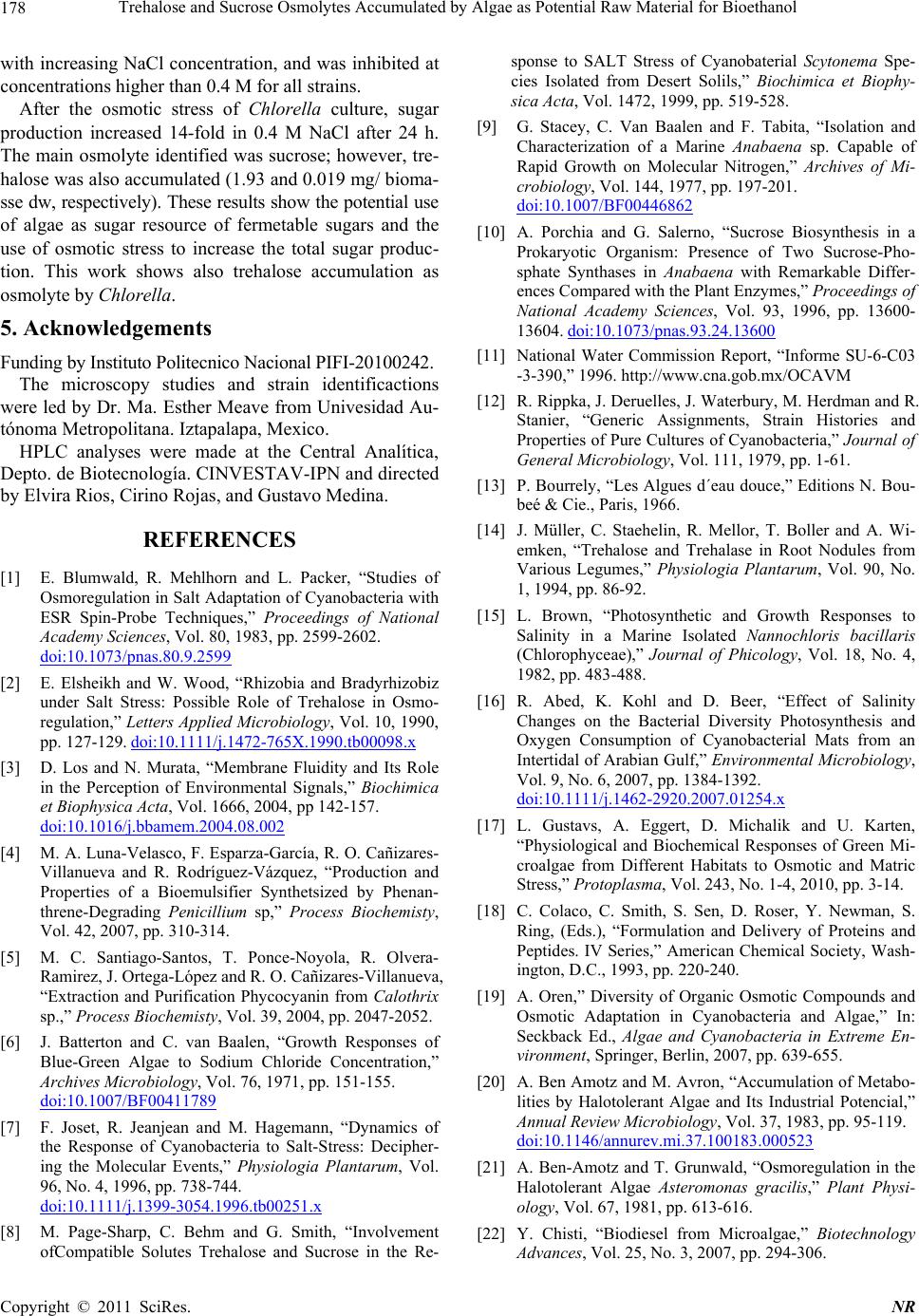
Trehalose and Sucrose Osmolytes Accumulated by Algae as Potential Raw Material for Bioethanol
178
with increasing NaCl concentration, and was inhibited at
concentrations higher than 0.4 M for all strains.
After the osmotic stress of Chlorella culture, sugar
production increased 14-fold in 0.4 M NaCl after 24 h.
The main osmolyte identified was sucrose; however, tre-
halose was also accumulated (1.93 and 0.019 mg/ bioma-
sse dw, respectively). These results show the potential use
of algae as sugar resource of fermetable sugars and the
use of osmotic stress to increase the total sugar produc-
tion. This work shows also trehalose accumulation as
osmolyte by Chlorella.
5. Acknowledgements
Funding by Instituto Politecnico Nacional PIFI-20100242.
The microscopy studies and strain identificactions
were led by Dr. Ma. Esther Meave from Univesidad Au-
tónoma Metropolitana. Iztapalapa, Mexico.
HPLC analyses were made at the Central Analítica,
Depto. de Biotecnología. CINVESTAV-IPN and directed
by Elvira Rios, Cirino Rojas, and Gustavo Medina.
REFERENCES
[1] E. Blumwald, R. Mehlhorn and L. Packer, “Studies of
Osmoregulation in Salt Adaptation of Cyanobacteria with
ESR Spin-Probe Techniques,” Proceedings of National
Academy Sciences, Vol. 80, 1983, pp. 2599-2602.
doi:10.1073/pnas.80.9.2599
[2] E. Elsheikh and W. Wood, “Rhizobia and Bradyrhizobiz
under Salt Stress: Possible Role of Trehalose in Osmo-
regulation,” Letters Applied Microbiology, Vol. 10, 1990,
pp. 127-129. doi:10.1111/j.1472-765X.1990.tb00098.x
[3] D. Los and N. Murata, “Membrane Fluidity and Its Role
in the Perception of Environmental Signals,” Biochimica
et Biophysica Acta, Vol. 1666, 2004, pp 142-157.
doi:10.1016/j.bbamem.2004.08.002
[4] M. A. Luna-Velasco, F. Esparza-García, R. O. Cañizares-
Villanueva and R. Rodríguez-Vázquez, “Production and
Properties of a Bioemulsifier Synthetsized by Phenan-
threne-Degrading Penicillium sp,” Process Biochemisty,
Vol. 42, 2007, pp. 310-314.
[5] M. C. Santiago-Santos, T. Ponce-Noyola, R. Olvera-
Ramirez, J. Ortega-López and R. O. Cañizares-Villanueva,
“Extraction and Purification Phycocyanin from Calothrix
sp.,” Process Biochemisty, Vol. 39, 2004, pp. 2047-2052.
[6] J. Batterton and C. van Baalen, “Growth Responses of
Blue-Green Algae to Sodium Chloride Concentration,”
Archives Microbiology, Vol. 76, 1971, pp. 151-155.
doi:10.1007/BF00411789
[7] F. Joset, R. Jeanjean and M. Hagemann, “Dynamics of
the Response of Cyanobacteria to Salt-Stress: Decipher-
ing the Molecular Events,” Physiologia Plantarum, Vol.
96, No. 4, 1996, pp. 738-744.
doi:10.1111/j.1399-3054.1996.tb00251.x
[8] M. Page-Sharp, C. Behm and G. Smith, “Involvement
ofCompatible Solutes Trehalose and Sucrose in the Re-
sponse to SALT Stress of Cyanobaterial Scytonema Spe-
cies Isolated from Desert Solils,” Biochimica et Biophy-
sica Acta, Vol. 1472, 1999, pp. 519-528.
[9] G. Stacey, C. Van Baalen and F. Tabita, “Isolation and
Characterization of a Marine Anabaena sp. Capable of
Rapid Growth on Molecular Nitrogen,” Archives of Mi-
crobiology, Vol. 144, 1977, pp. 197-201.
doi:10.1007/BF00446862
[10] A. Porchia and G. Salerno, “Sucrose Biosynthesis in a
Prokaryotic Organism: Presence of Two Sucrose-Pho-
sphate Synthases in Anabaena with Remarkable Differ-
ences Compared with the Plant Enzymes,” Proceedings of
National Academy Sciences, Vol. 93, 1996, pp. 13600-
13604. doi:10.1073/pnas.93.24.13600
[11] National Water Commission Report, “Informe SU-6-C03
-3-390,” 1996. http://www.cna.gob.mx/OCAVM
[12] R. Rippka, J. Deruelles, J. Waterbury, M. Herdman and R.
Stanier, “Generic Assignments, Strain Histories and
Properties of Pure Cultures of Cyanobacteria,” Journal of
General Microbiology, Vol. 111, 1979, pp. 1-61.
[13] P. Bourrely, “Les Algues d´eau douce,” Editions N. Bou-
beé & Cie., Paris, 1966.
[14] J. Müller, C. Staehelin, R. Mellor, T. Boller and A. Wi-
emken, “Trehalose and Trehalase in Root Nodules from
Various Legumes,” Physiologia Plantarum, Vol. 90, No.
1, 1994, pp. 86-92.
[15] L. Brown, “Photosynthetic and Growth Responses to
Salinity in a Marine Isolated Nannochloris bacillaris
(Chlorophyceae),” Journal of Phicology, Vol. 18, No. 4,
1982, pp. 483-488.
[16] R. Abed, K. Kohl and D. Beer, “Effect of Salinity
Changes on the Bacterial Diversity Photosynthesis and
Oxygen Consumption of Cyanobacterial Mats from an
Intertidal of Arabian Gulf,” Environmental Microbiology,
Vol. 9, No. 6, 2007, pp. 1384-1392.
doi:10.1111/j.1462-2920.2007.01254.x
[17] L. Gustavs, A. Eggert, D. Michalik and U. Karten,
“Physiological and Biochemical Responses of Green Mi-
croalgae from Different Habitats to Osmotic and Matric
Stress,” Protoplasma, Vol. 243, No. 1-4, 2010, pp. 3-14.
[18] C. Colaco, C. Smith, S. Sen, D. Roser, Y. Newman, S.
Ring, (Eds.), “Formulation and Delivery of Proteins and
Peptides. IV Series,” American Chemical Society, Wash-
ington, D.C., 1993, pp. 220-240.
[19] A. Oren,” Diversity of Organic Osmotic Compounds and
Osmotic Adaptation in Cyanobacteria and Algae,” In:
Seckback Ed., Algae and Cyanobacteria in Extreme En-
vironment, Springer, Berlin, 2007, pp. 639-655.
[20] A. Ben Amotz and M. Avron, “Accumulation of Metabo-
lities by Halotolerant Algae and Its Industrial Potencial,”
Annual Review Microbi ol ogy, Vol. 37, 1983, pp. 95-119.
doi:10.1146/annurev.mi.37.100183.000523
[21] A. Ben-Amotz and T. Grunwald, “Osmoregulation in the
Halotolerant Algae Asteromonas gracilis,” Plant Physi-
ology, Vol. 67, 1981, pp. 613-616.
[22] Y. Chisti, “Biodiesel from Microalgae,” Biotechnology
Advances, Vol. 25, No. 3, 2007, pp. 294-306.
Copyright © 2011 SciRes. NR