The Effect of Mineral Composition, Microstructure on Physical Properties of Aggregate from River Punjkora and Kunai, Dir (Lower), Khyber Pakhtunkhwa, Pakistan ()
1. Introduction
Crushed rocks are chiefly produced and used as coarse aggregate in concrete and road construction. Typically aggregates are coarse type rock materials that are less than 40 mm and larger than 4 mm in size while the fine type consists of less than 4 mm (Bell, 2007). Aggregates are the major constituents of concrete typically occupying between 70% and 80% of its volume. Therefore, aggregates are expected to have an important influence on the concrete parameters (Thomas & Folliard, 2007). The selection of the coarse aggregate type in concrete is a dominant factor for the quality of concrete for the same cement quality, different litho-types of coarse aggregates characterized by different micro-roughness, mineralogical composition, structure, and compressive strength may result in different concrete compressive strength. Mineralogical composition of the aggregates, and more specifically their alteration degree, strongly influence their mechanical behavior and their in-service performance (Al-Oraimi, Taha, & Hassan, 2006; Yilmaz, Goktan, & Kibici, 2011; Petrounias et al., 2018). Increased percentages of certain secondary minerals negatively affect the physical, as well as the mechanical properties of aggregates due to their smooth layers, cleavage, and platy or fibrous crystal habit (Petrounias et al., 2018) which harm the performance of aggregates (Yilmaz, Goktan, & Kibici, 2011). Aggregate consists of rock fragments or grains that are quarried and used after crushing, sizing, washing, or in their natural state. Concrete, mortar, and asphalts are formed by the combination of sand, gravel, and crushed stone with binding media (Langer, 2003).
To better understand the nature of rocks with their microstructure (petrographic characteristics), some techniques were developed. They reported that micro-fractures, grain boundaries, mineral cleavages, and twinning planes influence the properties of rock and may act as surfaces of weakness, which control the direction in which failure occurs. Modal mineralogical composition, grain size, grain shape, the overall abundance of flaky minerals (mica), and more importantly the degree of their preferred orientation, and degree of weathering or alteration are geotechnically important parameters fine-grained rocks are generally stronger than coarse-grained ones. It is not only the grain size but also the grain size distribution that is important, and better resistance to fragmentation and wear compared to a more equigranular or idioblastic rock. Euhedral mineral grains may act as discontinuities where cracks may initiate in the structure (Lindqvist, Åkesson, & Malaga, 2007). Going from an idioblastic texture, in which the shape of the grains is largely defined by straight surfaces, to xenoblastic texture with more irregular grain boundaries, increases the resistance to fragmentation and degradation of aggregate (Åkesson et al., 2003).
Aggregates have been used in construction since the earliest period. The suitability of aggregates for their applications is determined by evaluating their physical, physicochemical, and microstructural properties, which are collectively known as engineering properties. The engineering properties of rocks mainly depend on their mineralogical composition, textural characteristics, chemical alteration degree, weathering, and deformation (Irfan, 1996; Youssef, 2015; Giannakopoulou et al., 2018). (Shakoor & Bonelli, 1991) determined that water absorption is a useful property for the suitability of aggregate. The greater the content of water absorbed; the greater will be the loss in strength of the rock. (Blyth & De Freitas, 1974) reported that low water absorption value, in turn, means that the given rock has high resistance to weathering. Such rocks have a very low disintegration effect from frost action and chemical weathering the suitable specific gravity of rocks for heavy construction is ≥2.55 (Blyth & De Freitas, 1974). The specific gravity capacity of coarse aggregate is a key parameter in the design and construction of any structure using aggregates (Mills-Beale & You, 2010). Abrams (1925) introduced the idea of fineness modulus for the assessment of the ratio of fine and coarse aggregates.
The purpose of this study was to investigate the relationship between petrographic characteristics and engineering properties of aggregates derived by river Panjkora and Kunai near Timergara city in lower Dir (L) district, KP, Pakistan. Geologically the study area constitutes a part of the Kohistan Island Arc (KIA) which lies between latitude 34˚51'59.1"N and longitude 71˚52'48.0"E of River Panjkora and latitude 34˚54'32.5"N and longitude 71˚49'48.3"E of River Kunai. The main Malakand-Dir-Chitral Road passes through the area. Both the River Panjkora and Kunai contain sand to boulder size particles of various lithologies in the form of mid-channel bar, point bar, and river bed sediments. A preliminary petrographic account of the rocks around the study area has been presented by (Chaudhry & Chaudhry, 1974).
During the field, certain lithologies have been identified in hand specimens like amphibolites, granites, volcanic, and gneiss, while few were difficult to identify in hand specimens due to their weathered nature. The engineering properties, which were determined in the course of the present study, include specific gravity and water absorption Loss Angles abrasion (LA), Fines Modulus (FM), and soundness. These properties were analyzed to know the response of the investigated rocks when subjected to load thereby assessing their suitability for construction material and seeing the possible relationship between them and petrographic details.
2. Geology and Tectonic
The northern part of Pakistan consists of the Eurasian plate in the north, Kohistan Island Arc (KIA) in the middle, and the Indo-Pakistan plate in the south. The area under investigation is part of Kohistan Island Arc which is bounded to the north by Shyok suture zone or MKT (Main Karakoram Thrust) and to the south by Indus suture zone or MMT (Main Mantle Thrust) (Tahirkheli, 1979). The entire Kohistan sequence represents the crust and mantle of a complete island arc. Therefore, from south to north, the KIA is composed of six tectonic stratigraphic units including Jijal Complex, Kamila amphibolites, Chilas complex, Kohistan batholith, and Gilgit gneisses Chalt, volcanic, and Yasin group meta-sediments (Khan et al., 1997).
A volcano-sedimentary sequence is present around Dir and Swat areas known as the Dir group is divided into further three lithologic units i.e., Barawal Banda Slates, Dir-Utror volcanic series, and the Panakot meta-arkose (Tahirkheli, 1979, 1982). Dir group show mostly porphyritic texture having plagioclase as a phenocryst, epidote and quartz veins are also present in these rocks. Baraul banda slate consists of slate, shale, wakes, calcareous beds, and some volcanic rocks (Khan, 1979; Sullivan et al., 1993).
Microfossils in the Baraul Banda slate are early Eocene in age (Tahirkheli, 1979, 1982). The Panakot meta-arkose forms a thin band < 1 km wide which separates volcanic rocks of the Dir-Utror volcanic suite from the Lowari quartz diorite. Utror Volcanic Group is mainly consisting of silicic to intermediate lavas, tuffs, ignimbrites, and agglomerates. The thickness of this unit near Utror village ranges from 5 - 8 km and consists of relatively schistose, meta-andesitic agglomerates or lavas and metadacites. Plutonic rocks mainly granites and quartz diorite are intruded in the metamorphic and sedimentary rock of this unit. Small stocks and sills of coarse-grained granodiorite and diorite intrude the metavolcanic sequence (Shah & Shervais, 1999).
One of the main lithologies which these two rivers drain is the Khagram amphibolites which represent the western extension of Kamila Amphibolite. (Sajid, Arif, & Muhammad, 2009) divided the Khagram Amphibolites into epidote amphibolite, hornblendite, and gabbro-norite. Epidote amphibolite consists of small to large patches of gabbro-norite and Hornblendites. In the field, they are easily distinguishable from the surrounding epidote amphibolite by their dark black color while Kumrat granite represents stage-2 plutons of Kohistan batholith which were intruded in Dir-Utror volcanics and Sharman volcanic, represent Cenozoic magmatism in the KIA (Sullivan et al., 1993; Treloar et al., 1996).
The study area is located in the southwest part of the KIA, northwestern Himalaya, Pakistan. The samples are taken from crushed plants and river floats of the Panjkora and Kunai River. The present work is mainly focused on the petrographic and physical analysis of aggregates along rivers Panjkora and Kunai. These rivers dissect the northwestern KIA from north to south and therefore, contain different floats of the northwestern KIA (Figure 1).
3. Material and Method
The present study has focused on river-derived aggregates of Panjkora and Kunai
for their suitability as aggregate in construction purposes. The Panjkora river starts from the kumrat and Barawal valleys while the Kunai River starts from the maidan area which meets each other near Odigram just five km north of timargara city.
3.1. Petrography
For petrographic analysis, 22 bulk samples including grab samples were collected from River Panjkora and Kunai. These samples mostly consist of granitic rocks (35% by volume), gabbroic rocks (24% by volume), amphibolite (12% by volume), ultramafic (12% by volume), volcanic (12% by volume), and gneiss (5% by volume) as shown in Chart 1. In hand specimen, granitic rocks were fine to medium-grained while gabbroic rocks were medium-grained. For microscopic studies, eighteen bulk samples were cut into thin sections and polished with abrasive powder to a smoothness level to visible all the features in microscopic viewing. The detailed petrographic analysis was analyzed by a petrographic microscope with an equipped digital camera to record the important features and
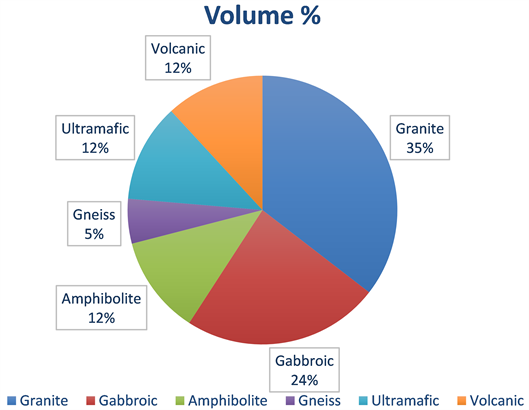
Chart 1. Volume (%) of studied rocks samples.
properties. The analysis was aimed to determine the modal mineralogy, textural relationships, and possible origin of the rock.
3.2. Physical Properties
3.2.1. Water Absorption
At first, the rock sample was put in water for 24 hours, and vanished the shining surface with a towel to take the SSD (surface saturated dry) weight. This sample was kept in the oven for 24 hours at 110˚ C and the oven-dry weight was recorded. The absorbed water was calculated by the formula as shown in Equation (1).
(1)
W1 = Dry weight of rock sample (g)
W2 = SSD weight (g)
3.2.2. Specific Gravity
Specific gravity, Saturated Surface Dry (SSD), dry specific gravity, and apparent specific gravity were determined in the laboratory for both river panjkora and kunai. The rock samples were placed in the oven for 24 hours at 110˚C and then taken out to cool. Each of the rock samples is then weighted as dry weight (W1). All the rock sample is then placed in water for 24 hours, then for the surface saturated dry (SSD) weight all the specimens are removed from the water and then vanished the shining surface by towel and calculated its weight as W2. After that, leave the sample to dry and then weigh in water as W3. Test were conducted through the standard method of ASTM (ASTM C127, 2001).
3.2.3. Loss Angeles (LA)
Resistance to grinding is determined by the LA test machine. This test is used to determine the toughness and abrasion properties of aggregate. In this study, a coarse aggregate was subjected to an abrasion machine containing 12 steel balls. After the rotation of the drum, the retained weight is deduced from the original weight to obtain the total percentage of weight that has broken down and pass-through the No. 12 sieve. Sample preparation and test are conducted through the standard method (ASTM, 2014). Results of each sample are listed in Table 3.
3.2.4. Fineness Modulus (FM)
Fine aggregate is placed in the oven for 24 hours and then take the diagonals and pass-through sieve No. 4 and the retained weight was taken. FM is the total percentage retained on each specified sieve divided by 100. The upper and lower limit of the specified sieves is the No. 4 and No. 100 sieve respectively is shown. The size for calculating FM of fine aggregate is No. 4, 8, 16, 30, 50, and 100. (ASTM C33, 2010) “standard specification for concrete aggregates” shows the requirements for the FM of fine aggregate. ASTM C-33 requires the FM of fine aggregate to be between 2.3 and 3.1.
3.2.5. Soundness
The group of samples was immersed in the prepared solution for 18 hours in such a way that the solution covered them to a depth of nearly one and a half inches. The containers were covered to stop vaporization and prevent the addition of the outside substances. Fraction of samples was immersed in the mixture at a temperature of 21˚C. After eighteen hours the aggregate sample was removed from the solvent. It was then dried for fifteen minutes and in a drying oven at a temperature of 110˚C for six hours and cooled at room temperature. In this way, the first cycle was completed. Similarly, five cycles were completed. After completion of the final cycle, the sample is cooled and washed free from sodium sulfate by reaction of wash water with barium chloride (BaCl 2). At 43˚C the sample was washed by circulating water in the container. This may be done by introducing water near the bottom and allowed to overflow. During washing operation, the sample should not be placed to impact or abrasion which may break up the particles. Each group of samples dried after removal of sodium sulfate to a constant weight at 110˚C. The weight of each friction of the sample retained on the sieve was recorded. Loss in each friction is expressed as a percentage of its initial weight.
The value of material loss depends on which type of soundness test is used. According to the standard method the value ranges from 0% - 15% for sodium sulfate while 0% - 30% is thefor magnesium sulfate. Sample preparation and test are conducted by the standard method (ASTM, 2014).
4. Results and Discussion
The river Panjkora (RP) and the river Kunai (KK) samples fall in the category of granite (Figure 2). The thin section study shows that granite is texturally in-equigranular, fine to medium-grained, and has no preferred orientation. Quartz, plagioclase, alkali feldspar, and biotite are dominant mineral phases in rock (Table 1; Plate 1). The plagioclase and alkali feldspar has undergone alteration and plagioclase has converted to clays. The plagioclase has been undergone sericitization and also displays normal zoning (Plate 1(A)). The quartz ranges in size from very fine to medium grain and is fractured and deformed. Hornblende, biotite, epidote, garnet, muscovite, and ore minerals occur as minor to accessory minerals (Table 1). The rock is fractured and epidotes and sericite have developed along with these fractures.
Among the gabbroic rocks, all the samples of the river Kunai spread over the field of pyroxene-hornblende gabbro/norite. However, one sample of the river Panjkora (RP-4) falls with the river Panjkora samples in the pyroxene-hornblende gabbro/norite, while, one sample (RP-14) due to a high amount of pyroxene falls in the field of plagioclase bearing hornblende-pyroxenite (Figure 3). The gabbroic rocks are texturally in-equigranular, containing anhedral to subhedral grains, and are medium-grained. The essential minerals in gabbroic rock are hornblende, pyroxene and plagioclase (Figure 4(B)). The pyroxene mainly includes clinopyroxenes and exhibits a prominent simple twining (Table 1; Plates 2(A)-(C)).
![]()
Table 1. Model mineralogy of the studied samples in thin section.
Qtz = Quartz; A. F = Alkali Feldspar; Plg = Plagioclase; Hbl = Hornblende; Pxn = Pyroxene; Bt = Biotite; Epi = Epidote; Rut = Rutile; Zr = Zircon; Sph = Sphene; Gt = Garnet.
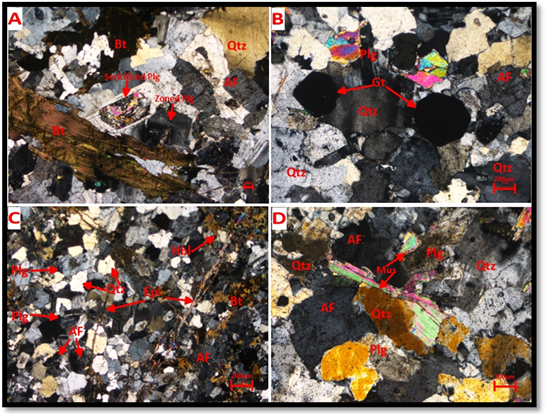
Plate 1. Photomicrographs of granites (A) showing zoned and sericitized plagioclase along with the large grain of biotite, (B) showing deformed quartz and garnet, (C) showing fractured grain, development of epidote and sericite along the fracture, (D) showing plagioclase, quartz, alkali feldspar and muscovite.
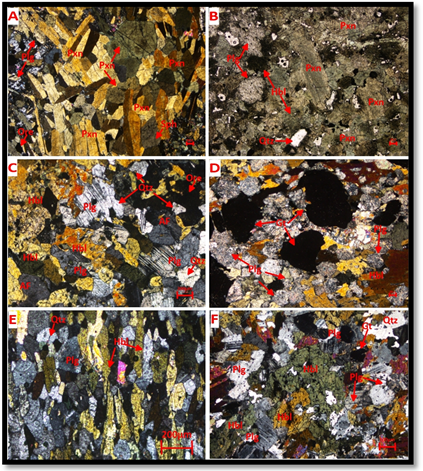
Plate 2. Photomicrographs (A, B, and C are of gabbroic rock while D, E, and F for amphibolite) (A) show hornblende, plagioclase, and undolose extinction in quartz in amphibolite. (C, PPL; D, XPL) showing garnet of high fracture and ore.
![]()
Figure 4. Model mineralogical analysis for all thin sections (A) amphibolite and hornblende, (B) gabbroic, (C) granite, (D) all thin sections.
Plagioclase show typical polysynthetic twinning and in places it has been sericitized (Plate 2(C)). Ore mineral, clays, and epidote occur as accessory minerals (Table 1).
The amphibolite is medium to coarse-grained and dominantly composed of hornblende and plagioclase. Quartz and alkali feldspar is present in a minor amount. Hornblende has altered into chlorite and plagioclase into clays. A slight fabric has been developed in the amphibolite due to which the hornblende grains show proper orientation (Plate 2(E)). Accessory minerals include alkali feldspar, garnet, sphene, chlorite, clays, and ore minerals (Table 1; Figure 3; Plates 2(D)-(F))
The petrographic analysis indicates different types of Igneous and Metamorphic rocks in the river floats of Kunai and Panjkora rivers. Based on petrography these rocks are identified as Granite, Gabbroics, and Amphibolite (Figures 4(A)-(C)). The volume of granite in the studied sample is 35% while gabbroic rock is 24%. Amphibolite, ultramafic, volcanic and gneissose rocks are 12% and 5% respectively by volume which is shown in Chart 1.
Generally, the alteration product of plutonic rocks has high clay content and their abundance has a deleterious effect on the durability aspects of a rock (Lindqvist, Åkesson, & Malaga, 2007). In this review, petrographic studies of Granitic rocks show that Quartz grains are strained and show wavy extinction which might be highly reactive in high alkaline aggregate. In the investigated samples, the feldspars in granites have altered to clays and sericite, which will ultimately give low strength to the rock strength. Alkali Feldspar grains are subhedral to euhedral, sub-equigranular to in-equigranular (Plate 1).
Metamorphic rocks are characterized by certain textures that have a marked preferred orientation which is appreciably stronger across than along the lineation but on the other hand shape-preferred orientation may form planes of weakness in a structure (Bell, 2007). A foliation defined by micas or clay minerals has a stronger influence on the properties than foliations without micas. Randomly oriented micas have a considerably less significant effect. This is because micas act as a large discontinuity in the foliation where cracks can initiate and propagate easily. The total amount of mica in rock is in itself not as important as foliations defined by micas (Lindqvist, Åkesson, & Malaga, 2007).
Mineralogically, Amphibolite consists of hornblende; plagioclase, quartz, and minor to the accessory amount of muscovite and are texturally sub-equigranular. Plagioclase-bearing pyroxenite is highly fractured (Plate 2) which indicates the ultimate weakness of aggregate. Gneiss consists of hornblende, quartz, feldspar, and biotite and is texturally in-equigranular, and holocrystalline. The gabbroic rock consists of hornblende, pyroxene and plagioclase. Quartz, rutile, clay content, epidote, and ore minerals occur as minor to accessory minerals (Figures 4(A)-(D)). The texture of the gabbroic rock is in-equigranular while the grains are anhedral to subhedral and medium-grained. The ultramafic rock consists of clinopyroxene and orthopyroxene which are highly fractured. The grains of clinopyroxene are medium to coarse while that of orthopyroxene are fine to medium (Plate 2(A), Plate 2(B)).
Rocks containing large amounts of physically competent minerals are strong and durable. Similarly, rocks with finer grain size are stronger than those with similar modal mineralogy but coarser grain size. Furthermore, rocks whose constituent mineral grains are irregularly shaped are likely to be stronger than otherwise similar ones but composed of grains with a regular shape (Arif et al., 2013). The irregular orientation of micas provides a strong significant effect on strength (Lindqvist, Åkesson, & Malaga, 2007).
The specific gravity and water absorption capacity of coarse aggregate are key parameters in the design and construction of any structure using aggregates (You, Adhikari, & Kutay, 2009; Mills-Beale & You, 2010). Result for the Specific gravity (RP 2.90 and RK 3.01) and water absorption (RP 0.67% and RK 0.73%) shown in (Table 2, Table 3). Such values are considered to be most durable and suitable for selecting a good quality aggregate. A small difference was founded between the values of both rivers but the results of the studied samples suggest that specific gravity and water absorption values of both sites are within range of
![]()
Table 2. Details and results of the Loss Angeles abrasion test.
![]()
Table 3. Results for specific gravity and Fineness modulus.
ASTM (Table 5). Therefore, the current hypothesis failed to reject the use of aggregate for various construction. In the investigated samples results for WA & SG of RP are less than RK which shows alternate strongness and more durability. Petrographic studies of RK show more minerals of biotite, alkali feldspar, and ore minerals which ultimately decrease the strength properties of aggregate. Most rocks samples show the same age and igneous activities but the difference is due to the degree of weathering and alteration.
According to the Loss Angles abrasion test result, the aggregate of both rivers showed high resistance to abrasion. LA test result is 19.2% for River Panjkora and 21.4% for Kunai River as shown in (Table 2). It can be observed that RP has less value of LA than RK which shows more abrasion and durability properties. The mineralogical study of river punjkora (RP) can RP3, RP4, RP7, RP9, RP10, RP11, RP13 (Figure 4(C)) are quartz rich which show more durability and resistance to abrasion. The LA results for both rivers are according to the ASTM standard (Table 5). According to these results, aggregate is used for concrete and road filler, especially where traffic is high and abrasive factors are numerous.
Increase of Fine Aggregate Fineness Modulus (FAFM) along with the increase in Concrete Aggregate Fineness Modulus (CAFM), concrete compressive strength increases in a significant amount (Donza, Cabrera, & Irassar, 2002). Generally, concrete strength increases with the increase of aggregate fineness modulus (Gauhar et al., 2016). Laboratory base results for both rivers show fineness modulus in Table 4. According to ASTM C-33 requires the FM of fine aggregate to be between 2.3 and 3.1. Results of fineness modulus for RK come within the range of ASTM which shows suitability for a good quality aggregate in concrete. In Figure 5 result for RP shows less value of FM than ASTM standard which ultimately decreases the strength of aggregate.
Soundness test is an indirect approach by which aggregate shows resistance to disintegration/breakdown when subjected to alternate freezing and thawing action occurs in pores water of aggregate. Soundness of aggregate depend on mineral mineralogy, texture, grain size, granular bonding and, porosity of aggregates (Hartley, 1974; Dolar-Mantuani, 1978). In the current study soundness results
![]()
Table 4. Soundness of river Kunai and Panjkora.
![]()
Figure 5. Results of the fineness modulus of aggregate.
are 3.5 for River Kunai while 4.4 for River Panjkora. In relation to both rivers it can be confirmed that River Punjkora has more loss in weight as compare to River Kunai due to the high value of water absorption and more porosity (Table 4)
![]()
Table 5. Details and results of various physical tests
but according to the ASTM standard both the values are within the range where it shows the suitability for any construction purposes as shown in (Table 5) (ASTM C88/C88M-18, 2018). Results of both rivers reveal that aggregate can be used as a construction material, especially where wetting and drying are too much especially where the temperature changing abruptly and continually. Aggregates from both rivers can be used for concrete inside water zones and glacial areas due to the strong resistance for water immersion, frost wedging and chemical alteration.
5. Conclusion
This study shows that the river float contains various amounts of Igneous (both intrusive and extrusive) and Metamorphic rocks. The Quartz of the granitic rocks and hence some other metamorphic rock shows deformed nature alteration while most of them are strong and show competent mineralogy. The microstructures and altered minerals are the main agents for affecting aggregate strength. However, the experimental results show that the tested rocks are suitable for raw material and construction projects. In addition, it can also be confirmed that a river-derived aggregate can be used for any engineering purposes and construction project. Many researchers have worked on aggregate from a specific rock or queries of the same lithology but a very little amount of data is available on river-derived aggregate which contains a mixture of complex lithologies. Due to less amount of data, the current study is lack finding the mechanical strength of aggregate in cement and asphalt. In heavy construction the strength tests are of high importance, therefore, various strength tests like uniaxial compressive strength test (UCS), uniaxial/splitting tensile strength test (UTS), and flexural strength tests are recommended for fracture use as coarse aggregate in concrete. From the mineralogical study, it can be concluded that some minerals can prone to the potential of alkali-silica reaction (ASR), thus a detailed alkali-silica reactivity (ASR) study is required to evaluate their nature as reactive silica in concrete.
Acknowledgements
Department of Geology, Shaheed Benazir Bhutto University Sharingal provided facilities for carrying out field and petrographic studies. Bulk samples were cut and thin sections are prepared in the rock cutting laboratory Department of Geology, University of Peshawar, Pakistan. For mechanical properties, tests of aggregate are conducted in the Geotechnical laboratory of the NCE in Geology, University of Peshawar, Pakistan.