Upgrading of Biomass-Derived Feedstocks to Liquid Transportation Fuel Precursors by Aldol Condensation ()

1. Introduction
World use of petroleum and other liquid fuels is projected to grow from 90 million barrels per day (b/d) in 2012 to 100 million b/d in 2020 and to 121 million b/d in 2040 [1] . Most of the growth in liquid fuels consumption is in the transportation and industrial sectors. The environmental impacts associated with greenhouse gas emissions (GHG) emissions resulting from combustion of fossil fuels have motivated the search of new technologies for a sustainable production of transportation fuels [2] . Utilization of renewable resources for the preparation of transportation fuels has potential advantages in the reduction of our dependence on fossil resources [3] . Biomass is a renewable resource that can also reduce GHG by capturing CO2 during photosynthesis [4] . There are several methods to convert biomass into liquid transportation fuels including fast pyrolysis [5] [6] , liquefaction [7] [8] and Fischer Tropsch synthesis [9] . These strategies convert biomass to upgradeable platforms such as bio-oil or syngas [10] . Another important route involves aldol condensation of biomass-derived feedstocks to upgrade small oxygenates to larger ones that are more similar in molecular size to conventional fuels. These biomass-derived feedstocks include methyl ketones [11] , n-Butanal [12] , 5-Hydroxymethylfurfural [13] [14] [15] and other ketones and aldehydes. Aldol condensation has a number of advantages compared with fast pyrolysis, liquefaction, and Fischer-Tropsch synthesis. For example, extreme temperature and pressures are not required, unlike Fischer-Tropsch synthesis or liquefaction. In addition, this process has much higher selectivity compared with fast pyrolysis, which produces a wide variety of products.
Herein, we review the most advanced progress in the use of aldol condensation as a method for preparation of liquid transportation fuels. In particular, we focus on that catalysts achieve both a high conversion and a high selectivity. Because hydrodeoxygenation has been intensively summarized and reviewed by many research groups [16] [17] , we do not discuss this field in this review.
2. Mechanism of Aldol Condensation
Although aldol condensation is an important and well-known organic reactions because of its ability to form new C-C bonds, its mechanism has never been fully established [18] . Aldol condensation includes reactions producing β-hydroxy aldehydes or β-hydroxy ketones by self condensation or mixed condensation of aldehydes and ketones as well as reactions leading to α, β-unsaturated aldehydes or α,β-unsaturated ketones formed by dehydration of intermediate β-aldols or β-ketals.
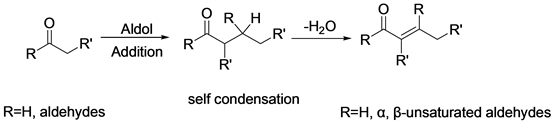
Aldol condensation of aldehydes can be catalyzed by acid, base or acid-base bifunctional catalysts [19] [20] . In aldol condensation catalyzed by base (Scheme 1), an enolate is formed by the abstraction of the α-hydrogen of the carbonyl compound with the help of a base. The enolate then attacks the second carbonyl molecule. This leads to formation of another ionic species which extracts the proton from the protonated base (HB). This finally leads to the formation of the aldol (or if a ketone was present as the starting material, a ketol). Next the aldol

Scheme 1. Aldol condensation catalyzed by base.
(or ketol) undergoes spontaneous dehydration due to base catalyzed dehydration to yield the α,β-unsaturated aldehyde or α,β-unsaturated ketone.
Which step is the rate-limiting step of the aldol condensation was discussed [18] , rate-limiting step in the base-catalyzed aldol condensation of benzaldehydes with acetophenones is the final loss of hydroxide and formation of the C=C bond. When an acid is used to catalyze aldol condensation (Scheme 2), the acid acts as a proton donor and activates the carbonyl oxygen into a protonated form. The protonated carbonyl then reacts with the conjugate base of the acid to produce the enol, followed by conjugation of the enol with another protonated carbonyl compound to produce the aldol (or ketol). Completing the reaction, the aldol (or ketol) undergoes spontaneous dehydration due to acid catalyzed dehydration to yield the α,β-unsaturated aldehyde or α,β-unsaturated ketone.
Aldol condensation is generally carried out in the presence of base catalysts. Including basic compounds (alkali metals or alkaline earth oxides), hydrogen oxidation, bicarbonate, carbonate, carboxylate, organic amine compounds, Anion exchange resin and so on. The solid base catalysts that are active in organic liquid phases or in the vapor phase. Few studies have been reported for aldol condensation using solid base catalysts in water, because leaching of catalyst components into the water phase and poor hydrothermal stability pose significant challenges. In actual industrial applications, the alkaline catalysts used in the aldol condensation reaction can be weak bases (such as sodium carbonate, sodium bicarbonate, sodium acetate), or strong bases (such as sodium hydroxide, calcium hydroxide, sodium hydroxide, sodium alcohol, etc.). Weak bases are usually used for condensation between active aldehydes. Products are generally β-hydroxyl compounds. Strong bases are usually used for condensation of ketone with large steric hindrance. Acid catalysts are often used in aldol condensation reactions [19] [20] . Commonly used acid catalysts include (VO2P2O7, VOHPO4), niobate and MFI zeolite. Acid-base catalyst also has an acidic alkaline active site, such as two oxide oxides or hydrotalcite which is suitable for gas phase aldol condensation and liquid phase. The application of acid-base catalyst in aldol condensation reaction is more and more important.
3. Aldol Condensation of Biomass-Derived Carbonyl Molecule
3.1. Aldol Condensation between 5-Hydroxymethylfurfural and Acetone
5-hydroxymethylfurfural (HMF) is a suitable platform chemical for the production of useful chemicals and fuels [21] . Recently, efforts have been devoted to the

Scheme 2. Aldol condensation catalyzed by acid.
conversion of biomass into 5-hydroxymethylfurfural (HMF) (Table 1), and excellent efficiencies have been achieved for both fructose and glucose. The HMF yields were as high as 96% and 81% for fructose and glucose, respectively [22] . Typically, aldol condensation furfural and ketones is catalyzed by solid base catalysts [23] [24] .
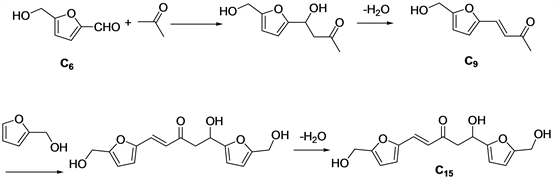
Once produced, HMF can be combined with other aldehydes and ketones to produce longer carbon chains that can eventually be converted to hydrocarbon fuels, A variety of solid base catalysts have been used for aldol condensation between HMF and acetone. Zirconium carbonate Zr(CO3)x was used as a water tolerant solid base catalyst, producing 92% C9 aldol products at nearly 100% HMF conversion. The activity of Zr(CO3)x was maintained for five consecutive cycles. Aldol products were further hydrodeoxygenated with a bifunctional Pd/Zeolite catalyst in ethanol, producing n-nonane and 1-ethoxynonane with 40% and 56% selectivity, respectively. Basic MgO-ZrO2 [25] , MgAl and MgZr mixed oxides have also been studied for aqueous-phase 5-hydroxymethylfurfural-acetone aldol condensation. The highest C15 selectivity is obtained in stoichiometric conditions (2:1). MgZr catalyst shows better than MgAl to aldol condensation products, especially for the formation of the second adduct (C15). The best results were obtained working with 0.5 g of MgZr, at 323 K. The highest C15 yield was reached with 5-HMF excess (2:1) (16.1%), whereas the whole yield is maximized at equimolar conditions (37%). CO2-Catalysed aldol condensation of 5-hydroxy-methylfurfural and acetone to a jet fuel precursor was reported by Roland Lee et al. [26] , CO2-Catalysed aldol condensation of HMF with acetone gives a >95% yield, with the mono-aldol condensate as the only detected product. Cu/MgAl2O4 as bifunctional catalyst for aldol condensation of 5-Hydroxymethylfurfural and acetone [27] , magnesium aluminate exhibits superior activity compared to zinc and cobalt-based aluminates, reaching full conversion and up to 81% yield of the 1:1 aldol product. The high activity can be
![]()
Table 1. Heterogeneous catalysts for aldol Condensation between 5-Hydroxymethylfurfural and acetone.
correlated to a higher concentration of basic surface sites on magnesium aluminate. Applying continuous regeneration, the catalysts can be recycled without loss of activity. The Cu/MgAl2O4 catalyst was used in the hydrogenation of condensation products and showed high selectivity for C-O bond cleavage of primary alcohols, delivering 3-hydroxybutyl-5-methylfuran as the main product. Amine-grafted hierarchical basic FAU-type zeolite nanosheets for the aldol condensation of 5-hydroxymethylfurfural (5-HMF) and acetone (Ac) has been studied [28] . The superior catalytic performance achieved due to the synergistic effect of hierarchical structures, featuring basic active sites together with surface modification. Theoretical study on the reaction mechanisms of the aldol-condensation of 5-hydroxymethylfurfural with acetone catalyzed by MgO and MgO+ were reported [29] , The reaction mechanism involves the crucial reaction steps of H-shift and C-C bond formation, while the rate-determining step is concerned to the H-shift. The Lewis acidity of the cationic MgO+ makes it exhibit better catalytic performance than the neutral MgO. Water, as a bridge in the H-shift, interacts with both the neutral MgO and the cationic MgO+ moieties, leading to the Brønsted basicity, and then promoting the catalytic performance both of the neutral MgO and the cationic MgO+. Both the Lewis acidity and Brønsted basicity of active sites can enhance the catalytic performance in the aldol-condensation of HMF with acetone.
3.2. Condensation of Biomass-Derived Aliphatic Ketones
Ketones can be produced from biomass-derived sugars, suggesting that they could be possible starting compounds for synthesis of liquid fuels. For example, it was reported that acetone/butanol/ethanol (ABE) mixtures produced by Clostridial fermentation of sugars can be catalytically converted to aliphatic ketones [30] Potential starting ketone such as 2-butanone which can be produced by acid-catalyzed dehydration of fermentative 2,3-butanediol [31] 2,3-butanediol can be produced with concentrations up to 152 g/L [32] by various bacterial strains belonging mainly in the genera Klebsiella, Serratia, Bacillus, Paenibacillus and Enterobacter. A mixture of n-alkyl methyl ketones and their derivatives obtained from bio-mass via base catalyzed aldol condensation and Michael addition, further hydrodeoxygenation of these products, possible to produce jet fuel. Condensation of aliphatic ketones is a very complex reaction and numerous products are possible via competitive self-condensation and cross-condensation between the same or different ketones that are formed in the reaction.
Typical reactions of self-condensation of ketones in the presence of MgAlO or niobic acid catalysts as shown in Scheme 3.
In the presence of base (MgAlO), the selective of C3n isomers (3) is higher than C2n isomers and C3n isomers (4) [33] .
Mg-Al Hydrotalcite Catalysts was also used to aldol condensation of benzaldehyde and acetone, hydrotalcites should transformed into basic solids by activation, the activity went through a maximum after calcination at 723 K, followed by rehydration by water vapor at room temperature. It was suggests that aldolisation is catalysed by OH− [34] .
An aldol yield higher than 85 mol% was obtained after optimum activation.. Highly selective trimerization of methyl ketones catalyzied by MgAlO to produce bio-lubricants were reported [35] (Scheme 4).
Calcined niobic acid (Nb2O5) contains a considerable amount of water, nevertheless it shows a high acidity on the surface corresponding to the acidity of 70% sulfuric acid [36] . Nb2O5 exhibits not only a high activity and selectivity but also a remarkably good stability as a catalyst for the reactions in which water molecules participate or are liberated [37] . However, in the presence of acid (Nb2O5) catalysts, hindered internal ketones condense to form C2n isomers (2), ketones containing methyl functionality at one end form C3n isomers (4), mainly aromatic compounds. Acid catalysts Ta2O5/SBA-15 was also used to self-condensation of biomass-derived methyl ketones, which promote dehydration leading to the production of aromatic products.
3.3. Aldol Condensation of Acetoin with Lignocellulose Derived Aldehydes
Acetoin, a novel C4 platform molecule derived from new ABE (acetoin-butanol-ethanol) type fermentation via metabolic engineering [38] , can be used as a bio-based building block for the production of liquid hydrocarbon fuels [39] , as shown in Scheme 5.
The condensation reaction between two acetoin molecules is slow because of steric hindrance and because the electron donating properties of the attached

Scheme 3. Self-condensation of ketones in the presence of solid acid/base catalysts.

Scheme 4. Aldol self-condensation of n-alkyl methyl ketones.
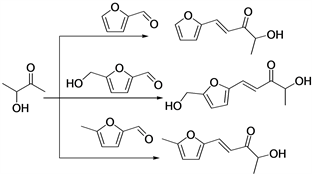
Scheme 5. Synthesis of diesel or jet range alkanes using acetoin as a bio-based synthon.
hydroxyl groups prevent the formation of the reactive enolate intermediate. However, acetoin easily reacts with lignocellulose derived aldehydes, including 5-hydroxymethylfurfural or 5-methylfurfural. Solid base catalysts were tested for furfural and acetoin, except for MgO-ZrO2 exhibiting moderate catalytic activity, other solid base were unsatisfactory. Organocatalysts such as L-proline, tryptophan, and 1,8-diazabicycloundec-7-ene were failed to catalyze this aldol reaction.
3.4. Aldol Condensation of Furfural with Acetone
Furfural and acetone are readily available chemicals, which can be produced by the hydrolysis of biomass [40] .
Liquid phase aldol condensation of furfural and acetone catalyzed by solid base catalysts (Mg-Al hydrotalcites and Mg-Al mixed oxides) has been investigated. The best results were achieved with calcined catalyst sample having Mg/Al molar ratio equal to 3 at 100˚C (>95% furfural conversion and > 90% selectivity to the 4-(2-furyl)-3-buten-2-one and 1,5-di-2-furyl-2,4-pentadien-1-one). The catalytic performance of the prepared TiO2 sample in aldol condensation of furfural with acetone was evaluated and compared with those for Mg-Al hydrotalcites [41] and BEA zeolite [42] (Scheme 6). It showed that uncalcined TiO2 possesses good activity which could be competitive with that reported for other inorganic solids. Aldol condensation between aldehyde and ketone over TiO2 proceeded with the participation of basic rather than acidic sites [43] . Potassium-containing BEA zeolites were prepared by ion-exchange from NH4-BEA with potassium nitrate aqueous solution or ion-exchange combined with impregnation were used as basic catalysts for aldol condensation of furfural and acetone [44] . The ion-exchanged K-BEA catalysts exhibited low activity in the aldol condensation because of a weak strength of intrinsic basic sites. In contrast, the samples prepared by ion exchange combined with impregnation possessed strong basic sites, plausibly K2O clusters, and demonstrated appreciable activity
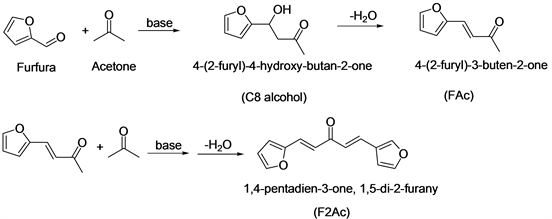
Scheme 6. Aldol condensation of furfural with acetone.
in the aldol condensation. Solid base catalysts MgO-ZrO2, NaY and nitrogen-substituted NaY (Nit-NaY) were studied for aldol condensation reactions, The aldol condensation of furfurals with acetone produces two different products, the monomer and the dimer. The monomer is formed from reaction of furfurals with acetone. The dimer is formed from reaction of the monomer with furfurals. MgO-ZrO2 had a higher selectivity towards dimer formation. In contrast, Nit-NaY was more selective towards the monomer product due to the cage size in the FAU structure, indicating that Nit-NaY is a shape selective base catalyst.
3.5. Aldol Condensation of Biomass-Derived Levulinic Acid and Furfural
Levulinic acid (LA) is one of the most promising platform chemicals derived from biomass. The efficient production of LA (about 80% yield) from biomass has been achieved on a large scale through the Biofine Process [45] . The aldol condensation of furfural with levulinic acid in the aqueous phase was investigated over a series of solid catalysts [46] (Scheme 7), including oxides (MgO, ZnO, TiO2, ZrO2, MgO-Al2O3, CeO2, Nb2O5, SnO2, and WO3) and acidic zeolites (HY, Hβ, HZSM-5, H-MOR, and SAPO-34).
MgO is an effective basic catalyst for synthesis of C4 and C8 chemicals from ethanol [47] . The aldol reaction of levulinic acid and furfural on MgO is supposed to be difficult. Own to both types of α-H in the levulinic molecule could be activated by a base, which resulted in a mixture of two C10 oxygenates with poor selectivity. Two isomeric condensation products, β- and δ-furfurylidenelevulinic acids (β- and δ-FDLA) were produced via two fundamentally different mechanisms. The formation of δ-FDLA on MgO follows a typical base-catalyzed mechanism, while the formation of β-FDLA on ZnO is via an acid-catalyzed mechanism. LA could then be used as a cheap and easily available biomass feedstock for further transformations by aldol condensation.
3.6. Aldol Condensation of Furfural with Cyclopentanone
The furfural conversion about 98% and cyclopentanone yield of 92.1 mol% were achieved using only 1 wt% concentration of 5% Pd - 10% Cu/C catalyst and a reaction time of 1 h [48] . The product of Aldol condensation of furfural with
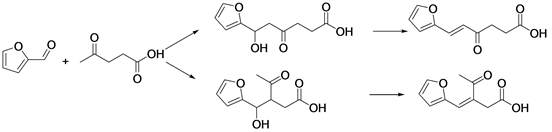
Scheme 7. Aldol condensation of levulinic acid and furfural.
cyclopentanone yields a cyclic ether that could be considered as biomass-derived diesel blending agent for transportation fuels [49] (Scheme 8).
The aldol condensation of furfural with cyclopentanone is achieved with a low concentration of base and a molar ratio of the reactants of 2:1. This reaction is highly selective. After 40 - 80 min of reaction at a temperature of 40˚C - 100˚C, more than 95 mol% yield of 2,5-bis(2-furylmethylidene) cyclopentan-1-one was obtained [50] . Subsequent hydrogenation/hydro-deoxygenation steps yield a dialkylcyclopentane that could be used as a diesel or jet fuel.
3.7. Self-Condensation of Levulinic Acid
Most of previously studied reactions are focused on the reactivity of the carboxyl group of the levulinic acid, transformation of levulinic acid into g-valerolactone with industrial interest [51] . The base-catalyzed condensation of levulinic acid (LA) under mild conditions was proposed as a new approach for the upgrading of this molecule by using mixed oxides as catalysts [52] (Scheme 9).
Aldol condensation of levulinicacid would yield a mixture of C10-C15 adducts. These adducts could have high value as fuel additives or surfactants, and hydrodeoxygenation of these compounds would result in a good-quality liquid fuel without needing further purification steps. MgAl, MgZr and MgZr/HSAG were used as catalyts. The best results were obtained with MgZr, for which a 33% conversion of levulinic acid was observed after 24 h with almost 83% selectivity for the formation of condensation products.
Nb2O5 catalyst with excellent activity and stability in the aldol condensation of biomass-derived carbonyl molecules (Scheme 10). It is found that in the aldol condensation of furfural with 4-heptanone, Nb2O5 has obviously superior activity, which is not only better than that of other common solid acid catalysts (ZrO2 and Al2O3), more importantly, but also better than that of solid base catalysts (MgO, CaO, and magnesium-aluminum hydrotalcite) [53] . Optimization of the process conditions for minimizing the aldol condensation recently reported by many research groups [54] [55] .
4. Conclusions
While some interesting information has been forwarded by aldol condensation of biomass-derived feedstocks studies, several gaps remain in this very important research area. These gaps can be divided into economic and technology gaps. From an economical viewpoint, economic feasibility plays a crucial role in the
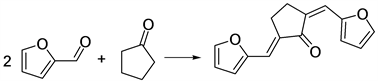
Scheme 8. Aldol condensation of furfural with cyclopentanone.
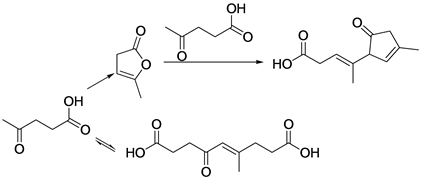
Scheme 9. Self-condensation of levulinic acid.
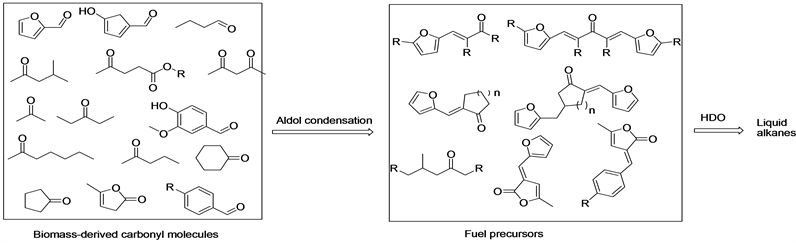
Scheme 10. Aldol condensation of biomass-derived carbonyl molecules.
field, and fuels are a low-value commodity produced on a very large scale. Therefore, development of economical processes for fuel production requires a large investment in both money and time. The cost of producing transportation fuels from biomass is controlled primarily by the costs associated with the processing of biomass to produce the fuel, making it imperative to develop new processes for the conversion of biomass to liquid fuels, which involves a limited number of processing steps. However, no literature is available to offer detailed information on the energy recovery and economic analysis of the heterogeneous catalytic conversion of biomass-derived feedstocks in the aqueous phase. Given that water has a higher heat capacity and results in worse corrosion than organic solvents, the energy consumption of water and equipment maintenance may lead to higher costs. Hence, a reliable study of economic feasibility should be done to design an efficient process.
From a technical viewpoint, extensive research on biomass-derived feedstocks, catalyst systems as well as reactor technology is well studied in this area. The fundamental chemistry of most of these reactions is not well understood, and it is likely that further scientific understanding will lead us to improved processes. To obtain high-quality fuels, multiple transformation routes are required to increase the carbon-chain length and to decrease the oxygen content. A continuous or one-pot process can be designed to meet this requirement. We believe that efficient processes for the sustainable production of biofuels will be continuous in the future.
Acknowledgements
We gratefully acknowledge support from Zhejiang public welfare technology research plan (LGJ20B060001).