Hydrochemical Characterisation and Assessment of the Level of Contamination of Groundwater Collected by Private Waterworks in the Town of Moundou in the South of Chad ()
1. Introduction
Water quality around the world has deteriorated significantly in recent years, due to uncontrolled industrial discharges, the intensive use of chemical fertilisers in agriculture and the irrational exploitation of water resources. These have produced a chemical change in the water, making it unsuitable for the desired uses (Belksier et al., 2016) . Groundwater quality is influenced by many factors, such as the chemistry of the infiltrating water, the geological nature of the reservoir rock and anthropogenic factors (Frape et al., 1984) .
In Africa, demographic growth accompanied by rapid urbanisation is causing numerous disturbances to natural environments (Ghazali & Zaid, 2013) . The urban environment concentrates populations and activities in a small area, which has to support numerous flows (Host & Telle-Lamberton, 2014) . Much of this growth takes place in or around already overcrowded slums, peri-urban areas and informal settlements. Rural migrants from extreme poverty are moving into residential areas lacking basic water and sanitation infrastructure (PNUD, 2006) . This is the case in most developing countries, where the major problems that local authorities in large African cities constantly have to face are those linked to the sustainable management of urban waste and access to an adequate sanitation system (Soro et al., 2010) . The concentration of the population in urban areas only exacerbates the problem of access to water (Boubakar Hassane, 2010) . Furthermore, industrialisation, the non-rational use of fertilisers and pesticides, and the lack of public awareness of the need to protect the environment all lead to an imbalance in the ecosystem and generate pollutants that can affect the physico-chemical and biological quality of water (Mulliss et al., 1997) . Such pollution is not without risks for the local population, with persistent exposure to pollutants and possible migration to surface or groundwater, leading to a deterioration in drinking water resources (Host & Telle-Lamberton, 2014) . The problem affects more vulnerable and deprived populations in disadvantaged neighbourhoods (Sy et al., 2011) . With the inadequacy of the conventional water network in African environments, these populations resort to alternative water sources of dubious quality for domestic needs (Temgoua, 2011) . Untreated water can contain a multitude of parasites and bacteria that cause serious illness in humans.
In Niamey in Niger (Hassane et al., 2016) , Ouagadougou in Burkina-Faso (Yameogo-Ouandaogo, 2008) , N’Djaména in Chad (Kadjangaba et al., 2018) and Dakar in Senegal (Ndiaye et al., 2010) , groundwater contamination is linked to the fact that wastewater produced at the surface of the ground infiltrates through the unsaturated zone and reaches the shallow water table without having been able to benefit from effective filtration.
The town of Moundou is located in the southern part of Chad, with specific hydro-climatic characteristics. This, in “a context of climate change where cities face frequent droughts and floods” (Bedoum et al., 2014) . Moundou is one of the cities in Africa south of the Sahara with strong demographic growth, “this acceleration of the population is followed by a dizzying spatial expansion and widespread poverty in and around it” (Djangrang et al., 2011) . As a result, “in most cases, the population occupies flood-prone areas and is vulnerable to heavy rains” (Tchotsoua, 2007) . Faced with urban growth, the commune is finding it difficult to control the pace of spatial development in the new districts, and water, which is a factor in development and spatial organisation, is becoming a worrying problem for the town’s population.
Moundou has a sedentary population of 137,251, representing 1.3% of the Chadian population (INSEED, 2009) . Water is supplied by public standpipes, individual connections from the Société Tchadienne des Eaux (STE), which is responsible for water distribution in the town, traditional boreholes and private wells, private water vendors and surface water (the Logone River and Lake Wey). There are many private boreholes and wells, compared with the public standpipes and individual connections provided by the Chadian Water Company (STE). Wells (modern or traditional) are now the most common method of water supply in the town (32%). Water from boreholes and private wells is sometimes used for household purposes, and water from standpipes for drinking water. In the absence of standpipes and the STE network, this water is the only source of water for households and for drinking (STUDI, 2010) . The water table is fed directly by rainfall, but rivers also contribute to groundwater recharge during periods of high water, while draining the water table during the rest of the year. During the rainy season, the water table rises to the surface of the ground, where it mixes with rainwater run-off (Schneider & Wolff, 1992) . However, it should be emphasised that the current state of knowledge of the water table in the town of Moundou only allows us to sketch the broad outlines of how the aquifer functions (in particular its recharge by rainfall) and to approach only its main organoleptic characteristics. In fact, there is virtually no hydrodynamic or hydrochemical monitoring of the aquifer, as the available data comes from the few studies carried out for the boreholes (Bandé, 2016) .
2. Study Area
The town of Moundou, which is the subject of this study and the capital of the province of Logone Occidental, is located in the south of Chad in the Sudanian zone. It can be located between latitudes 8˚33' and 8˚36' North and longitudes 16˚06' and 16˚06' East. Its relief is marked between altitudes of 382 m and 439 m. It is built in the middle of three bodies of water, namely the Logone River to the south, Lake Wey to the west and Lake Taba to the east, but also on a basin The town of Moundou is second only to N’Djamena in terms of population, so it is home to a number of industrial production units and enjoys the status of Chad’s economic capital” (Djangrang et al., 2011) . It is the capital of the Lac Wey department and the capital of the Logone Occidental region. It has four (4) arrondissements with twenty-seven (27) districts, themselves subdivided into 251 squares. It is undergoing rapid spatial expansion towards the north-west, with accelerated urbanisation like other African towns, due to its economic dynamism and its openness to Cameroon via the Touboro road. It faces many problems, including repeated flooding” (Mbaihadjim, 2016) . It had a population of 137,251 sedentary inhabitants, including 26,186 ordinary households with an average of 4.9 people, spread over an area of 3.6 ha (INSEED, 2009) . Its surface area was 2200 ha in 2010 (Studi, 2010) . The town of Moundou is located in the Koros region. The Koros region forms the southern part of Chad, stretching between the crystalline massifs corresponding to the border with the Central African Republic and the Chari flood plains. The region takes the form of a plateau divided into 8 plateaux, hence the name “KOROS”, by valleys. The altitude of the relief can exceed +450 and +500 m, with a summit at +627 m (Koro de Bebangher, Schneider & Wolff, 1992 ). The plateaux are deeply incised by the hydrographic network, and the very wide valleys (Logone Oriental and Occidental, Chari, Ouham, Tandjilé, Pendé, Mandoul, etc.) cut into the Continental Terminal terrain by several tens of metres (Pias, 1970) . The town of Moundou, the subject of this study, is located in the hilly part of the Koros region, at an altitude of +398 m.
In the Koros region, the bedrock is composed of crystallophyll and igneous rocks of Precambrian age. It is the site of discontinuous aquifers. It is found at variable depths of between 200 and 600 m for most of the region, and at great depth in the trenches. The overburden formations are essentially made up of Terminal Continental (TC) and Intercalary Continental (Schneider, 1968, 1970; Mathieu, 1980) . Groundwater is free, shallow groundwater that is accessible to wells. The water table is fed by rainfall infiltration through permeable soils. The Koros region has a general water table that is mainly found in the Continental Terminal (CT) formations. The Terminal Continental formations can be more than 500 m thick and are made up of sand and sandstone with criss-crossing stratifications, multi-coloured clays and lenses of kaolin. This water table is drained by rivers in the valley bottoms. As a result, the surface area of the water table is minimal (less than 10 m) in the alluvial plains and increases as you move away from the valleys towards the summits of the Koros. The wells can then be over 60 and 80 m deep. The highly varied productivity of wells dug in the Continental Terminal formations demonstrates their great lithological heterogeneity. Groundwater is low or very low in mineral content (dry residue less than 200 and very often less than 100 mg/l). The water has a calcic to calcosodic bicarbonate facies and is markedly acidic and corrosive (Schneider, 1968; Schneider & Wolff, 1992) . In the town of Moundou, during the wet season (rainy season), the water table is fed directly from the rainfall, its level rises to ground level and its water then mixes with the run-off. The Logone and the Taba and Wey lakes also contribute to the recharge of this surface water table during periods of high water, while draining the water table during the rest of the year. In fact, this water table, which is exploited by private wells, is very heavily polluted by infiltration from cesspools, latrines and domestic wastewater (SEURECA, 2014) .
3. Material and Methods
3.1. Materiel
The equipment used for the research consists of computer tools for data processing and fieldwork tools. These are: RStudio for statistical analysis; Grapher 15 and Excel for plotting graphs; Diagramme software to determine the chemical facies of the groundwater in the study area; Qgis, Surfer and Arcgis to produce the maps.
A GPS for taking coordinates; 1.5 litre bottles for sampling well water, boreholes and surface water; a luminous piezometric probe with an amplitude of fifty (50) metres for measuring water levels in wells and boreholes; a WTW multi-parameter field meter to measure temperature, conductivity and hydrogen potential.
3.2. Methods
Water samples for laboratory analysis were taken from wells, boreholes and surface waters (Lake Taba, Lake Wey and the Logone River) (Figure 1) during the high-water period (August). These samples were taken in 1.5 L bottles for physico-chemical analysis (major ions (Cl−,
,
,
, Ca2+, Mg2+, Na+, K+), SS and dissolved oxygen, etc.) of groundwater and surface water were measured in situ using a pH meter and a WTW 330i multi-parameter field meter.
![]()
Figure 1. Presentation of the Moundou town with sampling collection in red.
3.3. Analysis
A total of 64 groundwater samples (wells and boreholes) and 5 surface water samples (Lake Taba, Lake Wey and the Logone River) were analysed in the laboratory. Major elements such as Chloride (Cl−), Sulphate (
), Nitrate (
), Calcium (Ca2+), Magnesium (Mg2+), Sodium (Na+) and Potassium (K+) were analysed by High Performance Liquid Chromatography (HPLC) at the Laboratoire National des Eaux (LNE) in N’djaména, Tchad. The bicarbonate ion (
) was determined using the titrimetric method.
These results were tested for ionic charge balance using the following formula (Freeze & Cherry, 1979) :
Analytical precision was ±5%. Results are expressed in mg/l. The parameters measured at the various sites are set out in Table 1.
![]()
Table 1. Results of physico-chemical analysis of groundwater and surface water in the town of Moundou.
3.4. Data Processing
The data collected on groundwater in the town of Moundou was processed using hydrochemical and multivariate statistical methods. For the hydrochemical study, the Piper diagram was used to classify the water. Indeed, the use of this diagram in the field of hydrochemistry by many authors (Biémi, 1992; Yaméogo, 2008; Abdou Babaye, 2012; Sambiénou et al., 2018) has led to very good results. The statistical approach used to study the phenomena at the origin of water mineralisation, the grouping of waters and to identify the factors responsible for these groupings is based on Principal Component Analysis (PCA) and Ascending Hierarchical Classification (AHC). Their application to the hydrochemical study of water has been carried out by several authors in Africa and around the world, with very significant results (Biémi, 1992; Yaméogo, 2008; Sambiénou et al., 2018) .
The applicability of the exploratory PCA technique is based on the choice of the number of axes representing the maximum retained inertia with the minimum possible number of factors. In general, the PCA of a region is only valid when the factorial designs studied retain at least 70% of the information (Faillat & Drogue, 1993; Abdou Babaye, 2012; Sambiénou, 2019) . Below this limit, it is considered that the study of the region did not take into account a large amount of information. Statistical analyses were performed on the following 18 variables: pH, temperature, TDS, electrical conductivity, Na+, K+, Mg2+, Ca2+, Cl−, F−, Br−,
,
,
,
,
,
and Salinity.
Statistical analyses were carried out using Rstudio software. The eigenvalues, the factorial weights of the variables and the factorial weights of the individuals resulting from this treatment are reported in the tables below.
4. Results and Discussion
4.1. Physico-Chemical Parameters of the Water
The water temperature varies between 26.2˚C and 33˚C, with an average of 29.35˚C. Overall, these temperatures are fairly close to the air temperature, which is 33˚C (ANAM, 2022) , indicating a thermal equilibrium between the aquifer and the atmosphere. The electrical conductivity of the groundwater varies between 7.29 μS/cm and 3670 μS/cm, with an average of 487.44 μS/cm. These values indicate high mineralisation in some wells and low to medium mineralisation in others, as some electrical conductivity values are higher than 1000 µS/cm, exceeding WHO standards, 2000 (i.e. 1500 µS/cm) and others are lower than 1000 µS/cm and do not reach WHO standards, 2000 (i.e. 1500 mg/l). The electrical conductivity of surface water obtained during field measurements ranged from 50.8 μS/cm to 77.5 μS/cm, with an average of 63.42 μS/cm. These values show low mineralisation compared with the WHO standard (2000) (i.e. 1500 mg/l).
Mineralization corresponds to the total dissolved salts contained in the water.
The TDS values of the water studied vary widely in the field, from 3.63 mg/l to 1840 mg/l, with an average of 241.08 mg/l. In the case of surface water, values ranged from 25.3 mg/l to 38.9 mg/l, with an average of 31.74 mg/l. These values show that the TDS of surface water is low and remains below 40 mg/l.
The pH of groundwater in the town of Moundou range from 3.26 to 6.41, with an average of 5.20.
With an average of 5.13, the pH of surface water is between 5.27 and 6.42 with an average of 6.08. These groundwater and surface waters are therefore acidic. The cations are, in descending order of weight: sodium, calcium, magnesium and potassium. Sodium is the most abundant cation in the groundwater and surface water of the town of Moundou, varying from 0.4 to 89 mg/l, with an average of 15.88 mg/l. All values are below the WHO standard (150 mg/l). Calcium levels range from a minimum of 1.4 mg/l to a maximum of 80 mg/l, with an average of 12.53 mg/l, below the WHO standard (250 mg/l). Magnesium, with an average concentration of 2.82 mg/l, for a minimum of 0.1 mg/l and a maximum of 24.3 mg/l, is the cation for which all the values obtained are below the WHO standard (i.e. 50 mg/l). Potassium concentrations varied from 0.1 mg/l to 14 mg/l, with an average value of 1.59 mg/l, with 1% of water points recording levels above the WHO standard (i.e. 12 mg/l).
Anions are listed in descending order of weight as follows: bicarbonate, chloride, nitrate and sulphate. Bicarbonate is the dominant anion, with levels ranging from 3.66 mg/l to 366 mg/l, with an average of 52.30 mg/l. For chloride, the average is 13.93 mg/l, with a minimum of 1 mg/l and a maximum of 100 mg/l. All values are below the WHO standard (i.e. 250 mg/l). Nitrate levels ranged from 2 mg/l to 88 mg/l, with an average of 12.19 mg/l. Some nitrate concentrations are very high in most water points. In fact, these levels exceed 50 mg/l (World Health Organization, 2004) . Sulphate ion levels are low, ranging from 0 mg/l to 36 mg/l, with an average of 4.45 mg/l. The values are all below the WHO standard (i.e. 250 mg/l). Examining the major element content shows that the mineralisation of the water is controlled by calcium, magnesium and sodium as cations and by chloride and bicarbonate as anions.
Figure 2 shows the Piper diagram for all the waters studied. Plotting the major ions on the Piper diagram (Figure 2) reveals four main groundwater facies in the town of Moundou: the chloride and sulphate facies, the calcium and magnesium facies, the sodium and potassium facies and the bicarbonate facies.
The values of the chemical parameters of the analysed samples, plotted on the Schoeller-Berkaloff diagram, show that all the waters analysed have maxima at the HCO3 + CO3 and Cl poles for anions, indicating that all the waters are bicarbonated and chlorinated. For the cations, most of the waters have maxima at the Ca and Na + K poles. This shows that the analysed waters have four (04) facies: the chloride and sulphate facies, the calcium and magnesium facies, the sodium and potassium facies and the bicarbonate facies (Figures 3(a)-(d)).
![]()
Figure 2. Piper diagram of well and borehole water.
4.2. Pearson Correlation Matrix
The relationship existing between all the variables taken in pairs and the correlation coefficients between these different variables are given by the correlation matrix (Table 2). This table, which presents the different correlations between the variables, can help to understand the different processes involved in the mineralisation of the waters studied. The correlation analysis shows a strong positive correlation between all the major ions taken in pairs. This observation suggests that these ions come from the same source, which would be mineralisation. Nitrates are strongly and positively correlated with sodium, potassium, calcium and magnesium. This link between nitrate and sodium, potassium, calcium and magnesium ions suggests an additional contribution of these ions to the water table from surface water infiltration. Chlorides, iron and ammonium showed no significant correlation with the other parameters studied.
4.3. Principal Component Analysis
Principal Component Analysis (PCA) is a multivariate statistical method that extracts correlations from a set of data through a set of orthogonal empirical functions. It is a descriptive method for studying linear relationships between variables without taking into account any a priori structure (Jolliffe, 2002) . In order to identify the most important processes controlling groundwater geochemistry, we used two multivariate statistical methods, namely Principal Component Analysis (PCA) and Hierarchical Ascending Classification (HAC). These statistical techniques establish relationships between the various physico-chemical parameters studied and between individuals (water points studied). This makes it possible to identify groups of parameters that may have a similar type of variation (Mudry, 1991) . The use of multivariate statistical analyses to study the
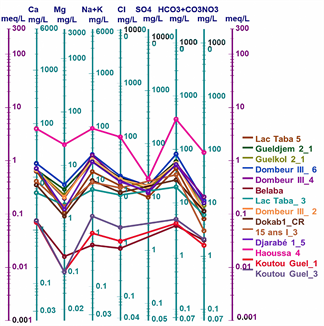
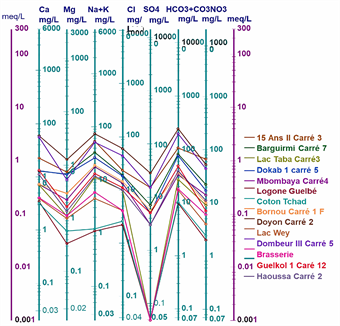
(a) (b)
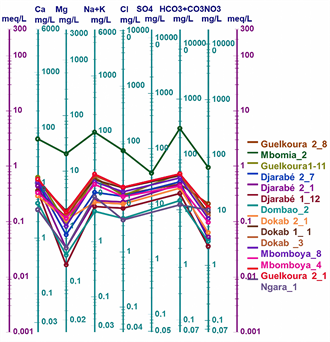
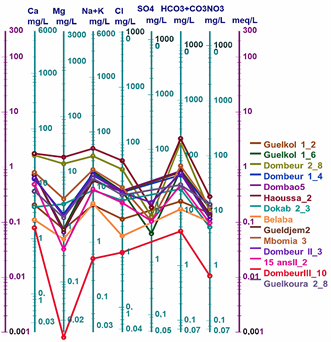
(c) (d)
Figure 6: Schöeller-Berkaloff diagram of well, borehole and surface water in the town of Moundou.
![]()
Table 2. Correlation matrix of variables.
chemical quality of groundwater enables us to differentiate their chemical characteristics, determine their overall variations (factors) along the main axes and, above all, characterise the different centres of mineralisation acquisition. The advantage of these techniques lies in the fact that they allow most of the variance expressed by the descriptors to be reduced to a few factorial planes. The first Dim.1 axis expresses the highest percentage of the total variance. The second axis, F2, which is independent of the first, is the one that expresses most of the residual variance, and so on (Blavoux & Mudry, 1986) . The chemical descriptors taken into account in this exercise are: EC, T˚C, turbidity, Na+, K+, Ca2+, Mg2+, Cl−,
,
, Fe2+,
,
. With regard to the number of principal components to be retained, we chose the eigenvalue analysis method (Table 3); thus the factorial axes with eigenvalues of less than 1 were eliminated, making it possible to retain the first four principal components, which explain almost 80% of the total variability.
The first component (Dim.1), explaining 52.8% of the total variance, shows positive correlations for the variables Na+, Ca2+, Mg2+, Cl−,
,
,
(Figure 4, Table 3). This strong correlation characterises the overall mineralisation of the groundwater. The presence of nitrate ions in this group is an indicator of anthropogenic pollution. As the study area is not close to the ocean, the presence of chloride ions would come from evaporites. This axis describes both the groundwater mineralisation process and the impact of anthropogenic activities on this resource through agricultural chemical fertilisers, solid and liquid waste and the decomposition of organic matter. A number of authors, including Sarazin et al. (1976) , Bourrié (1978) , Lelong et al. (1989) and Faillat & Drogue (1993) , have shown that all the strong acid anions (Cl−,
,
) and more than half of the organic matter in groundwater are present in the soil. Cations (Ca2+, Mg2+, Na+) could be contributed by precipitation in some cases.
The second component (Dim.2), explaining 10.34% of the total variance, contrasts temperature and iron with conductivity (Figure 4, Table 3). This correlation highlights the influence of climatic conditions on groundwater mineralisation in the study area and on iron dissolution. The third component (Dim.3) explains
![]()
Table 3. PCA components with the contribution of each water quality variable, the eigenvalues and the cumulative percentages explained by the factors.
![]()
Figure 4. Principal component analysis of the first four factorial axes (Dim1, Dim2, Dim3 et Dim4).
8.42% of the total variance and is positively correlated with turbidity (Figure 4, Table 3).
The fourth component (Dim.4) is positively correlated with NH4 with 7.88% of the total variance explained (Figure 4, Table 3). Factor axes 3 and 4 indicate that this groundwater is in contact with septic tanks and latrines, which is the source of its organic pollution and the turbidity of the water.
The graphical representation in the factorial space of the individuals (Figure 5 and Table 3) show the distribution of the water points according to the different
Dim.1 and Dim.2 components. Analysis of the projection of individuals onto the Dim.1—Dim.2 plane shows five (5) main groupings of water samples with regard to their content of the different variables studied. The first grouping concerns all individuals whose content of the variables Na+, Ca2+, Mg2+, Cl−,
,
,
.
4.4. Hierarchical Component Classification
The dendrogram (Figure 6) derived from the Ascending Hierarchical Classification (HHC) revealed four (04) main classes of sampled water points. The first class accounts for 88% of the sampled water sites analysed. In terms of geographical location, the water points in this category are spread across the three hydrogeological zones. The majority of the samples from sites (10, 7, 12, 58, 8, 13, 38, 48, 53, 60, 5, 30, 68, 37, 47, 54, 50, 35, 46, 14, 62, 32, 6, 28, 69, 36, 41, 20, 61, 49, 67, 70, 19, 4, 17, 16, 39, 25, 22, 42, 18, 27, 29, 56, 66, 21, 23, 40, 24, 65, 64, 45, 43, 55, 57, 52, 15, 63, 59, 2, 51, 33). The second class, comprising 1%, includes water from samples (3) with a conductivity of 325 μS/cm. This class includes waters with a low mineral content that are also bicarbonated, either calciferous or sodic. The third class, made up of 6% of the sample waters (1, 34, 31, 11), has an electrical conductivity of between 204 and 1212 μS/cm. This class includes waters with a high mineral content and which are bicarbonated and chlorinated, either sodium or calcium.
Looking at Figure 6 and the first correlation circle (Figure 5), we see that the fourth class comprises 5% of the sample waters (26, 9, 44) with an electrical conductivity of between 269 and 749 μS/cm is highly mineralised, which is the average mineralisation of 1, 34, 11 and 31. This group of waters is less mineralised and are chlorinated, bicarbonated, either sodium or calcium.
![]()
Figure 6. Dendrogram of the ascending hierarchical classification (AHC) of water samples taken in the study area.
5. Discussions
The physico-chemical characterisation of groundwater in the town of Moundou shows the dominance of three facies: the chloride and sulphate calcic and magnesian facies (Cl−
Ca, Mg), the bicarbonate calcomagnesian facies (HCO3—CaMg) and the bicarbonate sodium potassium facies (HCO3—NaK). These three facies indicate mineral dissolution probably of carbonate and silicate minerals with sufficient aquifer recharge (Srinivasamoorthy et al., 2014; Ewodo et al., 2019) . Indeed, excess sodium, characterised by a ratio (Na/Cl) > 1), is linked to the alteration of silicates (Meybeck, 1987; Stallard & Edmond, 1983) .
A study of the correlations established between the concentrations of the main major elements (Cl−,
,
,
, Ca2+, Mg2+, Na+ and K+) and the in situ parameters (pH, electrical conductivity, TDS and temperature) of the water (Table 2) has made it possible to trace the origin of the mineralisation of the water in the town of Moundou. The presence of a positive correlation between major ions and conductivity indicates the participation of these elements in the acquisition of groundwater mineralisation. Alkalis (Na+ and K+): All the waters analysed showed higher concentrations of Na+ than K+. According to Banton et al. (1999) , the dominance of Na+ over K+ is due to the selective absorption of clays, K+ having a higher atomic mass than Na+, so the water contains more sodium than potassium. The levels of these two ions are also positively correlated at 0.61 (Table 2). Generally, sodium is present in some silicate minerals, but it comes mainly from marine water (however, our study area is not on the Atlantic coast, which would minimise the marine contribution). Ionic exchange is the most important chemical process affecting sodium concentration (Post, 2002) . Sodium and potassium could be the result of ionic exchanges with clay minerals, as well as pollution from human activities. Alkaline earths (Ca2+ and Mg2+): the relatively high Ca2+ content, in excess of 20 mg/l in certain water points, could be explained by leaching from the profile of soils rich in calcium minerals (Kamgang Kabeyene & Ekodeck, 1991; Vicat et al., 2002) . The correlation is positive between Mg2+ and Ca+ (0.88), which may be due to the fact that the dissolution of these two elements is related to the presence of bicarbonate ions, resulting in positive
Ca+ and
Mg2+ correlations of 0.99 and 0.95 respectively (Table 2). Nitrates: Nitrates are the main forms of nitrogen in water. They can be used as indicators of chemical pollution of water. Several authors (Antonakos & Lambrakis, 2000) link the presence of nitrates in water, in addition to the normal nitrogen cycle, to various sources: organic and inorganic chemical fertilisers, pesticides and herbicides, animal rearing facilities, domestic and industrial effluents (wastewater). In the study area, nitrate concentrations are high in some water points, with levels > 50 mg/l, which is the international drinking water standard (Organisation mondiale de la Santé & International Programme on Chemical Safety, 2000) . The high correlation between Cl− and
(0.86) and between
and
(0.68) in the groundwater studied has already been demonstrated in the groundwater of the town of Moundou and its origin attributed to anthropogenic inputs, fertilisers being the most likely form (Petelet et al., 2003; Mpakam, 2009) . Chlorides: Chloride levels are relatively high in groundwater. The presence of chloride ions in groundwater in the town of Moundou is characteristic of groundwater in the town and in Africa (Biémi, 1992; Oga et al., 2009) . The correlation coefficients (Table 2) between the Cl− ion and the other major elements (Mg2+, Na+ and K+) are strong (r2 > 0.50). Cl− ions in groundwater therefore come from several sources: atmospheric (Sigha-Nkamdjou et al., 2003) , alteration of silicate minerals, exchange phenomena (Cl−OH) and anthropogenic (Nguegang et al., 2008) : The presence of bicarbonates in the groundwater of the town of Moundou could come from several sources: the dissolution of CO2 originating from the atmosphere and the soil (Schwoerer et al., 1965; Kortatsi et al., 2007) .
Sulphates: Sulphates are naturally present in various minerals. They come from meteoric inputs (Kuitcha et al., 2012) and anthropogenic pollution (industrial effluents) (Nguegang et al., 2008; Kuitcha et al., 2010) .
6. Conclusion
The study carried out on the groundwater in the town of Moundou showed that the water is acidic with significant mineralisation and an average electrical conductivity of around 487.44 μS/cm. The hydrochemical classification of the water showed a dominance of the chloride and sulphate facies, calcium and magnesium facies, sodium and potassium facies and bicarbonate facies. The chloride facies reflects the anthropogenic contribution to the acquisition of mineralisation. This is also confirmed by the interpretation of the PCA, which shows that water quality in certain localities in the town of Moundou is strongly influenced by anthropogenic pollution.