A Review of Main Factors Involved in the Maturation of Oil Palm (Elaeis guineensis Jacq.) Fruit Bunches ()
1. Introduction
Oil palm (Elaeis guineensis Jacq.) is a diploid perennial plant of the Arecaceae family. Currently, it is the most important crop in terms of oil production, which was estimated at about 72,940 (×1000 MT) in 2019 [1] . This huge oil production is mainly due to average high yields compared to other oil crops. Favourable soil and climatic conditions for flourishing are fulfilled with a temperature of 25˚C - 28˚C, sunshine of at least 5 hours per day, rainfall between 1800 - 2400 mm/year and no continuous drought for more than 90 days. Oil palm prefers rich, well-drained soils, but grows well in poor soils if fertilized [2] , low altitude, ideally below 500 m [2] . The oil palm ensures its survival through its fruits borne by the female inflorescence. The female inflorescence is located in the axils of the 17th to 20th leaves from the central stem. The fruits are contained in bunches. The production of palm bunches is influenced by several factors including: nutrients, water, carbohydrate supply and pollination [3] . Changes in any of these factors may affect the level of production of fruit bunches. Of these factors, pollination has the greatest influence on palm bunch production. Poor pollination or inefficient pollination activity, separately or in combination, will lead to a low production of bunches [3] . The weight of palm bunches increases with age, with an average of 5 kg on 3 years old palms, and more than 25 kg for palms over 15 years of age [4] . It is also relevant to notice that the number of bunches produced per year per palm decreases with age, with a constant average weight compensated by an increase of the weigh of bunches. Moreover, the weight of a bunch depends partly on the fruit set and thus also on the efficiency of pollination [5] . From pollination to the maturity of a bunch it takes about 4.5 to 6 months [5] . Several events occur during this timeframe: seed enlargement, weight increase, colour change, etc. All of these constitute bunch maturation factors that can provide a better understanding of the biology of the seed, the average duration of bunch maturation period, and the changes in oil content and can as well open up new possibilities for seed production using innovative methods that are faster and less costly, particularly the germination of seeds before they enter the dormancy phase. The aim of this work was therefore to identify the biochemical, physiological and Environmental parameters involved in the development and maturation of an oil palm bunch.
2. Anatomical and Physiological Level
2.1. Evolution of the Endosperm
The endosperm is the plant tissue where nutrient reserves in the seed of gymnosperms are stored. During the early phases of growth initiation in the oil palm, the endosperm is in a liquid form [6] . This liquid consistency corresponds to the coenocytic phase of the formation of this nuclear-like organ [7] [8] , which lasts until 56 Days after Pollination (DAP). After 70 DAP this tissue starts to become gelatinous. Several events take place in an increasing chronology during the process of endosperm development. Thus, the endosperm is present from 17 DAP. It is in a liquid form until 42 DAP. Between 42 and 70 DAP, it becomes gelatinous (Figure 1 and Figure 2). At 84 DAP, it is cartilaginous and by 126 DAP, it has already acquired the final solid consistency of a mature endosperm [9] . This evolution coincides with the cessation of growth of the diameter of the seed coat which is thought to be associated with the accumulation of lipid reserves [9] . A similar evolution is described by [10] , where two weeks after pollination, the endosperm retains its liquid form. At the beginning of the 8th Week after Pollination (WAP), it increases in size and retains its liquid form. At 10 WAP the endosperm becomes semi-gelatinous. At the 12th week, lipogenesis starts and is almost complete at the 16th WAP. After the 16th WAP the endosperm starts to solidify [9] [10] [11] .
2.2. Evolution of Seed Cavities
During the first 28 DAP, the average diameter of the seed coat does not exceed 1.80 mm [9] . It grows exponentially after 42 DAP and stabilizes after 98 days until full maturity at 168 DAP to reach 10.07 mm (Figure 3).
![]()
Figure 2. Three stages of oil palm bunches (virescens form): left is underipe, the center is ripe, right is overripe [13] .
![]()
Figure 3. Average seed growth parameters of E. guineensis var. Dura [9] .
2.3. Evolution of the Embryo
The differentiation of the embryo takes place between 60 and 70 DAP, a period during which the fruit develops to near maximum size [13] . Morphologically, it appears as a small cylindro-conical peg, which at maturity is about 3 mm long with a circumference of 1.2 mm, [9] [14] [15] , and embedded in the endosperm near the apex of the nucleus [16] . Full maturity arises between 70 and 80 DAP [5] and is followed by dormancy [17] .
2.4. Evolution of the Endocarp
The endocarp refers to the innermost layer of the pericarp, the tissue of the fruit covering the seed. Tissue development begins during the first 70 DAP. This results in an exponential increase in its thickness. In the early stages of seed development, this tissue exhibits a whitish color. During the ripening phase of the fruit, the dehydration of this tissue continues accompanied by its hardening and color change [18] , with an outflow of P, K, Ca, and Mg. This is done such that at the end of the development of the fruit of E. guineensis, the endocarp is completely sclerified and has minimum water content. This dehydration begins from 10 WAP and will continue until the maturity of the fruit. Through this evolution, the endocarp progressively isolates and thus protects the seed from the rest of the fruit.
2.5. Mesocarp Evolution
During the first 10 WAP, the growth of the parenchymal cells of the ovary wall is thought to be related to the presence of a large amount of water in this tissue [19] . Indeed, during this phase the mesocarp is continuously waterlogged and has a whitish color. Several physiological activities take place in this tissue, which would contribute to its elongation and stretching [18] [19] [20] , thus increasing its thickness. At this stage, lipids are practically non-existent. They start to be present from the 56th DAP, which would probably represent cellular and protoplasmic lipids contained in the mesocarp [19] . During this phase the mesocarp gradually turns yellow, which is indicative of the presence of lipids.
2.6. Change in Color of the Fruits
This is the most important parameter. Classification according to color is based on certain principles. Before maturity in “nigrescens” form (the most common) the fruit is dark black at the base and orange at the top, and black after maturity (Figure 4), whereas in “virescens” form the fruit is green before maturity and at
![]()
Figure 4. Fruit types based on fruit color. (a): detached fruits of the type Nigresens (Nig), (b): detached fruits of the type Virescens (Vir); (c): mature diets of both types [21] .
maturity the color becomes entirely orange-green [22] . One of the first observations indicating the maturity of a bunch is the color change noticed on the fruits [5] . This color change is due to the presence of certain pigments (anthocyanin) lodged in the mesocarp. The mesocarp becomes increasingly orange as carotenoids are synthesized. [23] reported a 5-fold increase in carotenoid content between 105 and 140 (DAP).
3. Increase in Seed Weight
Increase in seed weight is a function of seed development, and is due to cell divisions and rapid expansion accompanied by a gradual accumulation of reserves. [6] and [10] have provided more details describing the development of a seed. For these authors, the fruits develop in size and weight, up to the 100th DAP, and also in dry matter weight. The same observation is made for other species of the plant kingdom such as soybean and maize [24] . In addition to cell division, lipid accumulation in the seed, evolution of the mesocarp and endosperm are closely related to weight increase [10] [19] (Figure 5).
4. Fruit Ripening
Fruit Detachment from Bunches
This is characterized by the abscission of fruits from the bunch. It occurs first in the central part where the fruit is attached to the bunch. The mesocarp produces ethylene which stimulates cell wall degrading substances [6] . The fruits fall on the ground and signals the maturity of the bunch. The presence of 1 - 5 detached and/or easily detachable fruits is one of the generally accepted criteria for harvesting bunches. This phenomenon is more accentuated in the dry season than in the rainy season. In the rainy season, the bunches take longer time to reach maturity.
![]()
Figure 5. Evolution of mesocarp during E. guineensis Jacq. var. dura fruit ripening [19] .
5. Biochemical Level
5.1. Endocarp
The endocarp is the part of the seed that protects and allows the dispersion of the seed [11] . It protects the endosperm and the embryo from external shocks. In addition to protection, the endocarp plays various roles in the function of the fruit such as communication with the developing endosperm. It can be fleshy as in watermelon, fibrous as in mango, or extremely hard and durable as in a peach [11] . The endocarp consists mainly of lignin comparable to that of some hardwoods with lignin accounting for about 52% [12] .
5.1.1. Establishment of Carbohydrate Reserves
In oil palm, the accumulation of soluble sugars varies considerably during seed development [25] . High levels of hexoses (glucose and fructose) accumulate early in seed development. This level is due to the intense mitotic activity [26] . The high level of hexoses at the beginning of growth seems to be important for cell growth.
5.1.2. Establishment of Mineral Reserves
Generally, mineral elements are present in high level at the beginning of seed development. Most of the minerals studied showed a high level at the beginning of seed development [10] . This is the case for chloride, sulphate, nitrate and potassium which decreases in the seed during its development. [10] [27] showed that there was a decrease in these mineral reserves during seed development.
5.1.3. Evolution of Oil Content
During seed development, the endosperm is in a liquid form for the first 70 DAP. This organ will gradually change shape and colour during its development and will solidify as the seed approaches maturity. At the end, it is greyish-white in colour and surrounded by a fibre-covered testa strand, incorporating the embryo within it. Oil synthesis in the seed tissue takes place relatively early in the development of the seed [28] . It starts at about 120 DAP and continues until the fruit detaches from the bunch [28] , and is correlated with massive water loss [9] [13] . On the other hand, many studies have shown that the oil content in the mesocarp is correlated with the level of maturity [29] . Ripening of bunches is associated with a detachment of the fruits from the bunch and maximum oil content in the mesocarp [14] . During the fruit ripening stage, the endosperm continues to solidify probably due to lipid synthesis which lasts until 20 WAP and also to water loss [6] . This lipogenesis at the level of the endosperm, associated with strong dehydration and loss of potassium and calcium may contribute to increase the mass of this tissue and to solidify it [30] [31] . This development coincides with the cessation of growth of the diameter of the seed coat, and could be associated with the accumulation of lipid reserves in the mesocarp.
5.1.4. Evolution of Water Content
Water plays a vital role in this part of the fruit, particularly with the growth of parenchymal cells. During the 10 first weeks after pollination, the mesocarp is continuously waterlogged [19] . Indeed, the water in this tissue would participate in various physiological activities, in particular the elongation and stretching of cells [18] , and subsequently the exponential increase in its thickness. Lipogenesis would begin between 12 and 13 Week After Pollination (WAA), followed by extensive lipogenesis associated with strong water dehydration in this tissue [19] . The mesocarp then becomes full of oil but with a low water content when the fruit matures.
5.1.5. Development of Protein Reserves
Fruits with a hardened endocarp are usually drupes [11] . It protects the seed from diseases and herbivores [12] . The endocarp generally consists of a multi-layered sub pectin and protein arrangement [11] . The endocarp in the oil palm does not really have proteins, except in traces.
5.2. Mesocarp
5.2.1. Evolution of Oil Content
In the early stages of the development of this organ, the water content is very high. A timid lipogenesis starts at 84 DAP and continues until seed maturity [11] [14] [26] . In the immature state, the mesocarp contains a large amount of sterols. As maturity approaches these sterols decrease due to the huge amount of triglycerides synthesized [32] . However, one of the main resources for fruit development and lipid biosynthesis are the carbohydrates generated by biosynthesis in the leaves [26] . Lipid synthesis in the mesocarp occurs mainly through two distinct pathways: the first is the Kennedy pathway which relies on a sequential acylation process of fatty acids on a glycerol-3-phosphate scaffold provided by dihydroxyacetone phosphate dehydrogenase, while the second pathway relies on acyl exchange between the lipids and involves the phospholipid diacylglycerol acyltransferase. Diacylglycerol is the last intermediate before the formation of triacylglycerol in the endoplasmic reticulum. Between 18 and 20 DAP three types of fatty acids are synthesized C16:0, C18:0 and C18:1 [33] . Furthermore, the resulting oil yield characteristics of oil palm are an additive effect of several genes [15] [34] . For these authors, several other factors may be involved in palm oil yield: the influence of the environment and their interactions, and also the genetic origins of the palm populations.
5.2.2. Evolution of Water Content
During the first 70 DAP, the mesocarp has a high and stable water content, a total absence of oil and continuous transverse growth. Between the 70th and 120th DAP, there is significant water loss and oil enrichment [9] . For [35] , high water content in early seed development is important for metabolic activity and maintenance of seed growth. At the mature stage, water loss is probably due to the deposition of storage reserves (lipids, carbohydrates, proteins) that displace water from the cells [21] .
5.2.3. Development of Protein Reserves
Enzyme activity in developing oil palm fruit starts between 16 and 20 WAP, which corresponds to the beginning of palm oil formation in the mesocarp [25] . The most important enzyme in this phase is lipase. It is an important indicator of the onset of oil synthesis in the mesocarp, as its activity is linked to it. Many enzymes involved in oil biosynthesis, such as acetyl-CoA carboxylase (ACCase), acetyl-CoA: ACP transacylase, malonyl CoA: ACP transacylase, β-ketoasil-ACP synthetase, β-ketoasil-ACP reductase, β-hidroksiacyl-ACP dehydratase, enoylACP reductase, acyl-ACP thioesterase and other enzymes catalyze the formation of triacyl glycerol [21] . ACCase is one of those enzymes considered as key enzymes for oil biosynthesis. According to [28] , two proteins of the mesocarp increased considerably just before the period of the biosynthesis of oil at 12 WAP (Figure 6): The 34.3 kDa protein which present from 8 - 12 WAP at low concentration, but its expression then increases up to 15 WAA [28] . Another protein, with a 31.0 kDa MW, was also weakly expressed between 8 - 15 WAP, but more strongly at 17 WAP.
5.2.4. Lipase Activity
The acidity of palm oil is correlated with the maturity level of the fruit. In mature fruits, there is a high lipase activity in the mesocarp compared to fruits at the beginning of their development. This enzyme is responsible for the massive release of free fatty acids in the mesocarp of mature fruits. Free fatty acids (FFA) are commonly used as indicators of palm oil quality [36] . Lipase activity is observed in the mesocarp as from 16 WAP, and shows a progressive increase until the 20th WAP. Triglyceride synthesis in the mesocarp starts from the 16th WAP
![]()
Figure 6. Oil and protein content of oil palm mesocarp from different developmental stages (8 - 24 WAA) [37]
and reaches its peak at 21 weeks. Lipase activity thus appears to be correlated with lipid biosynthesis [22] . It is involved in the acidity of the oil produced, according to the chemical reaction below [38] :
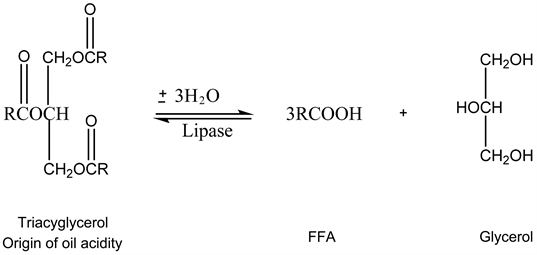
6. Influence of the Environment on Bunch Maturation Parameters
Influence of Temperature
Temperature is undoubtedly a very important factor for the maturation of bunches. [39] , showed that high temperatures would lead to an acceleration of the vegetative cycle and result in an earlier bud break and a higher growth rate. High temperatures generally influence fruit growth rates [40] . Higher temperatures lead to acute shortening of the duration of the development period of the reproductive organs in many species [41] . For [42] the results are different and vary depending on the species. For maize it would be around 30˚C (Figure 7).
![]()
Figure 7. Temperature response for maize and broccoli plants showing the lower, upper and optimum temperature limits for the vegetative growth phase [42] .
7. Conclusion
In the process of bunch ripening, some main criteria are involved notably color, fruit size, water content, oil content which are the most important. They allow a better understanding of the biology of the seed, its development and the different components that make up the pericarp (endocarp, mesocarp and endosperm). Thus, it takes about 168 DAP for a bunch to mature. In addition, during the development of the seed, a series of anatomical changes are observed amongst which are the change in color and the increase in size and weight. A detailed study of these parameters has not yet been done and the present study could open up new avenues for research into innovative, faster and low-cost methods of oil palm seed production particularly before seed dormancy. Also, future research works need to be undertaken to better understand the chronological order of formation of the organic molecules of the seed reserves. This will allow us to better understand their degradation rate during the germination process, and thus possibly increase the rate of germination. This may therefore open new avenues for research to better understand the physiology of oil palm germination.
Acknowledgements
The authors of this review would like to sincerely thank the UGAP (Unité de Génétique et d’Amélioration des Plantes) of the University of Yaoundé 1 and its supervisor for his support and guidance, Miss Lum Abienwi, all the researchers of the IRAD La Dibamba oil palm research station for their support.