Biodegradation of Styrene-Butadiene-Styrene Coploymer via Sugars Attached to the Polymer Chain ()
1. Introduction
Commodity elastomers like SBS have become an integral part of our society. However, these synthetic polymers resist microbial degradation when discarded in landfills. One possible method of introducing biodegradability into non-biodegradable hydrocarbon polymers is to introduce some functional groups along the SBS polymer chain [1]. A functional group when incorporated into a polymer can deliver enhanced properties, such as biocompatibility [2], antimicrobial property [3], biodegradation [4] etc., as evidenced by numerous reports in scientific literature. Synthesis of synthetic polymers having sugar branches (synthetic glycopolymers) has become an interesting area of work because of the importance of these materials as renewable materials and also due to their numerous biological and biomedical applications, including use as biodegradable polymers.
Although some aspects of functionalization of polybutadiene, such as synthesis of hydroxy-terminated polybutadiene and reaction of epoxidized cis-1,4-polybutadiene with p-aminodiphenylamine to obtain antioxidant properties [5-7], and functionalization of low molecular weight oligomers of poly(butadiene-co-acrylic acid) with sucrose [8,9] have been studied, there are no reports on functionalization of SBS with sugars. While there exists a significant body of literature on biodegradable plastics, there are hardly any reports on introducing biodegradability to synthetic elastomers like SBS. Such biodegradable elastomers can have interesting applications in packaging applications because of their film forming abilities.
It has previously been demonstrated in our lab that functionalization of maleated polystyrenes with minute quantities of sugars leads to a significant increase in the extent of their biodegradation [10,11]. Our very recent work has further demonstrated that functionalization of a commodity elastomer like SBS with sugars is possible by employing click chemistry technique; preliminary studies showed such polymers biodegrade to a significant extent [12]. In this paper we present our more detailed work on biodegradation of such polymers, including evaluating the changes occurring in their antimicrobial properties, since these polymers also contain some quaternary nitrogen atoms in the form of pyridinium groups onto the backbone of the polybutadiene fraction of SBS polymer [13].
Biodegradation studies were conducted for a period of 30 days using pure soil fungal and bacterial cultures. The fungal culture used in this study was Aspergillus niger (NCIM 1025) and the bacterial culture was Pseudomonas sp. (NCIM 2220). Biodegradation was monitored by weight loss in case fungal culture and by optical density (OD) in case of bacterial culture. The observed degradation was further corroborated using SEM studies of the elastomer before and after biodegradation. The effect of minute quantities (0.42 - 0.46 atomic%) of quaternary nitrogen pendants on biodegradation and antimicrobial properties was also evaluated.
2. Experimental
2.1. Materials
SBS (Mw 140,000, 30% polystyrene, 70% polybutadiene) was obtained from Aldrich, USA, Chloroform (HPLC grade) was purchased from Spectrochem, while isopropyl alcohol (HPLC grade) was purchased from SRL, India.
2.2. Synthesis of Sugar Linked SBS
Sugar molecules (glucose, glucose, mannose, galactose, maltose and xylose) and quaternary nitrogen were anchored into SBS by a procedure reported earlier (12,13). The sugar content of all the sugar linked SBS was determined by phenol sulphuric acid assay [14]. Details of the composition of the polymers are given in Table 1. The chemical structure of one representative SBS molecule with glucose anchored on it by click chemistry technique is shown in the Scheme 1.
2.3. Scanning Electron Microscopy (SEM)
The surface morphology of SBS and functionalized SBS was investigated using Leica SEM stereoscan 440, Cambridge, UK. In order to achieve conducting surface, the polymeric samples in the form of thin films, were coated with gold in a sputter coater.

Scheme 1.Idealized structure of glucose/acetylated glucose attached to the polybutadiene fraction of SBS (see ref. [12]).

Table 1. Surface composition of functionalized SBS polymers films for sugar and nitrogen content by different techniques.
2.4. Preparation of Polymeric Films
Sugar functionalized SBS containing quaternary nitrogen pendants were dissolved in CHCl3 with overnight stirring to ensure dissolution. 10% (w/v) solutions of these polymers were cast into films in Petri dishes by slowly evaporating the chloroform solution at room temperature. These films were used for antimicrobial activity studies.
2.5. Biodegradation
The microorganisms Aspergillus niger NCIM 1025 (ATCC 9642) and Pseudomonas sp. NCIM 2220 were obtained from the National Collection of Industrial Microorganisms, National Chemical Laboratory, Pune, India. These cultures were routinely maintained on Potato Dextrose Agar (PDA) slopes. The methodology followed was the same as our earlier published work [10,11].
2.6. Minimal Medium Preparation
2.6.1. Fungal Culture
The culture was grown in minimal medium (ASTM Nutrient Salts Medium) containing (g/l): KH2PO4 0.7; K2HPO4 0.7; MgSO4∙7H2O 0.7; NH4NO3 1.0; NaCl 0.005; FeSO4∙7H2O 0.002.; ZnSO4∙7H2O 0.002.; MnSO4∙ H2O 0.001. The pH of the medium was adjusted to 6.5 ± 0.2 and sterilized at 121˚C for 20 minutes.
2.6.2. Bacterial Culture
Cultures were grown in Minimal medium (OECD) containing (g/l): (NH4)2SO4, 2.0; K2HPO4, 14.0; KH2PO4, 6.0; MgSO4.7H2O, 0.2. The pH of the medium was adjusted to 7.0 prior to sterilization. The medium was sterilized at 121˚C for 20 min.
2.6.3. Testing of the Samples
The test samples were surface sterilized with 70% ethanol overnight and then added separately to the sterilized medium. The cells of the cultures grown in 10 mL Nutrient broth for 24 h at 30˚C were suspended in 10 ml of saline and this suspension was used as an inoculum. Approximately 1 mL (~108 cells) was inoculated into 50 ml of the minimal medium in 250 ml conical flasks. The flasks were incubated at 28˚C with shaking at 180 RPM. The growth was monitored over a period of four weeks. For bacterial culture optical density was measured at regular interval using Systronics 117 spectrophotometer. After 30 days the polymer was separated from the cells. The polymer was further washed many times with water followed by washing with 70% ethanol. It was dried at 50˚C and analyzed by scanning electron microscopy (SEM). Percentage weight losses of the polymers were also recorded.
2.7. Antimicrobial Activity
The bacterial cultures (E. coli DH5α and Bacillus subtilis RCK (Accession no. AJ937677; this culture has been deposited in the National Centre of Biotechnology Information (NCBI) Genbank in India), were procured from the culture collection of Lignocellulose Biotechnology Laboratory, Department of Microbiology, University of Delhi South Campus, New Delhi, India, and were maintained on Luria-Bertani (LB) agar plates at 37˚C. Pichia stipitis NCIM 3497 and P. stipitis NCIM 3499 were procured from National Collection of Industrial Microorganisms, National Chemical Laboratory, Pune, India and were maintained at 30˚C on YPD medium containing (g/L): Yeast extract 3.0, Peptone 5.0, Dextrose 20.0, agar 20.0 and pH 5.5. All cultures were subcultured fortnightly and stored at 4˚C.
For the inoculum preparation of bacterial culture, a loopful of cell mass from pre-grown agar plates was inoculated into sterile 50 mL Luria-Bertani (LB) broth in a 250 mL Erlenmeyer flask and incubated at 37˚C and 200 rpm for 16 h. While, the inoculum of yeast cultures was prepared by inoculating a loop-full of cell mass from pre-grown agar plates into 50 mL of YPD broth in a 250 mL Erlenmeyer flask and incubated at 30˚C and 150 rpm for 24 h.
Anti-microbial effect of the polymeric films was studied by adding 25.0 mg of each film separately in 50.0 mL sterilized LB broth for bacterial cultures and YPD broth for yeasts, in 250.0 mL Erlenmeyer flasks. Each flask was inoculated with bacterial or yeast culture (0.2% v/v) grown for 16 and 24 h, respectively. The bacterial cultures were incubated at 37˚C and 200˚C rpm in a rotatory incubator shaker, while the yeasts were grown at 30˚C and 150˚C rpm. The samples from each flask were taken after 24. The cell growth was analyzed by measuring the optical density (OD) at 600 nm in a UV-Vis spectrophotometer (Analytical Jena, Specord 205, Germany). The flask without the polymeric films served as control.
3. Result and Discussions
The functionalized SBS elastomers were characterized for sugar and nitrogen content. The sugar content of the functionalized SBS ranged from 0.09 - 0.37 (weight%). Atomic concentration (%) of these elastomers was determined by XPS analysis and the total nitrogen content as determined ranged from 1.53 - 1.68 (atomic wt%), as summarized in Table 1.
3.1. Biodegradation Studies of Sugar Linked SBS
We anchored minute quantities of various sugars like glucose, galactose, mannose, xylose, maltose and sucrose with the aim of improving biodegradation of SBS. Instead of using a mixture of several microorganisms, we chose a single soil bacterial culture (Pseudomonas sp.) and fungal culture (Aspergillus niger) for investigating their individual growth patterns on these new SBS elastomers, and compared their growth with that of a control solutions containing unmodified SBS elastomer as well as without any polymers.
3.2. Bacterial Biodegradation by Pseudomonas sp.
Test organisms used in the present study were standard cultures used for testing samples for their bacterial resistance. The test cultures used in the present study are organisms widely found in the environment. However, use of pure culture system offers the advantage of reproducibility of results, and would aid in designing biodegradation systems for different polymers after their disposal as waste materials. Since Pseudomonas sp. is a common soil bacteria, therefore these results have implication for disposal of these polymers in soil for obtaining biodegradation. The bacteria mediated biodegradation of sugar linked SBS by Pseudomonas sp. is presented in Figure 1.
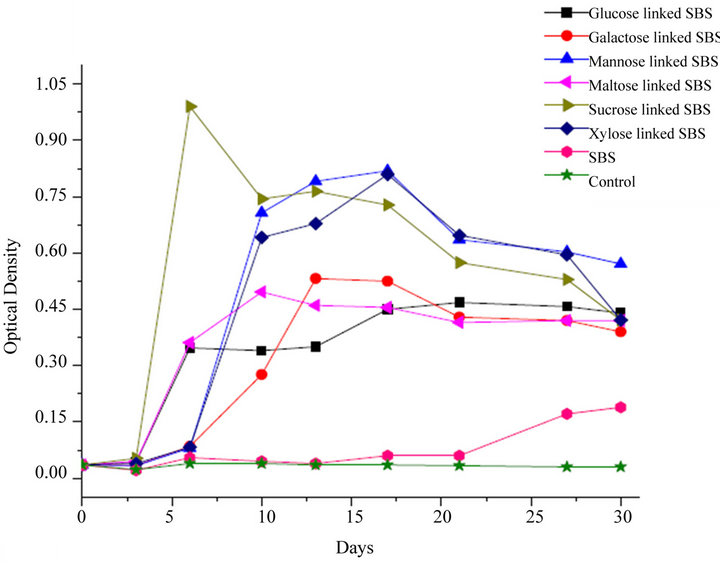
Figure 1. Growth pattern of Pseudomonas sp. on different sugars linked SBS.
From the growth pattern of Pseudomonas sp. on different monomeric sugars linked SBS, it can be observed that the rates of increase in optical density (reflecting material degradation) in sugars linked SBS is much higher than the unmodified SBS and the control. The galactose linked SBS showed OD maximum in two weeks, whereas the glucose and mannose liked SBS showed a maximum after two weeks. The mannose derivative showed significant increase in OD as compared to glucose and galactose linked SBS. However, it may be pointed out that the total weight loss after degradation is still insufficient to classify the modified SBS as a biodegradable material, even though the weight loss is several times the weight of the sugar attached. Therefore, though this strategy is promising, it needs another innovation to take it to the next level of biodegradation.
From the Figure 1, it is observed that the sucrose linked SBS was more degradable as compared to the maltose linked SBS. Sucrose linked SBS showed the highest OD among all the sugar linked SBS. For Pseudomonas sp. (NCIM 2220), the extent of biodegradation based on increase in optical density evaluated in the context of the extent of sugar incorporated, is as follows: sucrose > maltose > mannose > glucose > galactose > xylose.
This can also be discerned from the weight loss data presented in Tables 2 and 3 where the ratio of percent weight loss and percent sugar content of the sugar-linked SBS using bacterial and fungal cultures respectively. Due to the adherence of bacterial cells onto the polymer, the observed weight losses are generally lesser than expected. It is reported that in many such cases, weight loss data are inconclusive due to cell wall accumulation. From Table 2, we observe that SBS and partially epoxidized SBS without any sugar linked to it are not degraded by bacterial culture whereas the sugar linked SBS showed

Table 2. Weight loss data for bacterial degradation using Pseudomonas sp. over a period of 30 days.
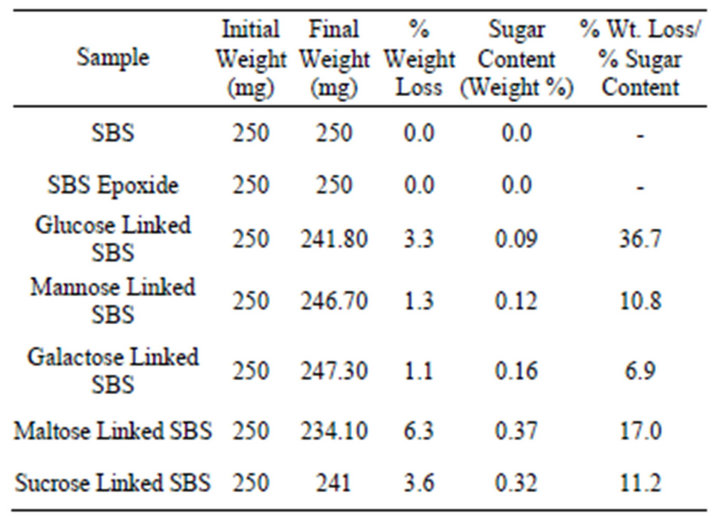
Table 3. Weight loss of the sugar-linked polymers degraded by fungal strain Aspergillus niger.
significant weight losses ranging from ~4 - 14 fold for the bacterial culture used in this study. This extent of weight loss is indicative of some polymer chain scission having also taken place along with the loss of sugar during biodegradation.
3.3. Weight Loss Data for Fungal Degradation of Sugar Linked SBS
Biodegradation of different sugar linked SBS was also studied with the fungal culture Aspergillus niger (NCIM 1025, ATCC 9642). Table 3 shows the weight data of the different sugar linked SBS after biodegradation. It is seen that weight loss varies with the type of sugar anchored and sugar content of the functionalized SBS. As in the case of bacterial biodegradation, here also the ratio of percent weight loss and percent sugar attached to SBS is as high as 36.7. SBS and epoxidized SBS having no sugar attached to them and was not degraded by the fungal culture.
The visual growth of the fungus (Aspergillus niger NCIM 1025) is seen from the photograph in Figure 2.
For Aspergillus niger (NCIM 1025, ATCC 9642) the extent of biodegradation based on weight loss evaluated in the context of the extent of sugar incorporated, is as follows: glucose > maltose > sucrose > mannose > galactose.
While the exact mechanism is still the subject of research, such findings have been reported before, with data proving reduction in molecular weight of the polystyrene polymer [10].
3.4. Scanning Electron Micrographs (SEM) of the Sugar Linked SBS after Degradation by Fungal Culture Aspergillus niger (NCIM 10,259)
The SEM of sugar linked SBS after biodegradation was recorded to investigate the morphological changes induced by biodegradation. Some representative samples were chosen in the form of films. After biodegradation the films were thoroughly washed with water to remove the adhering bacteria and fungus and were suspended in 70% ethanol and dried.
Figure 3 shows the morphology of the unmodified SBS and epoxidized SBS after biodegradation. There seems to be practically no change in the morphology of SBS and epoxidized SBS after exposure to the microorganisms. This is in accordance with the weight loss data where they do not show any weight loss indicating that they are not degraded by Aspergillus niger.