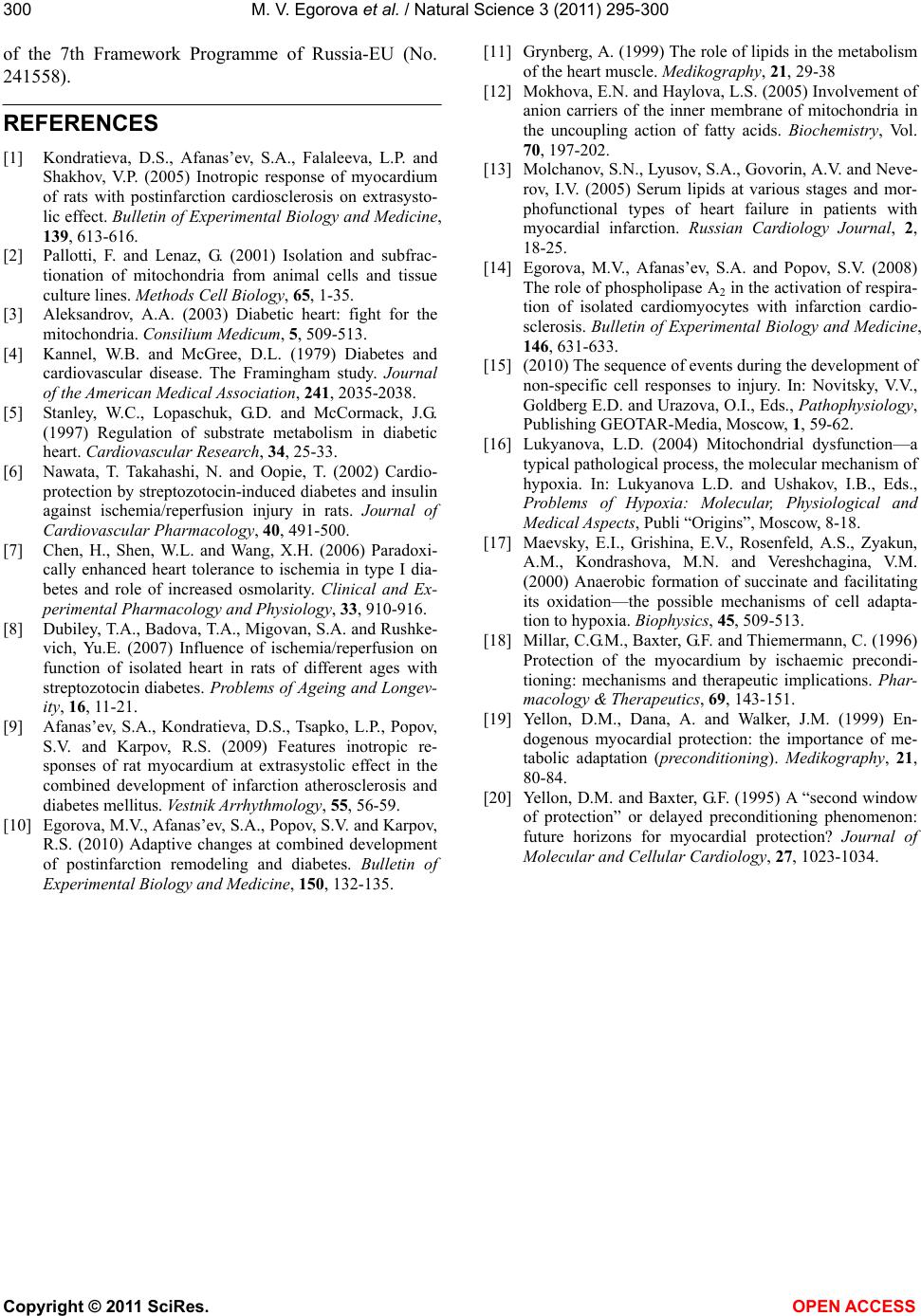
M. V. Egorova et al. / Natural Science 3 (2011) 295-300
Copyright © 2011 SciRes. OPEN ACCESS
300
of the 7th Framework Programme of Russia-EU (No.
241558).
REFERENCES
[1] Kondratieva, D.S., Afanas’ev, S.A., Falaleeva, L.P. and
Shakhov, V.P. (2005) Inotropic response of myocardium
of rats with postinfarction cardiosclerosis on extrasysto-
lic effect. Bulletin of Experimental Biology and Medicine,
139, 613-616.
[2] Pallotti, F. and Lenaz, G. (2001) Isolation and subfrac-
tionation of mitochondria from animal cells and tissue
culture lines. Methods Cell Biology, 65, 1-35.
[3] Aleksandrov, A.A. (2003) Diabetic heart: fight for the
mitochondria. Consilium Medicum, 5, 509-513.
[4] Kannel, W.B. and McGree, D.L. (1979) Diabetes and
cardiovascular disease. The Framingham study. Journal
of the American Medical Association, 241, 2035-2038.
[5] Stanley, W.C., Lopaschuk, G.D. and McCormack, J.G.
(1997) Regulation of substrate metabolism in diabetic
heart. Cardiovascular Research, 34, 25-33.
[6] Nawata, T. Takahashi, N. and Oopie, T. (2002) Cardio-
protection by streptozotocin-induced diabetes and insulin
against ischemia/reperfusion injury in rats. Journal of
Cardiovascular Pharmacology, 40, 491-500.
[7] Chen, H., Shen, W.L. and Wang, X.H. (2006) Paradoxi-
cally enhanced heart tolerance to ischemia in type I dia-
betes and role of increased osmolarity. Clinical and Ex-
perimental Pharmacology and Physiology, 33, 910-916.
[8] Dubiley, T.A., Badova, T.A., Migovan, S.A. and Rushke-
vich, Yu.E. (2007) Influence of ischemia/reperfusion on
function of isolated heart in rats of different ages with
streptozotocin diabetes. Problems of Ageing and Longev-
ity, 16, 11-21.
[9] Afanas’ev, S.A., Kondratieva, D.S., Tsapko, L.P., Popov,
S.V. and Karpov, R.S. (2009) Features inotropic re-
sponses of rat myocardium at extrasystolic effect in the
combined development of infarction atherosclerosis and
diabetes mellitus. Vestnik Arrhythmology, 55, 56-59.
[10] Egorova, M.V., Afanas’ev, S.A., Popov, S.V. and Karpov,
R.S. (2010) Adaptive changes at combined development
of postinfarction remodeling and diabetes. Bulletin of
Experimental Biology and Medicine, 150, 132-135.
[11] Grynberg, A. (1999) The role of lipids in the metabolism
of the heart muscle. Medikography, 21, 29-38
[12] Mokhova, E.N. and Haylova, L.S. (2005) Involvement of
anion carriers of the inner membrane of mitochondria in
the uncoupling action of fatty acids. Biochemistry, Vol.
70, 197-202.
[13] Molchanov, S.N., Lyusov, S.A., Govorin, A.V. and Neve-
rov, I.V. (2005) Serum lipids at various stages and mor-
phofunctional types of heart failure in patients with
myocardial infarction. Russian Cardiology Journal, 2,
18-25.
[14] Egorova, M.V., Afanas’ev, S.A. and Popov, S.V. (2008)
The role of phospholipase A2 in the activation of respira-
tion of isolated cardiomyocytes with infarction cardio-
sclerosis. Bulletin of Experimental Biology and Medicine,
146, 631-633.
[15] (2010) The sequence of events during the development of
non-specific cell responses to injury. In: Novitsky, V.V.,
Goldberg E.D. and Urazova, O.I., Eds., Pathophysiology,
Publishing GEOTAR-Media, Moscow, 1, 59-62.
[16] Lukyanova, L.D. (2004) Mitochondrial dysfunction—a
typical pathological process, the molecular mechanism of
hypoxia. In: Lukyanova L.D. and Ushakov, I.B., Eds.,
Problems of Hypoxia: Molecular, Physiological and
Medical Aspects, Publi “Origins”, Moscow, 8-18.
[17] Maevsky, E.I., Grishina, E.V., Rosenfeld, A.S., Zyakun,
A.M., Kondrashova, M.N. and Vereshchagina, V.M.
(2000) Anaerobic formation of succinate and facilitating
its oxidation—the possible mechanisms of cell adapta-
tion to hypoxia. Biophysics, 45, 509-513.
[18] Millar, C.G.M., Baxter, G.F. and Thiemermann, C. (1996)
Protection of the myocardium by ischaemic precondi-
tioning: mechanisms and therapeutic implications. Phar-
macology & Therapeutics, 69, 143-151.
[19] Yellon, D.M., Dana, A. and Walker, J.M. (1999) En-
dogenous myocardial protection: the importance of me-
tabolic adaptation (preconditioning). Medikography, 21,
80-84.
[20] Yellon, D.M. and Baxter, G.F. (1995) A “second window
of protection” or delayed preconditioning phenomenon:
future horizons for myocardial protection? Journal of
Molecular and Cellular Cardiology, 27, 1023-1034.