zarqa Ma’in (Province 2a) and Northern part: Mujib-Zarqa Ma’in to Zarqa River (Province 2b)。
· Province 3: The Eastern Plateau of Jordan。
Province 1: Disi-Mudawwara to Ras en Naqab area
The groundwater in this area is found in the clastic sediments, mainly sandstones, originating from the deposition of the weathering products of the nearby granitic basement complex; the northern extension of the Arabian Nubian Shield (Bender, 1968) . These clastic sediments, deposited during Pre Cambrian to Silurian times (Table 2), have, since their emergence from the Tethys Ocean influence, been flushed out by infiltrating precipitation water and therefore, they contain groundwater of very low salinity with EC values of 300 - 450 µS/cm, Table 5. The renewable amounts of the groundwater were estimated by Salameh & Gedeon (1999) to average around 40 MCM/yr. In the Piper diagram (Figure 6), these waters plot in the normal earth alkaline water type with prevailing bicarbonates and sulfates with an increased portion of alkalis. In the Durov diagram (Figure 7), all samples are plotted on the dissolution evaporation line.
All the groundwater in this area is found in the same aquifer complex and
![]()
Table 5. Composition of Disi-Mudawwara groundwater.
extractions from whatever depth have the same effects on the groundwater stock in quantity and quality. The deeper groundwater bodies may be older in their recharge times but they are also fresh.
In the northwestern part of the area, NW of Quweira (Quweira S5 and Jafr; G104 wells) the groundwater differs in its salinity and composition from that of Disi, and it resembles the groundwater of Ras en Naqab area, where the sandstones are covered by Upper Cretaceous calcareous rocks which receive direct recharge from precipitation. The groundwater flow lines (Figure 3) indicate that the groundwater NW of Quweira originates from recharge over Ras en Naqab area. In the Piper diagram groundwater plots in the earth's alkaline water type with increased portions of alkalis and prevailing sulfates, which can be explained by the presence of evaporate residues in the calcareous Upper Cretaceous rocks like the water of the calcareous aquifers in the area E and NE of Ras en Naqab. In Durov the water plots on the dissolution evaporation line.
In the Schöller diagram (Figure 5), Disi water shows ionic rations of Ca > Na ≥ Mg and HCO3 > Cl ≥ SO4, whereas, Quweira and Jafr samples show Na > Ca ≥ Mg and Cl > HCO3 ≥ SO4. In all the analyzed samples, Mg, Cl, Na, and Ca correlate well with the EC values indicating the dissolution of evaporates (Table 6).
The waters are under-saturated for halite, gypsum, and anhydrite and generally slightly under-saturated with aragonite, calcite, and dolomite (Table 7).
Province 2: Southern Part: Ras en Naqab to Mujib-Zarqa Ma’in area (2a)and Northern part: Mujib-Zarqa Ma.in to Zarqa River area (2b)
Province 2a: Ras en Naqab to Mujib-Zarqa Ma’in area
The geologic column in this area allows differentiating 4 aquifer systems
![]()
Figure 5. Schöller plot of the groundwater in Disi-Mudawwara area.
![]()
Table 6. Correlation matrix for the normal water component of Disi-Mudawwara groundwater.
![]()
Table 7. Saturation indices (SI) of the main minerals in Disi-Mudawwara groundwater.
namely, the deep sandstone aquifer system (Precambrian to Silurian directly overlain by Lower Cretaceous sandstones, the lower part of the Upper Cretaceous rocks (poor aquifer (A1-6, Table 3), the upper part of the Upper Cretaceous most lower Tertiary rocks (Excellent Aquifer) and the surficial Middle Tertiary aquifers together with basalts and quaternary sediments (B2/A7, B4, 5, Table 3).
From the area of Ras en Naqab northward and eastward the deep sandstone aquifer system receives negligible amounts of direct recharge, which are limited to its outcrops along the eastern escarpment of the Jordan Rift Valley. Its water originates mainly from recharge in the area south of Ras en Naqab Escarpment and from down percolation of water from the overlying calcareous Upper Cretaceous sequence of aquifers and semi-aquifers.
From Ras en Naqab to the Mujib-Zarqa Ma’in area the increase in salinity is a result of the increases in the downward percolated amounts of groundwater from the overlying calcareous Upper Cretaceous rocks containing evaporate residues into the deep sandstone groundwater system (Table 8).
The deep groundwater salinity increases accordingly from the Disi Area northward from 300 - 400 µS/cm in Disi to about; 550 in Wadi El-Hasa, 600 in Weda’a (Tributary of Wadi Karak), 850 µS/cm in Wadi Ibn Hammad, and 1400 µS/cm in Wadi Mujib area.
Province 2b: Mujib-Zarqa Ma.in to Zarqa River area
The gradual intercalation of Late Permian to Jurassic rocks between Mujib latitude and Zarqa River latitude increases the salinity of the deep sandstone aquifer system from about 3000 µS/cm in Wadi Zarqa Ma’in area to more than 6000 µS/cm east of the northeastern edge of the Dead Sea and to more than 10.000 µS/cm in Zarqa River area (Salameh & Tarawneh, 2017; Tarawneh, 2015) , (Figure 9, Table 8).
Direct recharge into the aquifer system here (Precambrian through Lower Cretaceous) is very limited due to the limited surface exposure of these rock formations and the groundwater originates from downward and lateral flows of groundwater from the overlying and neighboring aquifers, which dissolve evaporates, and lead to additional increases in the groundwater salinity of the deep aquifer system.
Springs and wells in this area discharge a mixture of groundwater chemistries affected by the composition of the late Permian, Triassic, Jurassic, and Lower Cretaceous aquifers. The water chemistry results from the original quality of the deep sandstone aquifer composition (Disi area), added to it contributions from the Triassic and Jurassic evaporates and down percolations of groundwater from
![]()
Table 8. Composition of the groundwater along its flow direction from Disi towards the Dead Sea and the Jordan Valley area in the north.
the calcareous Upper Cretaceous aquifer series also containing evaporates (Salameh, 2022) .
The groundwater salinity increases along the groundwater flow path reflecting increases in the concentrations of Na, Ca, Mg, Cl, SO4, and to a lesser degree K (Table 8). Two sources of water salinity contribute to the composition of the deep sandstone aquifer system extending from Disi to Zarqa River and these are the down-percolating water from the calcareous lower [arts of the Upper Cretaceous rocks containing evaporates in the whole area and the area from Mujib latitude to Zarqa River, in addition, dissolution of evaporate layers and residues intercalated within Late Permian, Triassic and Jurassic rock series (Table 4). All these deep groundwater sources contain, in addition, radioactive substances as can be shown in Table 9 on the example of radon gas analyses, which may further restrict the water uses, especially for drinking purposes because they require additional expensive treatment.
In the Piper diagram (Figure 6), the samples collected along the groundwater flow lines start as earth-alkaline water with increased portions of alkalis and prevailing carbonates or sulfates, gradually developing to alkaline and earth-alkaline with prevailing chlorides and sulfates, resulting from the dissolution of mainly gypsum and halides.
In the Durov diagram (Figure 7), the water plots on the dissolution-evaporation line with limited ion exchange and reverse exchange.
In the Scholler diagram (Figure 8), the water shows Na ≥ Ca > Mg and Cl > SO4 < > HCO3.
The water in the recharge area is undersaturated with calcite, dolomite, and aragonite and along the flow path, it generally becomes saturated to oversaturated with these minerals (Table 10). Along the same flow line, the undersaturation with halite, gypsum, and anhydrite decreases strongly as a sign of additional dissolution of evaporates.
The correlation (Table 11) of the different ions shows a good correlation between Na and Cl, Ca, and K and to a lesser degree with SO4.
In summary, in this area; Province 2, Disi to Zarqa River, the fresh, low salinity groundwater receives, along its flow path, down percolation water from
![]()
Table 9. Radon concentration in the different deep aquifer sources.
![]()
Figure 6. The Piper diagram showing the evolution of the groundwater type along the flow lines from Disi area through Zarqa Ma’in and to Zarqa River latitudes, evolving from earth alkaline water with increased portions of alkalis and prevailing carbonates or sulfates to alkaline and earth alkaline with prevailing chlorides and sulfates.
![]()
Figure 7. The Durov diagram showing that the water plots on the dissolution-evaporation line with limited ion exchange and ion reverse exchange and the evolution from carbonate to chloride and sulfate water.
![]()
Figure 8. The Schöller plot of the groundwater in the area Disi to Zarqa River.
![]()
Table 10. Saturation indices of the groundwater along the groundwater flow path from Disi to Zarqa River area.
![]()
Table 11. Correlation matrix for the normal water component of Disi to Zarqa Ricer groundwater.
the calcareous Upper Cretaceous aquifers containing evaporates, which leads to its increasing salinity and change in type from mainly carbonate to chloride and sulfate water. To the north of Mujib latitude evaporate-bearing intercalation of Late Permian, Triassic, and Jurassic rocks contribute with additional strong increases in the salinity to the deep groundwater as a result of water-rock matrix interactions and the water becomes of alkaline type with prevailing chlorides and sulfates.
Province 3: The Eastern Plateau of Jordan
In the eastern plateau of Jordan, the deep groundwater was encountered in the deep drilled water, as well as, in oil wells, where only brackish and/or saline water was found (GITEC, 1995; NRA, n.d.; National Petroleum Company, n.d.) .
Since the deep aquifer is horizontally and vertically interconnected via its primary, as well as, its secondary permeability, the chemical composition of its water changes gradually in both these directions. Therefore, the samples collected from the different formations and depths give good information about the general composition of the groundwater in the whole deep aquifer in this area.
As detailed above, the deep clastic aquifer dips in a northerly direction and becomes covered by a thick sequence of Upper Cretaceous and younger rocks, where it can be encountered at depths of more than 1000 mbgs. Therefore, the calcareous lower part of the Upper Cretaceous rocks; Na’ur, Fuheis, Hummar, and Shueib Formations (A1-6) functions here, in practical terms, as a deep aquifer.
The groundwater in the deep aquifers has salinities ranging from a few thousand µS/cm up to a few ten thousand µS/cm and the groundwater becomes rich on in sulfates and chlorides indicating dissolution of evaporates, ion exchange, and reverse ion exchange as observed in some of the deep groundwater bodies and as is detailed in the following:
Treibel area deep groundwater
The drilled wells in this area encountered a mixture of groundwater present in the different deep clastic aquifers. The salinity of the groundwater ranges from 4.480 to around 13.700 µS/cm Table 12. It plots in the Piper diagram (Figure 9) as earth alkaline type with prevailing sulfates and in the Durov diagram in the reverse ion exchange area, where Na dissolved in the water has replaced Ca and Mg in the rock matrix (Figure 10). The ionic ratios are Ca > Na > Mg and SO4 > Cl ≥ HCO3 (Figure 11).
The water is slightly oversaturated with calcite, dolomite, and aragonite, medium undersaturated with gypsum and anhydrite, and undersaturated with halite (Table 13). All EC, Ca, Mg, Na, K, SO4 and Cl correlate with each othe to more than 99% (Table 14).
![]()
Figure 9. Piper diagram showing Treibil water as of earth alkaline type with prevailing sulfates.
![]()
Table 12. Composition of the groundwater of the deep aquifer in the Treibil area (meq/L).
![]()
Figure 10. Durov plot of Treibel water showing reverse ion exchange processes.
![]()
Figure 11. Schöller plot of the groundwater in Treibil area.
All that indicates that the composition of the groundwater in this area is a result of water-rock interaction, involving the dissolution of evaporates, especially gypsum and some carbonates, and reverse ion exchange, where Na and K in the water were exchanged against Ca in the rock matrix.
Deep aquifer mixed water (Plateau area)
In both Hammad and Sirhan areas, deep wells encountered mixtures of the deep clastic aquifers’ water with a wide range of salinity 4.480 - 13.660 µS/cm (Table 15). These waters are of alkaline to earth alkaline type with prevailing chlorides and sulfated (Figure 12). In the Durov diagram the water plots near the dissolution/evaporation line with slight ion or reverse ion exchange (Figure 13). Their ionic ratios are: Na > Ca >> Mg and Cl > SO4 >> HCO3 (Figure 14).
![]()
Table 13. Saturation indices of the groundwater in Treibil deep wells.
![]()
Table 14. Correlation matrix of Treibil water parameters.
![]()
Table 15. Composition of the mixes groundwater of the Plateau area.
The water is under to oversaturated with calcite, dolomite, aragonite, anhydrite, and gypsum and moderately undersaturated with halite (Table 16). Na and K correlate well with Cl, but the other ions do not show any degree of correlation (Table 17), reflecting the mixed type of water in this area. The salinity of the groundwater is a result of carbonates and evaporates dissolution from the different aquifers’ rock matrices.
Deep aquifer in Hammad area; Ajlun Formations (lower part of the Upper Cretaceous)
As mentioned above, the calcareous lower part of the Upper Cretaceous rocks
![]()
Figure 12. Piper diagram for the mixed deep groundwater.
![]()
Figure 13. Durov diagram for the mixed deep groundwater.
was built in this area of the deep aquifer. Its water is a mixture of waters originating from a small component of direct recharge and a major component of inter-aquifer flows. The salinity ranges from around 3.000 to 4.000 µS/cm (Table 18). The water is of earth alkaline type with prevailing sulfates (Figure 15). In the Durov diagram the water plots on the dissolution/evaporation line (Figure 16), and shows no major ion exchange reactions. The ionic rations are Na =
![]()
Figure 14. Schöller plot for the mixed deep groundwater of the Plateau area.
![]()
Table 16. Saturation indices of the mixed groundwater of the Plateau area.
![]()
Table 17. Correlation matrix of the Plateau area water parameters.
![]()
Table 18. Composition of Ajloun deep groundwater in the Plateau area.
![]()
Figure 15. Piper diagram for Ajloun deep groundwater in the Plateau area.
![]()
Figure 16. Durov diagram for Ajloun deep groundwater in the Plateau area.
![]()
Figure 17. Schöller diagram for Ajloun deep groundwater in the Plateau area.
![]()
Table 19. Saturation indices of Ajloun deep groundwater in the Plateau area.
![]()
Table 20. Correlation matrix of Ajloun deep groundwater in the Plateau area.
Ca > Mg and SO4 < > Cl > HCO3 (Scoller Diagram, Figure 17). The water is saturated with calcite, dolomite, and aragonite and slightly undersaturated with gypsum and anhydrite (Table 19). Na correlates very well with Cl and NO3, Mg with SO4 and Ca with SO4, K, HCO3 and NO3 (Table 20).
5. Discussion
Based on the above elaborations on the hydrogeological, and hydrochemical situation of the deep aquifer system composed of clastic rocks of Precambrian through Lower Cretaceous with total missing Devonian, Carboniferous, and Early Permian rock formations and partial missing Late Permian, Triassic, and Jurassic, the whole rock sequence is found to be hydraulically, horizontally and vertically interconnected. This deep aquifer receives direct recharge from precipitation water along its outcrops in the Disi Mudawwara area in southern Jordan and some recharge along its limited outcrops along the eastern highlands of southern Wadi Araba. The main water source of this deep aquifer in the area north of Disi originates from down percolations of groundwater from the overlying aquifers composed of calcareous Upper Cretaceous and Tertiary rocks containing evaporates in the area extending from Ras en Naqab to Mujib area. To the north of Mujib latitude, Late Permian and Triassic fine clastic rocks intercalate between the Lower Cretaceous sandstone and the Silurian-Ordovician clastic rocks, and to the north of the Dead Sea latitude Jurassic rocks containing thick gypsum layers and other evaporates intercalate into the rock column. In this area, the major quantity of groundwater reaches the deep aquifer as a result of down percolation from the overlying aquifers, which contain high salt contents rendering the original deep aquifer water brackish to salty. The interconnectedness of the whole rock series overlying the granitic basement is not only provided by its rocks’ primary porosity but also due to its expansion tectonics dissecting the country, by an extensive network of normal and vertical faults into geologic blocks (Bender, 1968) of generally less than 20 × 20 km in extension. Thus, hydrogeological, guarantees the interflow of groundwater between aquifers horizontally and vertically.
In the northeastern parts of the country, the clastic deep aquifer lies at depths of more than 1000m bgs and the calcareous lower part of the Upper Cretaceous rocks (A1-6 Formations) are considered the deep aquifer there. Its water originates from precipitation over its outcrops in the highlands and from down percolation from the overlying calcareous upper part of the Upper Cretaceous rocks (B1, 2/A7 Formations). Evaporates in the A1-6 series are the main source of water salinity in this group.
Based on the geologic hydrogeologic and hadrochemical data discussed above, any extraction from the deep brackish and saline groundwater will be compensated by increasing downward and later flows from overlying and neighboring aquifers. Therefore, extracting the groundwater from the deep aquifer or its overlying aquifers will be, hydrogeological, the same with the following disadvantages and damaging effects of groundwater extractions from the deep aquifers:
· Enhancement of freshwater movement into brackish and saltwater regimes to substitute the extracted water.
· Salinization of the freshwater which substitutes the brackish and salty water extractions from the latter.
· Declining discharges from the deep aquifer springs including the thermal mineralized springs used for therapeutic purposes.
· Declining deep aquifers’ water seepages along the eastern slopes of the Jordan Rift Valley used in irrigation.
· In some areas, extractions from deep groundwater have led to enhanced seismicity due to pressure release within aquifers such as what happened in Wadi Arab, Yarmouk, and Lake Tiberias area (Shalev et al., 2023) .
· Generally, the deep groundwater contains high radioactivity, which makes additional treatment for use, especially for drinking purposes necessary.
6. Conclusion
In the end, it can be concluded that the extraction of the deep groundwater is another way around overexploiting the shallow aquifer. It is a vicious circle of drilling deep wells, extracting the brackish and salty water allowing the fresh water to substitute the extracted deep water, and desalinating the extracted deep water to become useable, with all the economic and environmental impacts of such projects like: How and where should the desalination brine be disposed?
Therefore, from a hydrogeological, hadrochemical, economic, and environmental point of view, Jordan should refrain from extracting the brackish and salty deep groundwater resources overlain by fresh groundwater aquifers due to the damaging percussions of such involvement.
Acknowledgements
The authors acknowledge the Ministry of Water and Irrigation in Jordan for providing the data.