1. Introduction
Continuous casting processes dominate steel production the world over due to their significant advantages, i.e., considerable energy saving, small waste material, improved labor productivity and reduced pollution. Tundish is the final and most important unit of a continuous casting process in which the final processing with liquid metal can be done before it reaches the mould. Since it is the final unit, tundish serves as a reservoir for the mold which also helps to avoid splashing and removal of inclusions particles resulting from various metal air interactions together with other impurities present in the molten steel.
A large number of studies have been reported in the literature dealing with the physical and mathematical modeling of fluid flow condition together with the inclusion motion behavior inside a tundish for different tundish geometries and flow conditions. Raghavendra et al. [1] investigated the behavior of inclusion in a four-strand tundish using the software OpenFoam. They concluded that the tendency of getting trapped at the top surface is more for inclusions with higher diameter as compared to that for smaller diameter inclusions. Singh et al. [2] studied the performance of a tundish possessing different sets of furniture using the residence time curve in a single-strand tundish. Zhang et al. [3] investigated the inclusion removal with the help of gas bubbling and concluded that bubbling affects large particles but tendency of floating out of small particle increases due to bubbling.
Yang et al. [4] used water model, mathematical modeling and industrial trials to design and optimize the configuration of a tundish. Hryb et al. [5] developed Eulerian and Lagrangian formulations to model particle transport in a turbulent flow. They concluded that Lagrangian formulation provides better physics as compared to Eulerian approach. Hou et al. [6] developed physical and mathematical methods for a swirling flow tundish and concluded that such tundishes have higher capacity for the inclusion removal as compared to that of a tundish equipped with turbulence inhibitors. Gang et al. [7] performed water modeling experiments and concluded that a tundish equipped with dam and turbulence inhibitors has a significant effect on the inclusion separation.
Lei and He [8] developed a three-dimensional, Eulerian-Lagrangian method to predict the dynamic growth of alumina inclusion in a continuous caster. They concluded that the cluster formation depends on turbulent flow which determines the inclusion growth mechanism. Zhang [9] used the k-ε model of turbulence to simulate the fluid flow within a single-strand tundish. He concluded that the stochastic model produces more accurate inclusion motion as compared to that by a non-stochastic model. He also concluded that the investigation of the residence time of particles in molten steel is not so meaningful for the motion of inclusions. Merder et al. [10] investigated flow inside a six-strand tundish with and without dam. They obtained residence time curves to verify whether tundish condition is suitable for non-metallic inclusion removal or not.
Tripathi and Ajmani [11] modeled three-dimensional fluid flow characteristics inside a six-strand tundish whose one side was curved and compared its performance with that of a delta shaped tundish. They concluded that the curved surface plays a strong role in improving the characteristics for inclusion flotation. Rogler [12] developed a mathematical model of inclusion removal by the gas bubbling and concluded that for better separation efficiency bubbles having diameter less than 1.0 mm should be used. Jha and Dash [13] used different models of turbulence and concluded that the standard k-ε model predicts flow properties closest to experimental measurements as compared to those using the RNG k-ε model. Therefore the standard k-ε model is used in the present study.
Morales et al. [14] used water modeling and mathematical simulation techniques to conclude that the presence of turbulenceinhibitors increases product quality in a four-strand bloom caster tundish. Zhang et al. [15] calculated the inclusion removal in a single-strand tundish with and without flow modifiers. They concluded that the collision of inclusion and adhesion to the solid surfaces also plays an important role in the removal of inclusions and flow control devices favor the inclusion removal. Miki and Thomas [16] developed mathematical models to predict the removal of aluminum inclusions from the molten steel for both isothermal and non-isothermal conditions in a single-strand tundish and compared their predictions with the experimental steel samples. They found that the non-isothermal model along with the random walk model was much more consistent as compared to the isothermal model. Therefore in the present study nonisothermal model along with random walk model is considered.
Zheng and Zhu [17] developed a new approach to estimate level fluctuations in a thick slab continuous casting mold with argon blowing. They observed that the level fluctuation occurs mainly near the nozzle wall and it increases with gas injected to the SEN. Ding et al. [18] plotted residence time curves to optimize the flow control devices in a single-strand tundish. They found that with an optimized geometry inclusions area ratio decreases by 32%. Yao et al. [19] investigated the influence of inclusions on fatigue strength of a powder metallurgy tool and observed that the inclusions having sizes more than 30 μm promoted the occurrence of sub-surface crack initiation and decreased the fatigue strength. Meijie et al. [20] showed, by using residence time curve, that the removal of small size inclusions from the molten steel increases with the help of argon blowing at the bottom of the tundish. From industrial experiments they concluded that the content of inclusions having diameters less than 20 µm decreases by 24% with proper blowing conditions.
Most studies reported in the literature deal with singlestrand tundishes. The present study deals with a multistrand tundish. Such tundishes are being increasingly used in continuous casting processes. Many studies reported in the literature use the residence time curves to predict the inclusions behavior inside the tundish. In the present study Lagrangian motion of inclusions is tracked and it provides a good insight into the behavior of tundish. The geometry of the tundish should be such that it separates maximum percentage of inclusions present in the molten steel. Being lighter than steel, inclusions can be removed by trapping at the top surface. The use of flow modifiers is reported to promote the flow field towards the top surface which increases the inclusion removal efficiency of a tundish. The arrangement of flow modifiers used for enhancing inclusion separation efficiency should be such that temperature and percentage of inclusion at each outlet are nearly equal to produce the same quality of steel from each strand. Moreover, tundish should be subjected to modification only at the interior geometry and at the same time, it should not be expensive to fabricate.
With continuous research efforts over the last decade, computational fluid dynamics (CFD) has now become an effective tool for the design of steel tundishes. In the present study, 3-D, turbulent flow field in a six-strand trough type tundish is investigated using the standard k-ε model of turbulence. The finite volume based commercial software FLUENT 6.3.26 is used for performing the simulations. The flow fields thus obtained are used to predict the inclusion separation by numerically solving the inclusion transport equation, considering its drag and buoyancy forces. To simulate the chaotic effect of turbulent eddies on particle motion a discrete random walk model is used. The effects of flow modifiers, i.e., dam and weir, together with their height and molten steel stream velocity at the inlet gate on the inclusion motion (for different particle size and density) and outlet temperature of liquid steel have been investigated. The geometry of the multi-strand tundish is described in Section 2. The governing equations, turbulence model and boundary conditions are presented in Section 3. The code validation and results are presented in Section 4 followed by conclusions.
2. Physical Description of the Problem
The geometry of multi-strand tundish considered in the present study is taken from the study reported Merder et al. [10]. The tundish considered in the present study (Figures 1-3) is an example of trough-type tundish used for casting ingots employed for small cross-section rolled products, such as wire, rods, etc. Half of the tundish is shown because of the symmetry relative to the transverse plane passing through the inlet. The detailed constructional parameters are: L1 = 2785 mm, L2 = 2700 mm, L3 = 300 mm, L4 = 500 mm, L5 = L6 = 1000 mm, W1 = 1040 mm, W2 = 850 mm, W3 = 640 mm, W4 = 450 mm and steel bath height H = 740 mm. The inlet and outlet diameters of the gate are taken as 66 mm and 14 mm, respectively [10].

Figure 1. Geometry of six-strand trough type tundish considered in the present study.

Figure 2. Top plane view of the tundish.
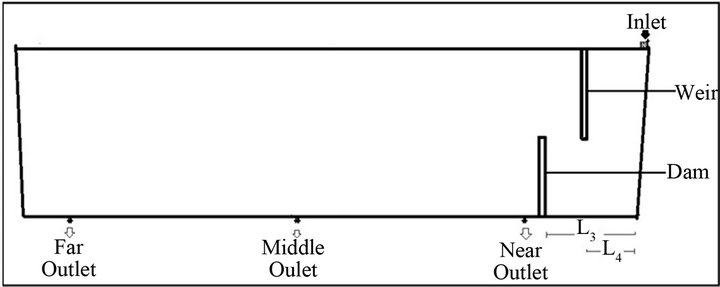
Figure 3. Front plane view of the proposed six-strand tundish with flow modifiers.
Figure 3 shows the proposed tundish with the flow modifiers. The dimensionless height (h*) is defined as the ratio of dam/weir height (h) to the bath height of the tundish (H). In all the cases considered in the present paper, the height of the dam measured from the bottom and the height of the weir measured from the top surface were taken to be the same. Here F.O. refers to the far outlet strand, M.O. to the middle outlet strand and N.O. to the near outlet strand. Four different values of h* (0, 0.25, 0.5, 0.75) were used to study the effect of height on the inclusion removal efficiency of the tundish. The values of the properties of liquid steel used are [10]: specific density = 7010 kg/m3, specific heat = 821 J/kg·K, thermal conductivity = 30.5 W/m·K and viscosity = 0.007 kg/m·s.