 American Journal of Plant Sciences, 2013, 4, 2240-2258 Published Online November 2013 (http://www.scirp.org/journal/ajps) http://dx.doi.org/10.4236/ajps.2013.411277 Open Access AJPS Susceptibility of Wheat Varieties to Soil-Borne Rhizoctonia Infection Gyula Oros1*, Zoltán Naár2, Donát Magyar3 1Plant Protection Institute, Hungarian Academy of Sciences, Budapest, Hungary; 2Department of Microbiology and Food Technol- ogy, Eszterházy Károly College, Eger, Hungary; 3National Institute of Environmental Health, Budapest, Hungary. Email: *gyoros@aim.com Received September 22nd, 2013; revised October 20th, 2013; accepted October 30th, 2013 Copyright © 2013 Gyula Oros et al. This is an open access article distributed under the Creative Commons Attribution License, which permits unrestricted use, distribution, and reproduction in any medium, provided the original work is properly cited. ABSTRACT Response of 19 wheat varieties cultivated in Hungary varied within large limits to soil borne Rhizoctonia infection. The most frequent symptom, usually leading to damping off was the root neck necrosis. Four significant factors influencing the susceptibility of wheat comprised 71% of total variation but none of them was dominant. The inhibition of devel- opment of survivors in Rhizoctonia infested soil correlated with overall susceptibility of variety concerned. The varie- ties Emese, Kikelet and Palotás are proved to be less susceptible, but none of the varieties could be certified as tolerant. No relationships were revealed between pathogenicity of 26 Rhizoctoni a strains studied and their taxonomic position or origin. The anamorph strains of Athelia, Ceratobasidium, Ceratorhiza and Waitea similar to Thanatephorus ana- morphs selectively infected the wheat varieties, but the syndromatic pictures were undistinguishable with unarmed eye. R. solani was proved to be more aggressive against germinating wheat than R. cerealis . Nine significant factors influ- encing the virulence of Rhizoctonia strains comprised 82% of total variation, and six of them influenced exclusively Thanatephorus anamorphs. Keywords: Wheat; Rhizoctonia ; Tolerance; Brown Patch; Soil-Borne; Virulence 1. Introduction In August 2002, brown patches were observed on turf grasses in parks at four locations in Budapest. The symp- toms observed were necrotic lesions on the roots and stems, as well as brown lesions on the leaves. Two types of sclerotia, nearly globose, pinkish to orange and ir- regularly shaped, dark brown were found on roots. On potato dextrose agar fast growing, colourless colonies with small reddish/coloured scelrotia uprose of the first type. This fungus was identified as Rhizo ctonia zeae Voor- hees (teleomorph Waitea circinata Warcup and P.H.B. Talbot) [1]. Buff-coloured, fast growing colonies of Rhizoctonia solani Kühn (teleomorph Thanatephorus cu- cumeris (A.B. Frank) Donk) arose of the second type. The study of more than 150 plant species cultivated in Hungary [2] revealed that R. zeae attacked monocotyle- donous species more aggressively than dicotyledonous ones, contrarily to R. solan i. This latter species was for- merly reported as pathogen of winter wheat [3] and oat [4] in Hungary. Traditionally, farmers paid little attention to field dam- age caused by soilborne Rhizoctonia infection in wheat, because either seedborne or airborne fungi (rust, mildew, smut etc.) infecting stem, leaves and spikelets had been the main constrains of yield. Due to success in breading and arousal of new synthetic fungicides, these fungi presently do not cause catastrophic yield losses. However, in the last two decades increasing number of papers was published on yield losses (30% to 50%) caused by Rhizoctonia species [2,5] in main wheat cultivating areas [6-8]. In Europe and North America winter wheat suf- fered mainly of R. solani AG-8 strains [7] with the R. cerealis [6-8], while in Australia AG-1 and AG-8 and in Turkey five different anastomosis groups of R. solan i [9,10] were revealed. In South Eastern Hungary damage by the R. cerealis and R. solani has been observed in spring wheat [3]. Rhizoctonia species are well known soil borne patho- gens frequently causing damping off prevalently in moist and cool conditions that are the main stress factors re- *Corresponding author.
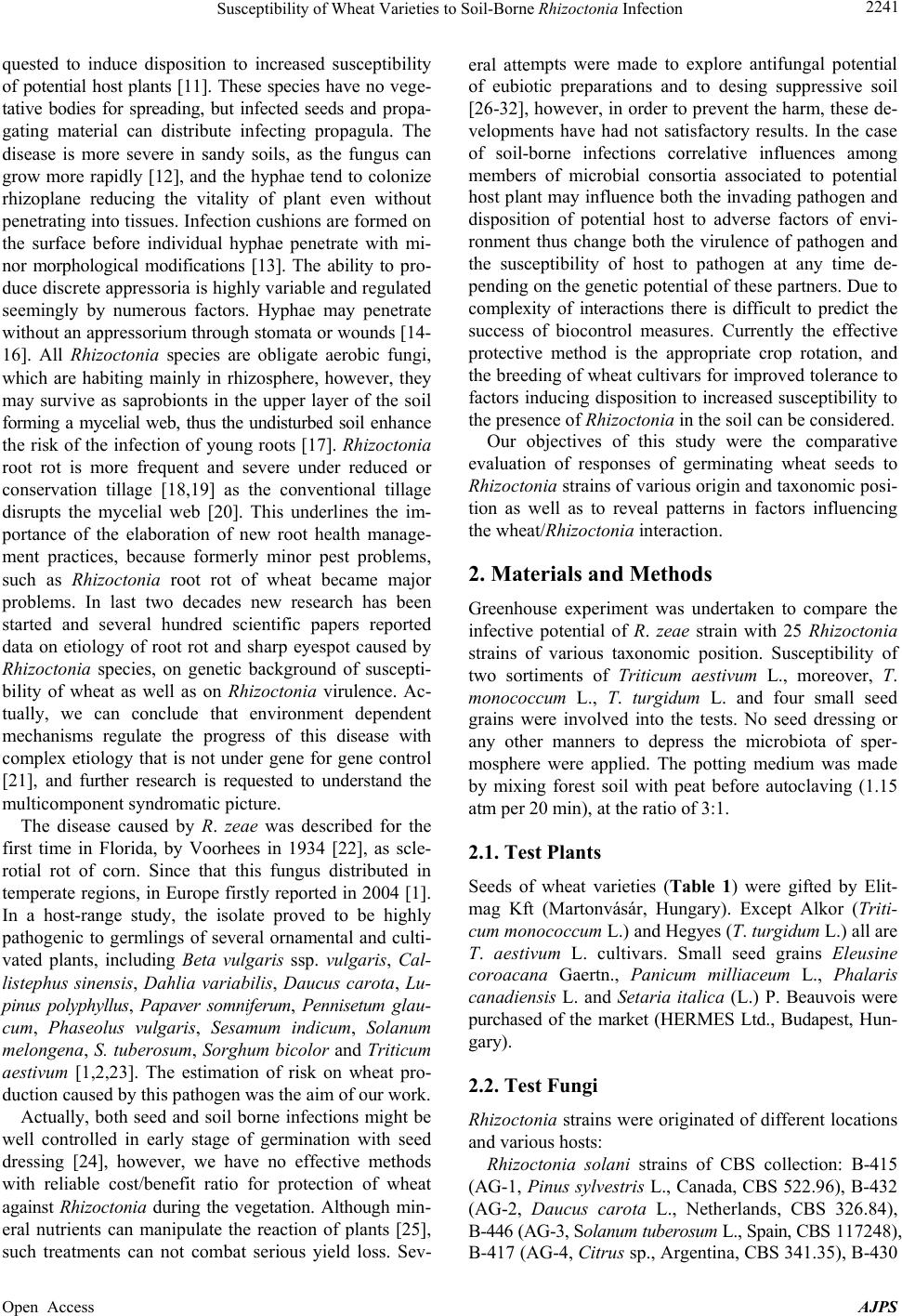 Susceptibility of Wheat Varieties to Soil-Borne Rhizoctonia Infection 2241 quested to induce disposition to increased susceptibility of potential host plants [11]. These species have no vege- tative bodies for spreading, but infected seeds and propa- gating material can distribute infecting propagula. The disease is more severe in sandy soils, as the fungus can grow more rapidly [12], and the hyphae tend to colonize rhizoplane reducing the vitality of plant even without penetrating into tissues. Infection cushions are formed on the surface before individual hyphae penetrate with mi- nor morphological modifications [13]. The ability to pro- duce discrete appressoria is highly variable and regulated seemingly by numerous factors. Hyphae may penetrate without an appressorium through stomata or wounds [14- 16]. All Rhizoctonia species are obligate aerobic fungi, which are habiting mainly in rhizosphere, however, they may survive as saprobionts in the upper layer of the soil forming a mycelial web, thus the undisturbed soil enhance the risk of the infection of young roots [17]. Rhizoctonia root rot is more frequent and severe under reduced or conservation tillage [18,19] as the conventional tillage disrupts the mycelial web [20]. This underlines the im- portance of the elaboration of new root health manage- ment practices, because formerly minor pest problems, such as Rhizoctonia root rot of wheat became major problems. In last two decades new research has been started and several hundred scientific papers reported data on etiology of root rot and sharp eyespot caused by Rhizoctonia species, on genetic background of suscepti- bility of wheat as well as on Rhizoctonia virulence. Ac- tually, we can conclude that environment dependent mechanisms regulate the progress of this disease with complex etiology that is not under gene for gene control [21], and further research is requested to understand the multicomponent syndromatic picture. The disease caused by R. zeae was described for the first time in Florida, by Voorhees in 1934 [22], as scle- rotial rot of corn. Since that this fungus distributed in temperate regions, in Europe firstly reported in 2004 [1]. In a host-range study, the isolate proved to be highly pathogenic to germlings of several ornamental and culti- vated plants, including Beta vulgaris ssp. vulgaris , Cal- listephus sinensis, Dahlia variabilis, Daucus carota, Lu- pinus polyphyllus, Papaver somniferum, Pennisetum glau- cum, Phaseolus vulgaris, Sesamum indicum, Solanum melongena, S. tubero sum, Sorghum bicolor and Triticum aestivum [1,2,23]. The estimation of risk on wheat pro- duction caused by this pathogen was the aim of our work. Actually, both seed and soil borne infections might be well controlled in early stage of germination with seed dressing [24], however, we have no effective methods with reliable cost/benefit ratio for protection of wheat against Rhizoctonia during the vegetation. Although min- eral nutrients can manipulate the reaction of plants [25], such treatments can not combat serious yield loss. Sev- eral attempts were made to explore antifungal potential of eubiotic preparations and to desing suppressive soil [26-32], however, in order to prevent the harm, these de- velopments have had not satisfactory results. In the case of soil-borne infections correlative influences among members of microbial consortia associated to potential host plant may influence both the invading pathogen and disposition of potential host to adverse factors of envi- ronment thus change both the virulence of pathogen and the susceptibility of host to pathogen at any time de- pending on the genetic potential of these partners. Due to complexity of interactions there is difficult to predict the success of biocontrol measures. Currently the effective protective method is the appropriate crop rotation, and the breeding of wheat cultivars for improved tolerance to factors inducing disposition to increased susceptibility to the presence of Rhizoctonia in the soil can be considered. Our objectives of this study were the comparative evaluation of responses of germinating wheat seeds to Rhizoctonia strains of various origin and taxonomic posi- tion as well as to reveal patterns in factors influencing the wheat/Rhizoctonia interaction. 2. Materials and Methods Greenhouse experiment was undertaken to compare the infective potential of R. zeae strain with 25 Rhizoctonia strains of various taxonomic position. Susceptibility of two sortiments of Triticum aestivum L., moreover, T. monococcum L., T. turgidum L. and four small seed grains were involved into the tests. No seed dressing or any other manners to depress the microbiota of sper- mosphere were applied. The potting medium was made by mixing forest soil with peat before autoclaving (1.15 atm per 20 min), at the ratio of 3:1. 2.1. Test Plants Seeds of wheat varieties (Table 1) were gifted by Elit- mag Kft (Martonvásár, Hungary). Except Alkor (Triti- cum monococcum L.) and Hegyes (T. turgidu m L.) all are T. aestivum L. cultivars. Small seed grains Eleusine coroacana Gaertn., Panicum milliaceum L., Phalaris canadiensis L. and Setaria italica (L.) P. Beauvois were purchased of the market (HERMES Ltd., Budapest, Hun- gary). 2.2. Test Fungi Rhizoctonia strains were originated of different locations and various hosts: Rhizoctonia solani strains of CBS collection: B-415 (AG-1, Pinus sylvestris L., Canada, CBS 522.96), B-432 (AG-2, Daucus carota L., Netherlands, CBS 326.84), B-446 (AG-3, Solanum tuberosum L., Spain, CBS 117248), B-417 (AG-4, Citrus sp., Argentina, CBS 341.35), B-430 Open Access AJPS
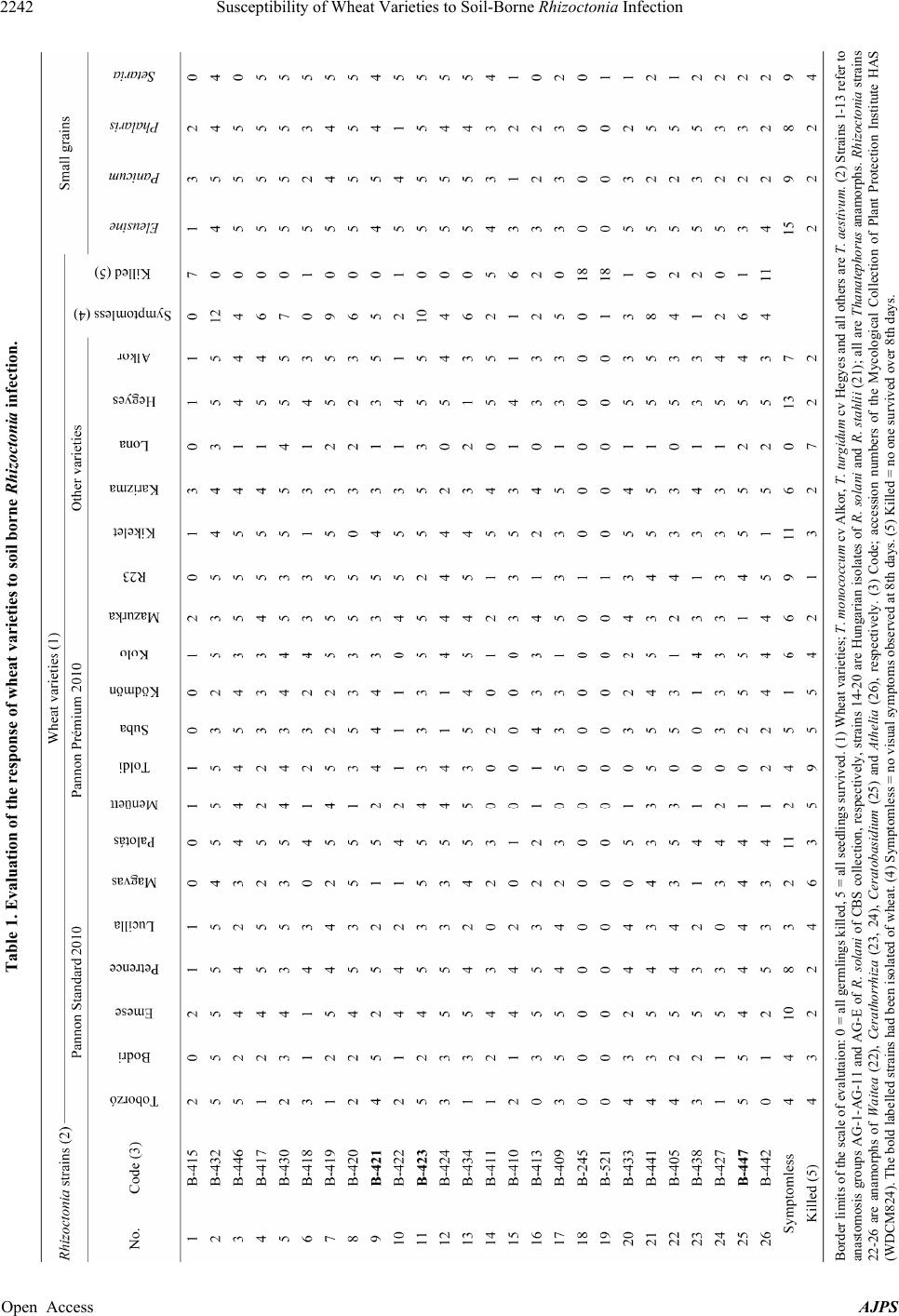 Susceptibility of Wheat Varieties to Soil-Borne Rhizoctonia Infection Open Access AJPS 2242 Border limits of the scale of evalutaion: 0 = all germlings killed, 5 = all seedlings survived. (1) Wheat varieties; T. monococcum cv Alkor, T. turgidum cv Hegyes and all others are T. aestivum. (2) Strains 1-13 refer to anastomosis groups AG-1-AG-11 and AG-E of R. solani of CBS collection, respectively, strains 14-20 are Hungarian isolates of R. solani and R. stahlii (21); all are Thanatephorus anamorphs. Rhizoctonia strains 22-26 are anamorphs of Waitea (22), Cerathorrhiza (23, 24), Ceratobasidium (25) and Athelia (26), respectively. (3) Code; accession numbers of the Mycological Collection of Plant Protection Institute HAS WDCM824 . The bold labelled strains had been isolated of wheat. 4 S m tomless = no visual s m toms observed at 8th da s. 5 Kille = no one survived over 8th da s. Table 1. Evaluation of the response of wheat varieties to soil borne Rhizoctonia infection.
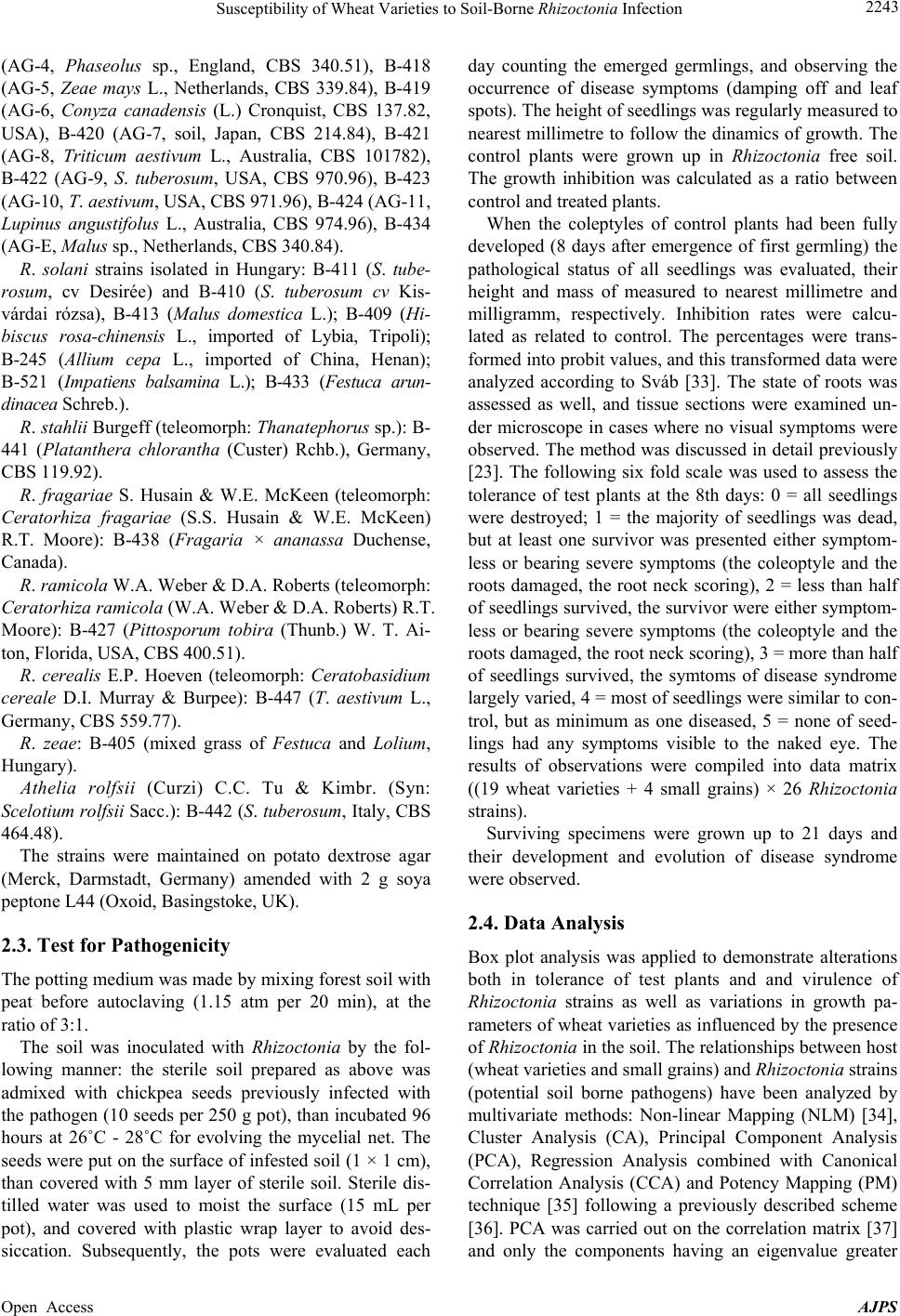 Susceptibility of Wheat Varieties to Soil-Borne Rhizoctonia Infection 2243 (AG-4, Phaseo lus sp., England, CBS 340.51), B-418 (AG-5, Zeae mays L., Netherlands, CBS 339.84), B-419 (AG-6, Conyza canadensis (L.) Cronquist, CBS 137.82, USA), B-420 (AG-7, soil, Japan, CBS 214.84), B-421 (AG-8, Triticum aestivum L., Australia, CBS 101782), B-422 (AG-9, S. tuberosum, USA, CBS 970.96), B-423 (AG-10, T. aestivum, USA, CBS 971.96), B-424 (AG-11, Lupinus angustifolus L., Australia, CBS 974.96), B-434 (AG-E, Malus sp., Netherlands, CBS 340.84). R. solani strains isolated in Hungary: B-411 (S. tube- rosum, cv Desirée) and B-410 (S. tuberosum cv Kis- várdai rózsa), B-413 (Malu s domestica L.); B-409 (Hi- biscus rosa-chinensis L., imported of Lybia, Tripoli); B-245 (Allium cepa L., imported of China, Henan); B-521 (Impatiens balsamina L.); B-433 (Festuca arun- dinacea Schreb.). R. stah lii Burgeff (teleomorph: Thanatephorus sp.): B- 441 (Platanthera chlorantha (Custer) Rchb.), Germany, CBS 119.92). R. fraga riae S. Husain & W.E. McKeen (teleomorph: Ceratorhiza fragariae (S.S. Husain & W.E. McKeen) R.T. Moore): B-438 (Fragaria × ananassa Duchense, Canada). R. ramico la W.A. Weber & D.A. Roberts (teleomorph: Ceratorhiza ramicola (W.A. Weber & D.A. Roberts) R.T. Moore): B-427 (Pittospo rum tobira (Thunb.) W. T. Ai- ton, Florida, USA, CBS 400.51). R. cerealis E.P. Hoeven (teleomorph: Ceratobasidium cereale D.I. Murray & Burpee): B-447 (T. aestivum L., Germany, CBS 559.77). R. zeae: B-405 (mixed grass of Festuca and Lolium, Hungary). Athelia rolfsii (Curzi) C.C. Tu & Kimbr. (Syn: Scelotium rolfsii Sacc.): B-442 (S. tuberosum, Italy, CBS 464.48). The strains were maintained on potato dextrose agar (Merck, Darmstadt, Germany) amended with 2 g soya peptone L44 (Oxoid, Basingstoke, UK). 2.3. Test for Pathogenicity The potting medium was made by mixing forest soil with peat before autoclaving (1.15 atm per 20 min), at the ratio of 3:1. The soil was inoculated with Rhizoctonia by the fol- lowing manner: the sterile soil prepared as above was admixed with chickpea seeds previously infected with the pathogen (10 seeds per 250 g pot), than incubated 96 hours at 26˚C - 28˚C for evolving the mycelial net. The seeds were put on the surface of infested soil (1 × 1 cm), than covered with 5 mm layer of sterile soil. Sterile dis- tilled water was used to moist the surface (15 mL per pot), and covered with plastic wrap layer to avoid des- siccation. Subsequently, the pots were evaluated each day counting the emerged germlings, and observing the occurrence of disease symptoms (damping off and leaf spots). The height of seedlings was regularly measured to nearest millimetre to follow the dinamics of growth. The control plants were grown up in Rhizoctonia free soil. The growth inhibition was calculated as a ratio between control and treated plants. When the coleptyles of control plants had been fully developed (8 days after emergence of first germling) the pathological status of all seedlings was evaluated, their height and mass of measured to nearest millimetre and milligramm, respectively. Inhibition rates were calcu- lated as related to control. The percentages were trans- formed into probit values, and this transformed data were analyzed according to Sváb [33]. The state of roots was assessed as well, and tissue sections were examined un- der microscope in cases where no visual symptoms were observed. The method was discussed in detail previously [23]. The following six fold scale was used to assess the tolerance of test plants at the 8th days: 0 = all seedlings were destroyed; 1 = the majority of seedlings was dead, but at least one survivor was presented either symptom- less or bearing severe symptoms (the coleoptyle and the roots damaged, the root neck scoring), 2 = less than half of seedlings survived, the survivor were either symptom- less or bearing severe symptoms (the coleoptyle and the roots damaged, the root neck scoring), 3 = more than half of seedlings survived, the symtoms of disease syndrome largely varied, 4 = most of seedlings were similar to con- trol, but as minimum as one diseased, 5 = none of seed- lings had any symptoms visible to the naked eye. The results of observations were compiled into data matrix ((19 wheat varieties + 4 small grains) × 26 Rhizoctonia strains). Surviving specimens were grown up to 21 days and their development and evolution of disease syndrome were observed. 2.4. Data Analysis Box plot analysis was applied to demonstrate alterations both in tolerance of test plants and and virulence of Rhizoctonia strains as well as variations in growth pa- rameters of wheat varieties as influenced by the presence of Rhizoctonia in the soil. The relationships between host (wheat varieties and small grains) and Rhizoctonia strains (potential soil borne pathogens) have been analyzed by multivariate methods: Non-linear Mapping (NLM) [34], Cluster Analysis (CA), Principal Component Analysis (PCA), Regression Analysis combined with Canonical Correlation Analysis (CCA) and Potency Mapping (PM) technique [35] following a previously described scheme [36]. PCA was carried out on the correlation matrix [37] and only the components having an eigenvalue greater Open Access AJPS
 Susceptibility of Wheat Varieties to Soil-Borne Rhizoctonia Infection 2244 than one were included in the evaluation of data to dem- onstrate potential number of factors influencing on host parasite system, and the results were not delineated in details. The protocol of experiments is shown in Figure 1. Statistical functions of Microsoft Office Excel 2003 (Microsoft, Redmondton, USA) and Statistica5 program (StatSoft 5.0., Tusla, USA) were used for analysis of data. The graphical presentation of result of data analysis was edited uniformly in MS Office Power Point 2003. 3. Results 3.1. Dynamics of Germination The dynamics of germination of wheat was altered by Rhizoctonia strains in cultivar dependent manner (Figure 2). In majority of cases first germlings emerged within 2 days after sowing in Rhizoctonia free soil, moreover, the process was finished rapidly. The seeds of Pannon Pre- mium sortiment germinated more uniformly, than the other varieties. In the presence of Rhizoctonia strains emergence had been delayed with exception of most tol- erant varieties (Petrence, Emese, Alkor, Hegyes). The last day means the limit after that no further germlings outcropped. Several seeds having been destroyed, seem- ingly, the susceptible individual were killed either before emergence or suffered damping off within 2 - 3 days after outcropping. This ratio strongly varied, and it was not possible to carry out the statistical analysis within frames of the experimental model applied. 3.2. Syndromatic Picture of Disease The growth of infected or diseased seedlings of wheat was conspiciously retarded than the control, however, not all symptoms of disease syndrome turned up. Even seed- lings without any visible symptoms suffered damping off before full development of coleoptyle, the roots of such individuals were symptomless in many cases, although brown spots could be frequently observed on roots and root neck, even in the cases of robust survivors too. In some cases small black spots (<1 mm) were found on root necks, but these were not spread later. Leaf spots with dark brown edge were randomly observed after 6 days. The development of seedlings which survived the infec- Antecedents 1 New disease 2 Survay of host range 3 Survay of data bases Zer o hypothesis Design of experiments Collection of test organism -Wheat varieties -Smal l gr ains -Rhizoctoniastrains Experimental Germinating seeds in Rhizoctoniainfested soil Sampling 8th day Observations Mass Length Coleoptiles Box plot Survivors Obser va tion until 21 days Potency Map Potency Map Box plotBox plot Regression Analysis F5 V Roots Microscopic survay V Dynamics of germination F2 Evaluation of disease syndrome Data Matrix (19+4)×26 Susceptibilty (T olerance) of wh eat cultivar s Virulence of Rhizoctonia strains Potency Map Potency Map Nonlinear Map Response of test plants F3 T1 Regression Analyisis Potency Map Cluster Analysis F8 F6,7 Cluster Analysis F4 F5 Principal Component Analysis Box plot V T2,3 Figure 1. Flow diagram of the experimental protocol. The labels F and T in ellipses mark figures and tables in the body text where the results of computations were used for demonstration, while V means verbal interpretation of result. The zero hy- pothesis was: on the base of screening large number of varieties there is possible to select candidates for breeding wheat cul- tivar tolerant to soil borne Rhizoctonia infection. Open Access AJPS
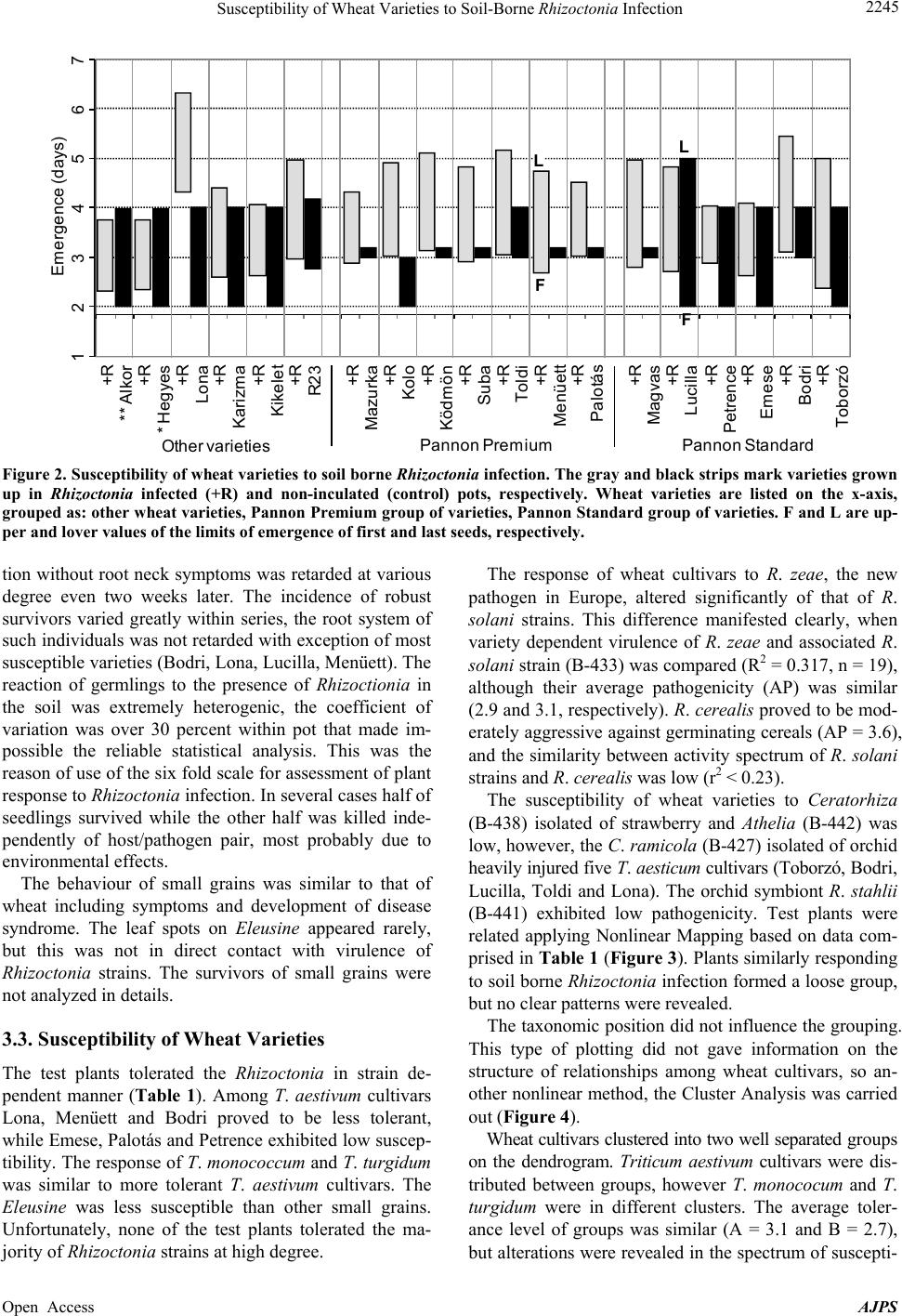 Susceptibility of Wheat Varieties to Soil-Borne Rhizoctonia Infection 2245 Emergence (days) 1234567 F L F L Toborzó Bodri Emese Petrence Lucilla Magvas +R +R +R +R +R +R +R R23 Kikelet Karizma Lona * Hegyes ** Alkor +R +R +R +R +R +R +R +R +R +R +R +R Palo tás Menüett Suba Toldi Ködmön Kolo Mazurka Other varieties Pannon Standard Pannon Premium Figure 2. Susceptibility of wheat varieties to soil borne Rhizoctonia infection. The gray and black stri ps mark varieties grow n up in Rhizoctonia infected (+R) and non-inculated (control) pots, respectively. Wheat varieties are listed on the x-axis, grouped as: other wheat varieties, Pannon Premium group of varieties, Pannon Standard group of varie ties. F and L are up- per and lover values of the limits of emergence of first and last seeds, respectively. tion without root neck symptoms was retarded at various degree even two weeks later. The incidence of robust survivors varied greatly within series, the root system of such individuals was not retarded with exception of most susceptible varieties (Bodri, Lona, Lucilla, Menüett). The reaction of germlings to the presence of Rhizoctionia in the soil was extremely heterogenic, the coefficient of variation was over 30 percent within pot that made im- possible the reliable statistical analysis. This was the reason of use of the six fold scale for assessment of plant response to Rhizocton ia infection. In several cases half of seedlings survived while the other half was killed inde- pendently of host/pathogen pair, most probably due to environmental effects. The behaviour of small grains was similar to that of wheat including symptoms and development of disease syndrome. The leaf spots on Eleusine appeared rarely, but this was not in direct contact with virulence of Rhizoctonia strains. The survivors of small grains were not analyzed in details. 3.3. Susceptibility of Wheat Varieties The test plants tolerated the Rhizoctonia in strain de- pendent manner (Table 1). Among T. aestivum cultivars Lona, Menüett and Bodri proved to be less tolerant, while Emese, Palotás and Petrence exhibited low suscep- tibility. The response of T. monococcum and T. turg idum was similar to more tolerant T. aestivum cultivars. The Eleusine was less susceptible than other small grains. Unfortunately, none of the test plants tolerated the ma- jority of Rhizoctonia strains at high degree. The response of wheat cultivars to R. zeae, the new pathogen in Europe, altered significantly of that of R. solani strains. This difference manifested clearly, when variety dependent virulence of R. zeae and associated R. solani strain (B-433) was compared (R2 = 0.317, n = 19), although their average pathogenicity (AP) was similar (2.9 and 3.1, respectively). R. cerealis proved to be mod- erately aggressive against germinating cereals (AP = 3.6), and the similarity between activity spectrum of R. solan i strains and R. cerealis was low (r2 < 0.23). The susceptibility of wheat varieties to Ceratorhiza (B-438) isolated of strawberry and Athelia (B-442) was low, however, the C. ramic ola (B-427) isolated of orchid heavily injured five T. aesticum cultivars (Toborzó, Bodri, Lucilla, Toldi and Lona). The orchid symbiont R. stah lii (B-441) exhibited low pathogenicity. Test plants were related applying Nonlinear Mapping based on data com- prised in Table 1 (Figure 3). Plants similarly responding to soil borne Rhizoctonia infection formed a loose group, but no clear patterns were revealed. The taxonomic position did not influence the grouping. This type of plotting did not gave information on the structure of relationships among wheat cultivars, so an- other nonlinear method, the Cluster Analysis was carried out (Figure 4). Wheat cultivars clustered into two well separated groups on the dendrogram. Triticum aestivum cultivars were dis- tributed between groups, however T. monoco cum and T. turgidum were in different clusters. The average toler- ance level of groups was similar (A = 3.1 and B = 2.7), but alterations were revealed in the spectrum of suscepti- Open Access AJPS
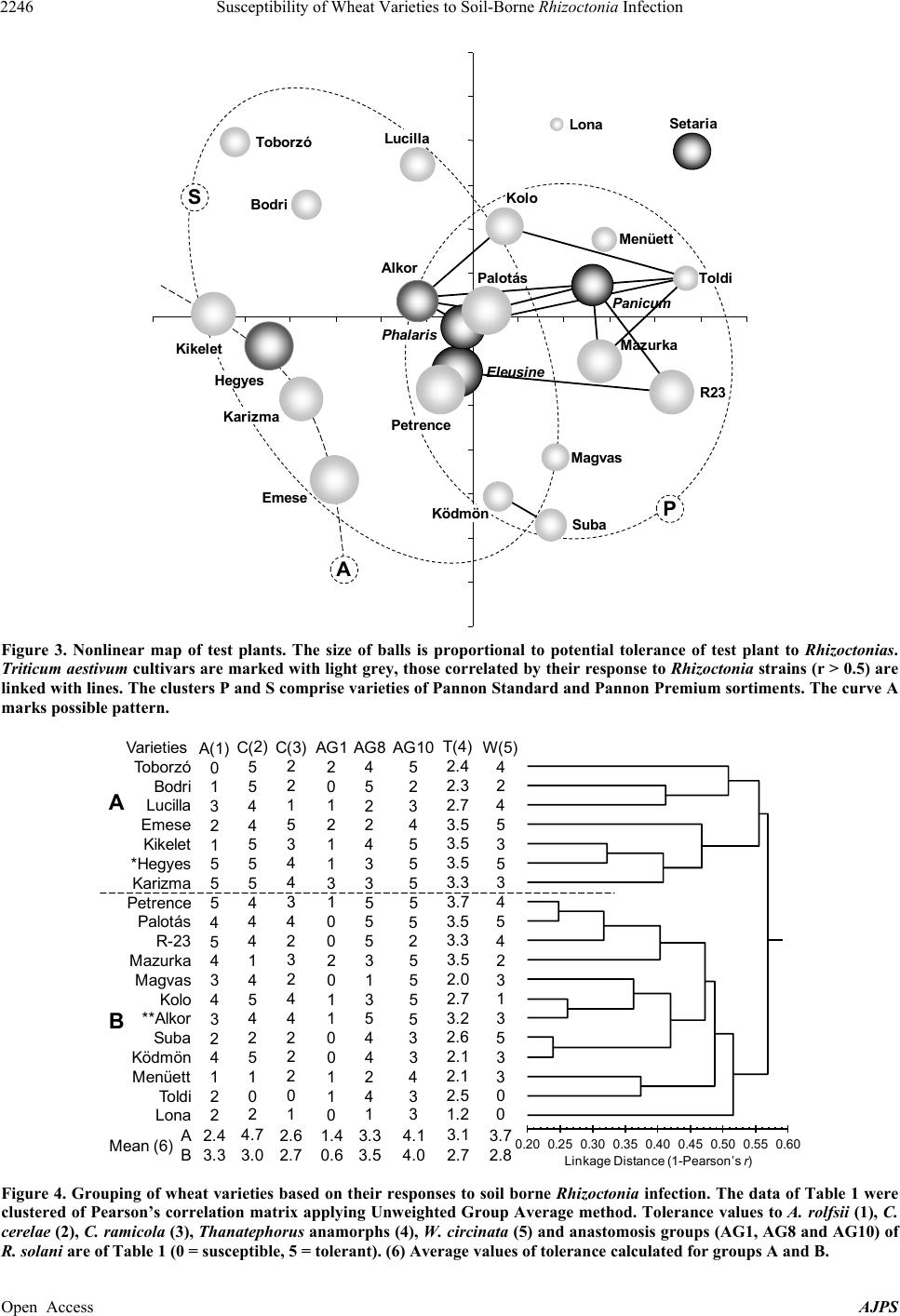 Susceptibility of Wheat Varieties to Soil-Borne Rhizoctonia Infection 2246 A S P Toborzó Bodri Em ese Lucilla Magvas Palotás Menüett Toldi Suba Ködmön Kol o Mazurka R23 Ki kelet Kar izm a Lona Hegyes Al kor El eusine Panicum Phalaris Setaria Petrence Figure 3. Nonlinear map of test plants. The size of balls is proportional to potential tolerance of test plant to Rhizoctonias. Triticum aestivum cultivars are marked with light grey, those correlated by their response to Rhizoctonia strains (r > 0.5) are linked with lines. The clusters P and S comprise varieties of Pannon Standard and Pannon Pre mium sortiments. The c urve A marks possible pattern. Linkage Distance (1- Pearson’s r) 0.200.25 0.30 0.35 0.40 0.45 0.50 0.55 0.60 Toldi M enüett Ködmön Suba **Alkor Kolo Magv as Mazurka R-23 Palotás Petrence Karizma *Hegyes Kikelet Eme se Lucilla Bodri Toborzó Varieties 1. 2 013 2210 Lona 2. 5 2.1 2.1 2.6 3. 2 2.7 2.0 3.5 3.3 3.5 3.7 3. 3 3. 5 3. 5 3. 5 2.7 2. 3 2. 4 T(4) 1 1 0 0 1 1 0 2 0 0 1 3 1 1 2 1 0 2 AG1 4 2 4 4 5 3 1 3 5 5 5 3 3 4 2 2 5 4 AG 8 3 4 3 3 5 5 5 5 2 5 5 5 5 5 4 3 2 5 AG10 2 1 4 2 3 4 3 4 5 4 5 5 5 1 2 3 1 0 A(1) 0 1 5 2 4 5 4 1 4 4 4 5 5 5 4 4 5 5 C(2) 0 2 2 2 4 4 2 3 2 4 3 4 4 3 5 1 2 2 C(3 ) 0 3 3 5 3 1 3 2 4 5 4 3 5 3 5 4 2 4 W(5) A B 3.1 1.4 3.3 4.1 2.4 4.7 2.6 3. 7 A2.7 0.6 3.5 4.0 3.3 3.0 2.7 2.8 B Mea n (6) Figure 4. Grouping of wheat varieties based on their responses to soil borne Rhizoctonia infection. The data of Table 1 were clustered of Pearson’s correlation matrix applying Unweighted Group Average method. Tolerance values to A. rolfsii (1), C. cerelae (2), C. ramicola (3), Thanatephorus anamorphs (4), W. circinata (5) and anastomosis groups (AG1, AG8 and AG10) of R. solani are of Table 1 (0 = susceptible, 5 = tolerant). (6) Average values of tolerance calculated for groups A and B. Open Access AJPS
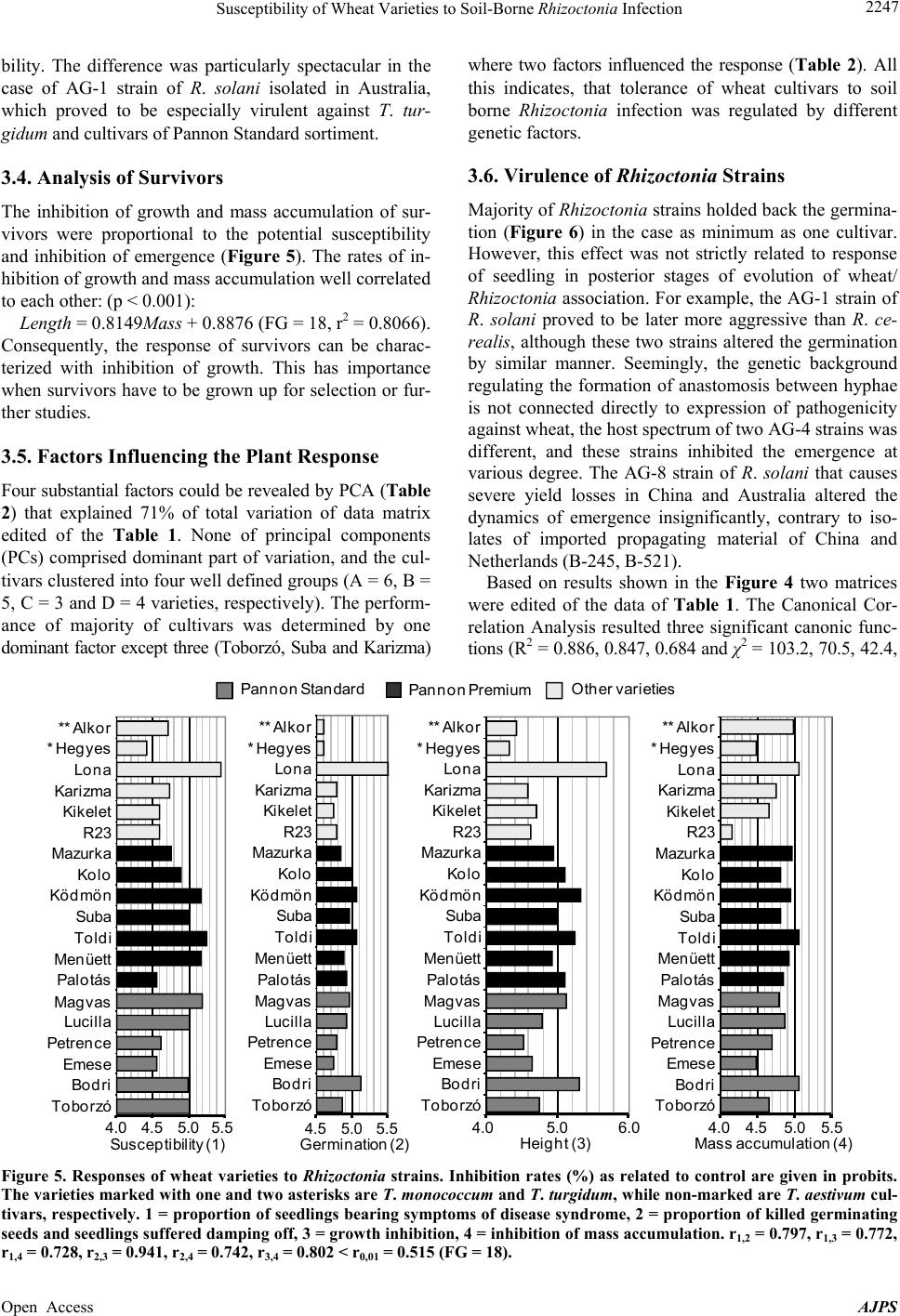 Susceptibility of Wheat Varieties to Soil-Borne Rhizoctonia Infection 2247 bility. The difference was particularly spectacular in the case of AG-1 strain of R. solani isolated in Australia, which proved to be especially virulent against T. tur- gidum and cultivars of Pannon Standard sortiment. 3.4. Analysis of Survivors The inhibition of growth and mass accumulation of sur- vivors were proportional to the potential susceptibility and inhibition of emergence (Figure 5). The rates of in- hibition of growth and mass accumulation well correlated to each other: (p < 0.001): Length = 0.8149Mass + 0.8876 (FG = 18, r2 = 0.8066). Consequently, the response of survivors can be charac- terized with inhibition of growth. This has importance when survivors have to be grown up for selection or fur- ther studies. 3.5. Factors Influencing the Plant Response Four substantial factors could be revealed by PCA (Table 2) that explained 71% of total variation of data matrix edited of the Table 1. None of principal components (PCs) comprised dominant part of variation, and the cul- tivars clustered into four well defined groups (A = 6, B = 5, C = 3 and D = 4 varieties, respectively). The perform- ance of majority of cultivars was determined by one dominant factor except three (Toborzó, Suba and Karizma) where two factors influenced the response (Table 2). All this indicates, that tolerance of wheat cultivars to soil borne Rhizoctonia infection was regulated by different genetic factors. 3.6. Virulence of Rhizoctonia Strains Majority of Rhizocton ia strains holded back the germina- tion (Figure 6) in the case as minimum as one cultivar. However, this effect was not strictly related to response of seedling in posterior stages of evolution of wheat/ Rhizoctonia association. For example, the AG-1 strain of R. solani proved to be later more aggressive than R. ce- realis, although these two strains altered the germination by similar manner. Seemingly, the genetic background regulating the formation of anastomosis between hyphae is not connected directly to expression of pathogenicity against wheat, the host spectrum of two AG-4 strains was different, and these strains inhibited the emergence at various degree. The AG-8 strain of R. solani that causes severe yield losses in China and Australia altered the dynamics of emergence insignificantly, contrary to iso- lates of imported propagating material of China and Netherlands (B-245, B-521). Based on results shown in the Figure 4 two matrices were edited of the data of Table 1. The Canonical Cor- relation Analysis resulted three significant canonic func- tions (R2 = 0.886, 0.847, 0.684 and χ2 = 103.2, 70.5, 42.4, P a nnon S t a nd a rdPannon PremiumOther varieti es 4.04.55.0 5.5 Susceptibility (1) To bor zó Bodri Emese Petrence Lucilla Magvas Palotá s Menüett Toldi Suba Ködmön Kolo Mazur ka R23 Kikelet Karizma Lona * Hegyes ** Alkor 4.0 4.55.0 5.5 Mass accumulation(4) To bo rzó Bodri Emese Petrence Lucilla Magvas Palotá s Menüett To l di Suba Ködmön Kolo Mazurka R23 Kikelet Karizma Lona * Hegyes ** Alkor 4.05.0 6.0 Height(3) To bo rzó Bodri Emese Petrence Lucilla Magvas Palotás Menüett To l di Suba Ködmön Kolo Mazurka R23 Kikelet Karizma Lona * Hegyes ** Alkor 4.55.0 5.5 Germination(2) To bo rzó Bodri Emese Petrence Lucilla Magvas Palotás Menüett To l di Suba Ködmön Kolo Mazurka R23 Kikelet Karizma Lona * Hegyes ** Alkor Figure 5. Responses of wheat varieties to Rhizoctonia strains. Inhibition rates (%) as related to control are given in probits. The varieties marked with one and two asterisks are T. monococcum and T. turgidum, while non-marked are T. aestivum cul- tivars, respectively. 1 = proportion of seedlings bearing symptoms of disease syndrome, 2 = proportion of killed germinating seeds and seedlings suffered damping off, 3 = growth inhibition, 4 = inhibition of mass accumulation. r1,2 = 0.797, r1,3 = 0.772, r1,4 = 0.728, r2,3 = 0.941, r2,4 = 0.742, r3,4 = 0.802 < r0,01 = 0.515 (FG = 18). Open Access AJPS
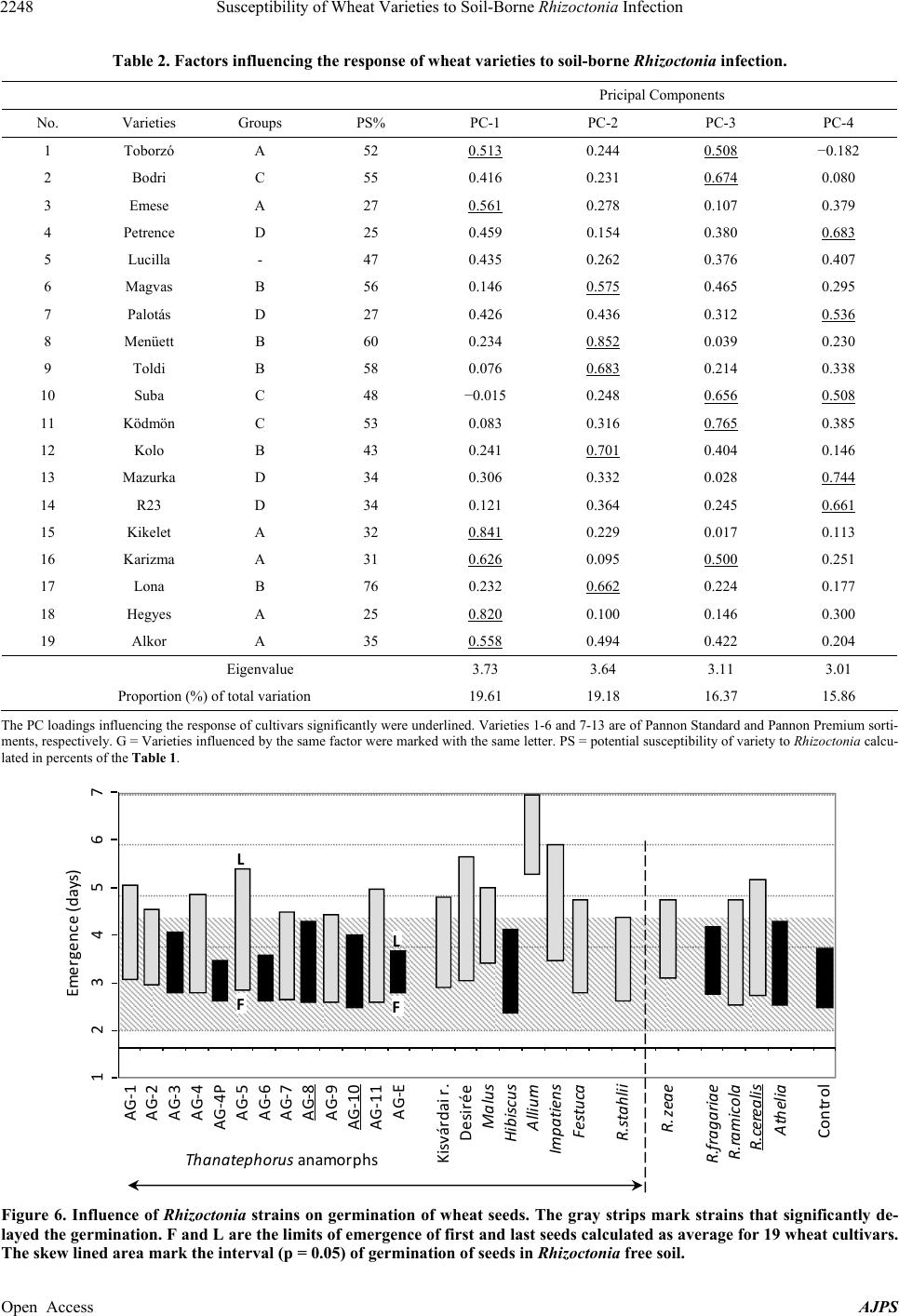 Susceptibility of Wheat Varieties to Soil-Borne Rhizoctonia Infection 2248 Table 2. Factors influencing the response of wheat varieties to soil-borne Rhizoctonia infection. Pricipal Components No. Varieties Groups PS% PC-1 PC-2 PC-3 PC-4 1 Toborzó A 52 0.513 0.244 0.508 −0.182 2 Bodri C 55 0.416 0.231 0.674 0.080 3 Emese A 27 0.561 0.278 0.107 0.379 4 Petrence D 25 0.459 0.154 0.380 0.683 5 Lucilla - 47 0.435 0.262 0.376 0.407 6 Magvas B 56 0.146 0.575 0.465 0.295 7 Palotás D 27 0.426 0.436 0.312 0.536 8 Menüett B 60 0.234 0.852 0.039 0.230 9 Toldi B 58 0.076 0.683 0.214 0.338 10 Suba C 48 −0.015 0.248 0.656 0.508 11 Ködmön C 53 0.083 0.316 0.765 0.385 12 Kolo B 43 0.241 0.701 0.404 0.146 13 Mazurka D 34 0.306 0.332 0.028 0.744 14 R23 D 34 0.121 0.364 0.245 0.661 15 Kikelet A 32 0.841 0.229 0.017 0.113 16 Karizma A 31 0.626 0.095 0.500 0.251 17 Lona B 76 0.232 0.662 0.224 0.177 18 Hegyes A 25 0.820 0.100 0.146 0.300 19 Alkor A 35 0.558 0.494 0.422 0.204 Eigenvalue 3.73 3.64 3.11 3.01 Proportion (%) of total variation 19.61 19.18 16.37 15.86 The PC loadings influencing the response of cultivars significantly were underlined. Varieties 1-6 and 7-13 are of Pannon Standard and Pannon Premium sorti- ments, respectively. G = Varieties influenced by the same factor were marked with the same letter. PS = potential susceptibility of variety to Rhizoctonia calcu- lated in percents of the Table 1. Emergence (days) Thanatephorus anamo rphs 1234567 L F Control Athelia R.cereali s R.ramicola R.fragariae Festuca R.zeae R.stahli i Ma lus Hibiscus Impatiens Allium Desirée Kisvárdair. AG‐1 AG‐2 AG‐3 AG‐4 AG‐4P AG‐6 AG‐5 AG‐7 AG‐8 AG‐9 AG‐10 AG‐11 AG‐E F L Figure 6. Influence of Rhizoctonia strains on germination of wheat seeds. The gray strips mark strains that significantly de- layed the germination. F and L are the limits of emergence of first and last seeds calculated as average for 19 wheat cultivars. The skew lined area mark the interval (p = 0.05) of germination of seeds in Rhizoctonia free soil. Open Access AJPS
 Susceptibility of Wheat Varieties to Soil-Borne Rhizoctonia Infection Open Access AJPS 2249 morphs, indicating that these properties have no decisive role in expression of pathogenicity. The physiological characters, which take place in anastomose contact have also minor importance, for example two R. solani strains of AG-4 anastomosis group (B-417 and B-430) are in notably different position. Similarly, the source of strain had no influence on clustering. For example, the strains isolated of potato tubers were located in different clusters, while anamorphs (B-441 and B-427) of Ceratorrhi za and Thanatephorus of orchid and potato, respectively, were closely related. The taxonomic position of source (host plant) also had no importance, the strains of mono- and dicot plants were placed into the same cluster (see B-417 of Citrus and B-433 of Festuca). Unfortunately, we have few data on exact geographic origin, but surprisingly, all isolates of Hungarian origin were separated into the same cluster. The strains B-413 and B-521 were of imported propagating material. This might be related to role of environmental (biogeographic and bioclimatic) factors. Being typical soil habitants and forming mycelial web in soil [38,39], the soil biota plays a crucial role in the microevolution of Rhizocton ia species, while the assem- blage of soil biota significantly depends on both structure and composition of mineral matrix and climatic condi- tions [40]. respectively). Plotting the strains as canonic scores by first two roots strict linear relationship was revealed, where only few strains deviated (AG-1, AG-5). However, the strains on plot of third canonic function (Figure 7) clus- tered into two well separated groups (p < 0.001). The members of each group differ by their virulence, and the strains of the same anastomosis group split within two clusters, indicating, that the properties responsible for separation into anastomosis groups have minor impor- tance in formation of these clusters. 3.7. Relationships among Rhizoctonia Strains The Rhizo ctonia strains were clustered on the base of their pathogenicity against wheat cultivars (Table 1) based on correlation matrix applying Unweighted Pair Group Average method (Figure 8). No clear patterns can be revealed on dendrogram. Two highly virulent strains iso- lated of onion (B-245) and garden jewelweed (B-521) with poorly virulent AG-E strain separated of others, most probably due to low diversity of response data. The properties responsible for taxonomic position of strains seemingly have minor importance as the anamorphs of Athelia, Cerato basidium, Ceratorrhiza and Waitea spe- cies formed mixed clusters with Thanatephorus ana- B B-441 G-3 G-6 G-7 G-10 G-11 B-410 B-413 B-245 B-521 B-433 B-427 G-1 G-2 G-4 G-4 G-5 G-8 G-9 G-E B-411 B-405 B-438 B-447 B-442 B-409 Figure 7. Separation of Rhizoctonia strains with Canonical Correlation Analysis. According to groups shown in Figure 3 two submatrices were edited of the data comprised in Table 1 and were related by means of CCA. Strains B-409 and B-430 (AG-4) were omitted of calculations. The size of black (A) and gray (B) balls is proportional to potential aggressivity of strains to wheat, respectively. The fitness of regression was over r = 0.97 (p < 0.001) for both function.
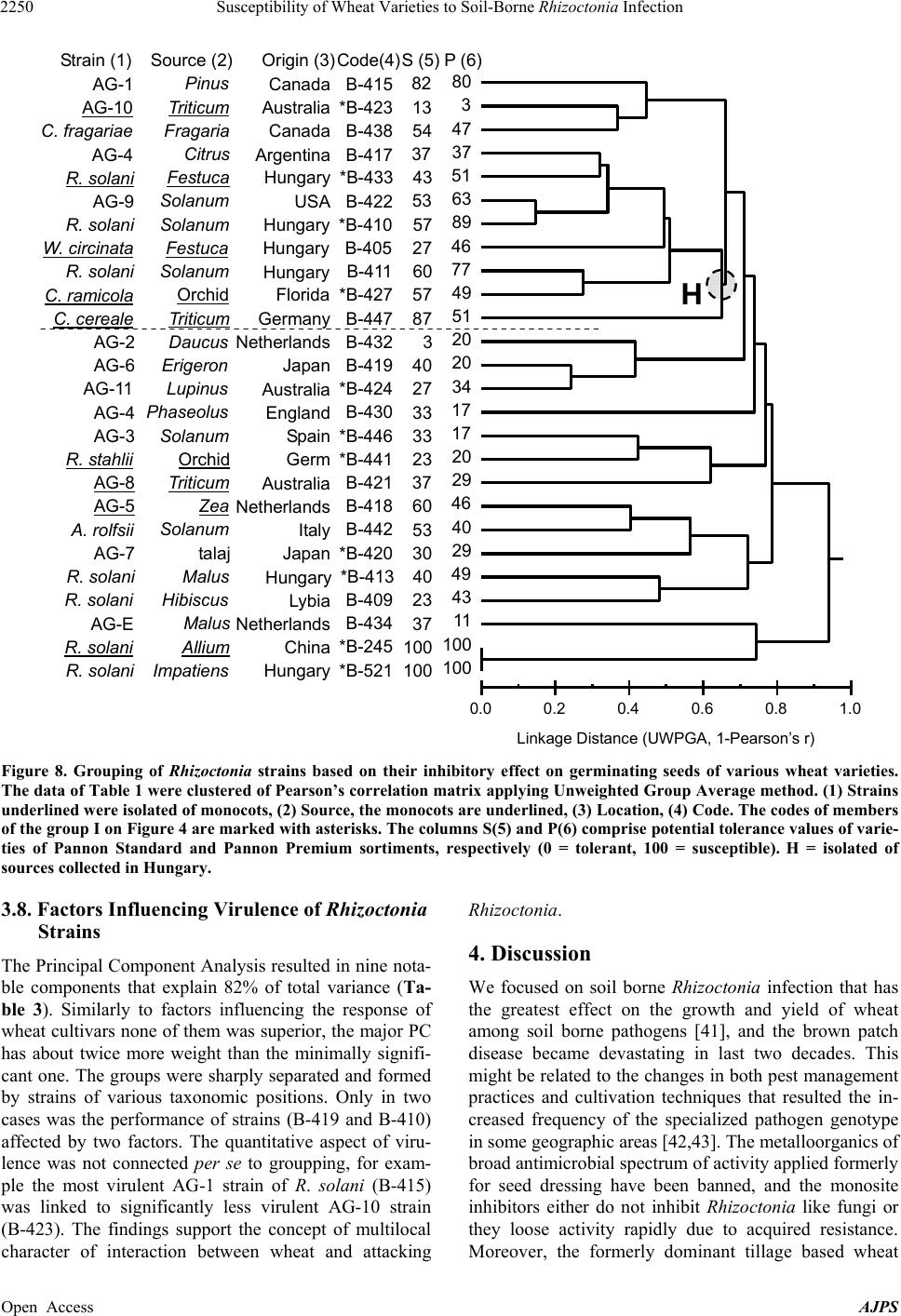 Susceptibility of Wheat Varieties to Soil-Borne Rhizoctonia Infection 2250 R. solani R. solani AG-E R. solani R. solani AG-7 A. rolfsii AG-5 AG-8 R. stahlii AG-3 AG-4 AG-11 AG-6 AG-2 C. cereal e C. ramicola R. solani W. circinata R. solani AG-9 R. solani AG-4 C. fraga r ia e AG-1 0 AG-1 Strain (1) Impatiens Allium Malus Hibiscus Malus tala j Solanum Zea Triticum Orchid Solanum Phaseolus Lupinus Erigeron Daucus Triticum Orchid Solanu m Festuca Solanu m Solanum Festuca Citrus Fragaria Triticum Pinus Source (2) *B-521 *B-245 B-434 B-409 *B-413 *B-420 B-442 B-418 B-421 *B-441 *B-446 B-430 *B-424 B-419 B-432 B-447 *B-427 B-411 B-405 *B-410 B-422 *B-433 B-417 B-438 *B-423 B-415 Code(4) 100 100 37 23 40 30 53 60 37 23 33 33 27 40 3 87 57 60 27 57 53 43 37 54 13 82 S (5) 100 100 11 43 49 29 40 46 29 20 17 17 34 20 20 51 49 77 46 89 63 51 37 47 3 80 P (6) Hungary China Netherlands Lybia Hungary Japan Italy Netherlands Australia Germ Spain England Australia Japan Netherlands Germany Florida Hungary Hungary Hungary USA Hungary Argentina Canada Australia Canada Origin (3) Linkage Distance (UW PGA, 1-Pearson’s r) 0.00.20.40.6 0.8 1.0 Figure 8. Grouping of Rhizoctonia strains based on their inhibitory effect on germinating seeds of various wheat varieties. The data of Table 1 were clustered of Pearson’s correlation matrix applying Unweighted Group Average method. (1) Strains underlined were isolated of monocots, (2) Source, the monocots are underlined, (3) Location, (4) Code. The codes of members of the group I on Figure 4 are marked with asterisks. The columns S(5) and P(6) comprise potential tolerance values of varie- ties of Pannon Standard and Pannon Premium sortiments, respectively (0 = tolerant, 100 = susceptible). H = isolated of sources collected in Hungary. 3.8. Factors Influencing Virulence of Rhizoctonia Strains The Principal Component Analysis resulted in nine nota- ble components that explain 82% of total variance (Ta- ble 3). Similarly to factors influencing the response of wheat cultivars none of them was superior, the major PC has about twice more weight than the minimally signifi- cant one. The groups were sharply separated and formed by strains of various taxonomic positions. Only in two cases was the performance of strains (B-419 and B-410) affected by two factors. The quantitative aspect of viru- lence was not connected per se to groupping, for exam- ple the most virulent AG-1 strain of R. solani (B-415) was linked to significantly less virulent AG-10 strain (B-423). The findings support the concept of multilocal character of interaction between wheat and attacking Rhizoctonia. 4. Discussion We focused on soil borne Rhizoctonia infection that has the greatest effect on the growth and yield of wheat among soil borne pathogens [41], and the brown patch disease became devastating in last two decades. This might be related to the changes in both pest management practices and cultivation techniques that resulted the in- creased frequency of the specialized pathogen genotype in some geographic areas [42,43]. The metalloorganics of broad antimicrobial spectrum of activity applied formerly for seed dressing have been banned, and the monosite inhibitors either do not inhibit Rhizoctonia like fungi or they loose activity rapidly due to acquired resistance. Moreover, the formerly dominant tillage based wheat Open Access AJPS
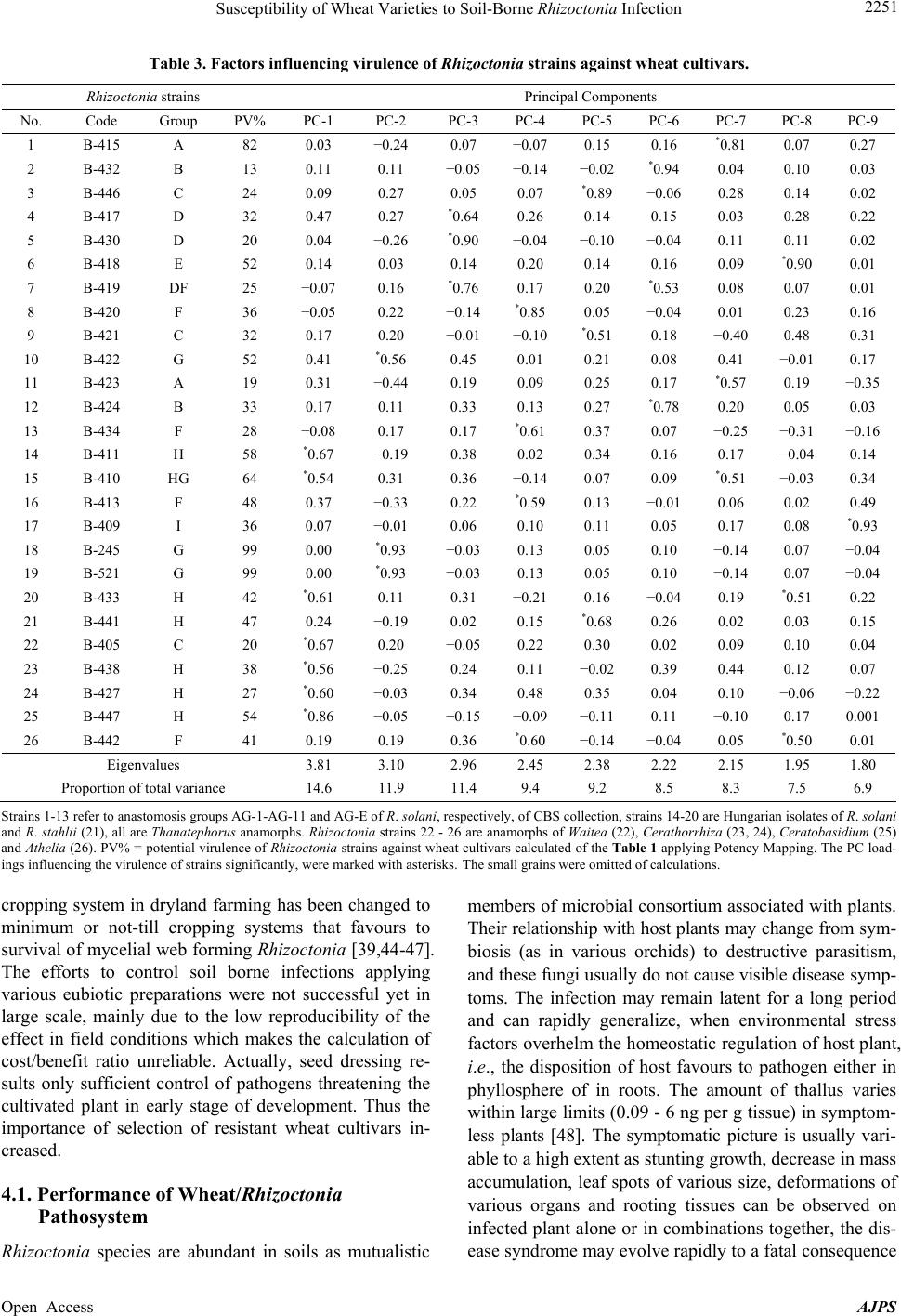 Susceptibility of Wheat Varieties to Soil-Borne Rhizoctonia Infection 2251 Table 3. Factors influencing virulence of Rhizoctonia strains against wheat cultivars. Rhizoctonia strains Principal Components No. Code Group PV% PC-1 PC-2 PC-3 PC-4 PC-5 PC-6 PC-7 PC-8 PC-9 1 B-415 A 82 0.03 −0.24 0.07 −0.07 0.15 0.16 *0.81 0.07 0.27 2 B-432 B 13 0.11 0.11 −0.05 −0.14 −0.02 *0.94 0.04 0.10 0.03 3 B-446 C 24 0.09 0.27 0.05 0.07 *0.89 −0.06 0.28 0.14 0.02 4 B-417 D 32 0.47 0.27 *0.64 0.26 0.14 0.15 0.03 0.28 0.22 5 B-430 D 20 0.04 −0.26 *0.90 −0.04 −0.10 −0.04 0.11 0.11 0.02 6 B-418 E 52 0.14 0.03 0.14 0.20 0.14 0.16 0.09 *0.90 0.01 7 B-419 DF 25 −0.07 0.16 *0.76 0.17 0.20 *0.53 0.08 0.07 0.01 8 B-420 F 36 −0.05 0.22 −0.14 *0.85 0.05 −0.04 0.01 0.23 0.16 9 B-421 C 32 0.17 0.20 −0.01 −0.10 *0.51 0.18 −0.40 0.48 0.31 10 B-422 G 52 0.41 *0.56 0.45 0.01 0.21 0.08 0.41 −0.01 0.17 11 B-423 A 19 0.31 −0.44 0.19 0.09 0.25 0.17 *0.57 0.19 −0.35 12 B-424 B 33 0.17 0.11 0.33 0.13 0.27 *0.78 0.20 0.05 0.03 13 B-434 F 28 −0.08 0.17 0.17 *0.61 0.37 0.07 −0.25 −0.31 −0.16 14 B-411 H 58 *0.67 −0.19 0.38 0.02 0.34 0.16 0.17 −0.04 0.14 15 B-410 HG 64 *0.54 0.31 0.36 −0.14 0.07 0.09 *0.51 −0.03 0.34 16 B-413 F 48 0.37 −0.33 0.22 *0.59 0.13 −0.01 0.06 0.02 0.49 17 B-409 I 36 0.07 −0.01 0.06 0.10 0.11 0.05 0.17 0.08 *0.93 18 B-245 G 99 0.00 *0.93 −0.03 0.13 0.05 0.10 −0.14 0.07 −0.04 19 B-521 G 99 0.00 *0.93 −0.03 0.13 0.05 0.10 −0.14 0.07 −0.04 20 B-433 H 42 *0.61 0.11 0.31 −0.21 0.16 −0.04 0.19 *0.51 0.22 21 B-441 H 47 0.24 −0.19 0.02 0.15 *0.68 0.26 0.02 0.03 0.15 22 B-405 C 20 *0.67 0.20 −0.05 0.22 0.30 0.02 0.09 0.10 0.04 23 B-438 H 38 *0.56 −0.25 0.24 0.11 −0.02 0.39 0.44 0.12 0.07 24 B-427 H 27 *0.60 −0.03 0.34 0.48 0.35 0.04 0.10 −0.06 −0.22 25 B-447 H 54 *0.86 −0.05 −0.15 −0.09 −0.11 0.11 −0.10 0.17 0.001 26 B-442 F 41 0.19 0.19 0.36 *0.60 −0.14 −0.04 0.05 *0.50 0.01 Eigenvalues 3.81 3.10 2.96 2.45 2.38 2.22 2.15 1.95 1.80 Proportion of total variance 14.6 11.9 11.4 9.4 9.2 8.5 8.3 7.5 6.9 Strains 1-13 refer to anastomosis groups AG-1-AG-11 and AG-E of R. solani, respectively, of CBS collection, strains 14-20 are Hungarian isolates of R. solani and R. stahlii (21), all are Thanatephorus anamorphs. Rhizoctonia strains 22 - 26 are anamorphs of Waitea (22), Cerathorrhiza (23, 24), Ceratobasidium (25) and Athelia (26). PV% = potential virulence of Rhizoctonia strains against wheat cultivars calculated of the Table 1 applying Potency Mapping. The PC load- ings influencing the virulence of strains significantly, were marked with asterisks. The small grains were omitted of calculations. cropping system in dryland farming has been changed to minimum or not-till cropping systems that favours to survival of mycelial web forming Rh izoctonia [39,44-47]. The efforts to control soil borne infections applying various eubiotic preparations were not successful yet in large scale, mainly due to the low reproducibility of the effect in field conditions which makes the calculation of cost/benefit ratio unreliable. Actually, seed dressing re- sults only sufficient control of pathogens threatening the cultivated plant in early stage of development. Thus the importance of selection of resistant wheat cultivars in- creased. 4.1. Performance of Wheat/Rhizoctonia Pathosystem Rhizoctonia species are abundant in soils as mutualistic members of microbial consortium associated with plants. Their relationship with host plants may change from sym- biosis (as in various orchids) to destructive parasitism, and these fungi usually do not cause visible disease symp- toms. The infection may remain latent for a long period and can rapidly generalize, when environmental stress factors overhelm the homeostatic regulation of host plant, i.e., the disposition of host favours to pathogen either in phyllosphere of in roots. The amount of thallus varies within large limits (0.09 - 6 ng per g tissue) in symptom- less plants [48]. The symptomatic picture is usually vari- able to a high extent as stunting growth, decrease in mass accumulation, leaf spots of various size, deformations of various organs and rooting tissues can be observed on infected plant alone or in combinations together, the dis- ease syndrome may evolve rapidly to a fatal consequence Open Access AJPS
 Susceptibility of Wheat Varieties to Soil-Borne Rhizoctonia Infection 2252 in formerly symptomless host (damping off and wilting). In our experiments all the above mentioned variations were observed, and the overall susceptibility of one re- spective variety influenced only the frequency of various symptoms of disease syndrome caused by soil borne Rhizoctonia infection independently on taxonomic posi- tion or origin of the strains. This indicates that some traits used for taxonomic classification can not be tightly associated with the properties determining the character of wheat/Rhizoctonia interaction. The small black spots that were occasionally observed on scutellum and meso- cotyl of surviving individuals may be result of hypersen- sitive reaction (HR) suggesting the defense mechanism against Rhizoctonia attack rapidly activates. Further stud- ies requested to connect this property with wheat re- sponse to soil borne Rhizoctonia infection as the in- volvement or manipulation of HR into breeding pro- grams may open door towards to the control of brown patch disease [49]. Furthermore, the role of Rhizoctonia toxins in pathogenesis has to be elucidated as well as factors regulating development of survivors in presence of Rhizoctonia should be identified. The genetic background of tolerance to Rhizoctonias is not elucidated yet. Discoveries show that both anatomic and physiological features are involved into manifesta- tion. Thus, the structure and composition of cell wall might have importance; the resistance to fungal xylanase is a tolerance factor [50]. The multivariate analysis of our experimental results supports the multigenic character of wheat response, although no dominant factor was revealed. Unfortunately, the screening of tolerance to Rhizoctonia in microscale provocative experiments can give data for only preliminary selection of wheat lines, and the survi- vors should be evaluated in field conditions as well [51]. Nevertheless, the data obtained are encouraging and on our opinion the screening of gene banks applying the method demonstrated here can result germlings useful for further manipulations (for example cultivars Emese, Petrence and Toldi). The high variation observed in re- sponse of wheat to soil borne Rhizoctonia might be caused by lack of preliminary selection of tested plants. Members of the genus Rhizoctonia are considered as a complex mixture of filamentous fungi, having in com- mon the possession of a non-spored imperfect state, usu- ally referred to as the anamorphs of five genera: Athelia, Ceratobasidium, C eratocys t is, Thanathephorus and Waitea. Here we included data on virulence of strains of the above five teleomorph genera, all of them attacked germinating seeds of cereals tested. Our results approve presumtion of Tomaso-Peterson and Trevathan [52] that the new for Europe pathogen, R. zeae, is considered as a hazard for wheat cultivation, with special regard to warm climate areas. The multivariate analysis of experimental data re- vealed nine significant factors influencing the aggressiv- ity of these strains; moreover, these factors are clearly not related to traits used for taxonomic purposes. The hyphal anastomosis interactions has been widely used for clustering of Rhizoctonia anamorphs within the complex, since other types of diagnostic features are usually scarce in these fungi [53]. It was considered, that the anastomo- sis groups are specialized to defined host plants [54]. Our experiments do not support this presumption in the case of wheat. Seemingly, the host range of single strains might be different, and reversely, strains clustered into different taxons, may have similar host range. By this reason, on our opinion, —based on presented results here, —for primary screening of wheat cultivars as minimum as six different strains should be used, including A. rolfsii, C. cerealis and W. circinata. 4.2. Future Prospects The plant rhizosphere is a dynamic environment in which many parameters may influence the population structure, diversity and activity of the microbial community. The soil C:N ratio has critical role in disease incidence caused by Rhizoctonia as it was demonstrated by Kuhn et al. [55]. The roles of mycorrhiza in facilitating the acquisi- tion and transfer of carbon (C) and nitrogen (N) is well known. A considerable amount of bidirectional transfer of C between host plant and its fungal symbiont, and a fungus-dependent pathway for organic N can be realized rapidly, thus influencing positively the stress tolerance of plant [56]. The micro- and mesofauna also can alter the disease incidence either wounding the roots [57] or de- creasing the size of inoculum [58]. Induction of suppres- sive soil by using mixed cropping or applying eubiotic preparations is contradictory, as the iron deficiency may harm the wheat although this can be overcame by leaf nutrition. Nevertheless, we can expect new, usable knowl- edge of the environmental research on microbial com- munities and plant microbe interaction studies, which can help to design and sustain suppressive soil that would be the most convenient and economic method for comatting yield losses caused by soil-borne diseases [59]. High number of papers describe antifungal effect of various plant extracts, but only few of them report con- vincing comparative data on efficacy, and in minority of cases these effects have been comparable with marketed fungicides [60,61]. Most of the active substances identi- fied are terpenoids, and on our view there is a few prob- ability to develop potentially effective and environmen- tally safer alternative fungicide to xenobiotics. However, these plants might be sources for transgenic modification to upgrade the sheath blight tolerance of wheat (Table 4). The breading is the most promising measure to improve the tolerance to soil-borne pathogen complex, because the use of any xenobiotic has adverse effects on soil biota Open Access AJPS
 Susceptibility of Wheat Varieties to Soil-Borne Rhizoctonia Infection Open Access AJPS 2253 Table 4. Potential candidates for tr ansge nic manipulation of wheat for improvement of tolerance to Rhizoctonia. Plant Factor Results (example) Ref. Hordeum vulgare rip30 increased tolerance (potato) [71] Hordeum vulgare chitinase increased tolerance (tobacco) [72] Pennisetum glaucum lipid transfer protein antifungal [73] Triticum sp. puroindoline increased tolerance (rice) [74] Celastrus hypoleucus pristimerin inhibiting the formation of infective body [75] Celastrus hypoleucus celastrol inhibiting the formation of infective body [75] Prokaryote 5-enolpyruvoyl-shikimate-3-phosphate synthetase Increased tolerance (wheat/Puccinia) [76] Oryza sativa thaumatin like protein increased tolerance (rice) [70] Oryza sativa OsPR-4b gene encoding pathogenesis related proteinenhanced resistance [77] Solanum tuberosum Potide G proteinase inhibitor [78] Bacillus subtilis Iturin A Antifungal [79] Bacillus subtilis flagellin Antifungal [80] Raphanus sativus defensin increased tolerance (wheat) [81] Solanum tuberosum Snakin 1 enhanced resistance [82] Dasypyrum villosum unknown tolerance to AG 8 [83] Oryza sativa Rice chitinase increased tolerance (Musa/Mycosphaerella) [84] Oryza sativa Rice chitinase increased tolerance (Eleusine/Magnaporthe) [85] Tichoderma harzianum glucanase inhibiting the formation of infective body [86] Tephrosia villosa defensin increased tolerance (tobacco) [87] Arabidopsis thaliana NADPH oxydase induced resistance [88] Oryza sativa ACCA synthase induced resistance [89] as well as can predispose host plant to pathogen [62,63]. In our experiments individual resistant to some Rhizocto- nia, which attack the majority of its fellows, occurred in each variety, likely to observations of other authors [64]. Such survivors can be objects of further breeding or ge- netic engineering. Unfortunately, the mechanistic ap- proach on concern surrounding the genetically modified maize with Bacillus thuringiensis toxin or the glyphosate tolerant crops borne overall social resistance to gene tech- nology. We should clarify, most of these concerns have no scientific base. First of all, the usefulness of geneti- cally modified (GM) crops can be sustained with careful management [65]. Although, Rhizoctonia species, which cause bare-patch disease and sharp eyespot in wheat are not among the top ten pathogens [66], economic improtance of their control is increasing from severe to catasrophic yield losses re- ported from main wheat cultivating areas [67-69]. There is an urgent need in sustainable management strategy to combat damages induced by soil habiting Rhizoctonia complex, first of all, new tolerant wheat cultivars. No major resistance genes to this pathogen have been identi- fied so far inspite of increasing efforts in studies of physiology and genetic background of wheat/ Rhizoc tonia interaction. Nevertheless, candidates for transgenic ma- nipulation can be selected (Table 4). The possible im- provement of tolerance demonstrated on rice [70] might serve as example for wheat. Some terpenoid phytoanticipins of Pelargo nium grav- olens [90], Artemisia arborescens [91], Helianthus tube- rosus [92] exhibited good antifungal effect, but on our opinion the use of antifungal polypeptides seems to be more promising for transgenic manipulation. For exam- ple, a basic oligopeptide of Bacillus subtilis exhibited excellent and broad spectrum antimicrobial activity in our experiments [93] and would fit for control of all four important soil borne pathogens of wheat. The incorpora- tion of Rhizoctonia specific mycovirus into genome of cereals also is a promising possibility [94], with special regard to root border cells. These, detached living cells form the “front line” in the soil, the special part of rhizosphere, described as a system first by Hawes [95], where the plant controls the microenvironment with these specially progammed cells [96]. The border cells have indisputably key function in plant defense controlling the dynamics of adjacent microbial populations in the soil to foster beneficial associations and inhibit pathogenic in- vasion [97], thus these cells are plausible objects of ge- netic engineering to desing wheat plant with optimum characteristics for root health management. PCR based molecular methods enable the comparative analysis of genes involved in plant defense [98]. The task is complex because the design of PCR based molecular markers linked to the Rhizoctonia resistance genes of
 Susceptibility of Wheat Varieties to Soil-Borne Rhizoctonia Infection 2254 wheat cultivars seems to be complicated due to high number of factors influencing on the type of plant re- sponse. Synergic joint action of several minor factor may result more stabile repel than a single major one as one gene mutation can not eliminate this type of defense [90]. However, we understand little about how genes interact because very few possible genetic interactions have been explored experimentally [99], thus depending on the insertion event, a particular transgene can have large effects on the entire phenotype of a plant and that these effects can sometimes be reversed when plants are moved from the glasshouse to the field [100]. On our opinion the more intense research of complex repertoire of small RNAs (microRNAs [miRNAs] and siRNAs) used as guides for post-transcriptional and epigenetic regula- tion would help to design new, either intraspecific or intrageneric wheat varieties. To promote research in this field it is necessary to make public the data on cereal crop genomes and discuss the contribution: what proteins, and their genome sequence organisation, play in plant defence. Although these data are extremely valuable tool for detailed analysis, and the emergence of informatic market makes difficulties as patent applications back out of scientific disputation [101], nevertheless, such free disputation in large scale of scientific community would significantly accelerate the progress in breeding of wheat tolerant to soil borne diseases. 5. Conclusions No relationship was found between taxonomic position and origin of Rhizoctonia strains, indicating that traits used for their classification are not closely related to ex- pression of their pathogenicity against wheat cultivars. Nine factors were revealed that significantly affect their virulence in wheat/Rhizoctonia system. We have got empirical evidence from plant/pathogen system on the possibility of selection; the wheat phenol- type resistant to soil-borne Rhizoctonia, that verifies our approach of using simplified scale for disease assess- ment. 6. Acknowledgements This study was supported by The National Office for Research and Technology, Grant No. K67688. REFERENCES [1] L. Vajna and G. Oros, “First Report of Rhizoctonia zeae in Hungary,” Plant Pathology, Vol. 54, No. 2, 2005, p. 250. http://dx.doi.org/10.1111/j.1365-3059.2005.01172.x [2] G. Oros, “Rhizoctonia Species. Gaps to Be Filled in the Hungarian Soil Science,” Tiszántúli Növényvédelmi Fórum Előadásai, Debrecen, 18-20 October 2004, pp. 321-329. [3] G. J. Kövics and N. Lőrincz, “Causal Agents of Stem- Base Diseases of Winter Wheat in Eastern Hungary,” In: Proceedings of Conference, Resources of the Environ- ment and Sustained Development, Oradea, 24-26 May 2001, Analele Universitátii din Oradea. Tom. VII. Scien- tific Communication Session: Partea I-a. Fascicula Agri- cultură-Horticultura, pp. 37-44. [4] E. I. Simay, “Outbreak of Rhizoctonia solani Kuhn on oat (Avena sativa) in Hungary,” Cereal Research Communi- cations, Vol. 17, No. 3-4, 1998, pp. 233-235. [5] F. Unal and F. S. Dolar, “First Report of Rhizoctonia solani AG 8 on Wheat in Turkey,” Journal of Phytopa- thology, Vol. 160, No. 1, 2012, pp. 52-54. http://dx.doi.org/10.1111/j.1439-0434.2011.01856.x [6] L. Chen, Y. Y. Zhang, H. X. Liang, H. X. Liu, L. P. Du, H. J. Xu and Z. Y. Xin, “Overexpression of TiERF1 En- hances Resistance to Sharp Eyespot in Transgenic Wheat,” Journal of Experimental Botany, Vol. 59, No. 15, 2008, pp. 4195-4204. http://dx.doi.org/10.1093/jxb/ern259 [7] M. S. Hamada, Y. N. Yin, H. G. Chen and Z. H. Ma, “The Escalating Threat of Rhizoctonia cerealis, the Causal Agent of Sharp Eyespot in Wheat,” Pest Man- agement Science, Vol. 67, No. 11, 2011, pp. 1411-1419. http://dx.doi.org/10.1002/ps.2236 [8] Y. P. Guo, W. Li, H. Y. Sun, N. Wang, H. S. Yu and H. G. Chen, “Detection and Quantification of Rhizoctonia cerealis in Soil Using Real-Time PCR,” Journal of Gen- eral Plant Pathology, Vol. 78, No. 4, 2012, pp. 247-254. http://dx.doi.org/10.1007/s10327-012-0390-x [9] E. Demirci, “Rhizoctonia Species and Anastomosis Groups Isolated from Barley and Wheat in Erzurum, Turkey,” Plant Pathology, Vol. 47, No. 1, 1998, pp. 10-15. http://dx.doi.org/10.1046/j.1365-3059.1998.00214.x [10] B. Tunali, J. M. Nicol, D. Hodson, Z. Uckun, O. Buyuk, D. Erdurmus, H. Hekimhan, H. Aktas, M. A. Akbudak and S. A. Bagci, “Root and Crown Rot Fungi Associated with Spring, Facultative, and Winter Wheat in Turkey,” Plant Disease, Vol. 92, No. 9, 2008, pp. 1299-1306. http://dx.doi.org/10.1094/PDIS-92-9-1299 [11] R. G. Grogan, “The Science and Art of Plant Disease Diagnosis,” Annual Review of Plant Pathology, Vol. 19, 1981, pp. 333-351. http://dx.doi.org/10.1146/annurev.py.25.090187.000245 [12] J. S. Gill, K. Sivasithamparam and K. R. J. Smettem, “Soil Types with Different Texture Affects Development of Rhizoctonia Root Rot of Wheat Seedlings,” Plant and Soil, Vol. 221, No. 2, 2000, pp. 113-120. http://dx.doi.org/10.1023/A:1004606016745 [13] J. Keijer, “The Initial Steps of the Infection Process In Rhizoctonia solani,” In: B. Sneh, S. Jabaji-Hare, S. Neate, and G. Dijst, Eds., Rhizoctonia Species: Taxonomy, Ecol- ogy, Pathology and Disease Control, Kluwer, Dordrecht, 1996, pp. 149-162. http://dx.doi.org/10.1007/978-94-017-2901-7_13 [14] V. N. Armentrout anf A. J. Downer, “Infection Cushion Development by Rhizoctonia solani on Cotton,” Phyto- pathogy, Vol. 77, 1987, pp. 623-630. http://dx.doi.org/10.1094/Phyto-77-623 [15] D. I. L. Murray, “Penetration of Barley Root and Coleoptile Open Access AJPS
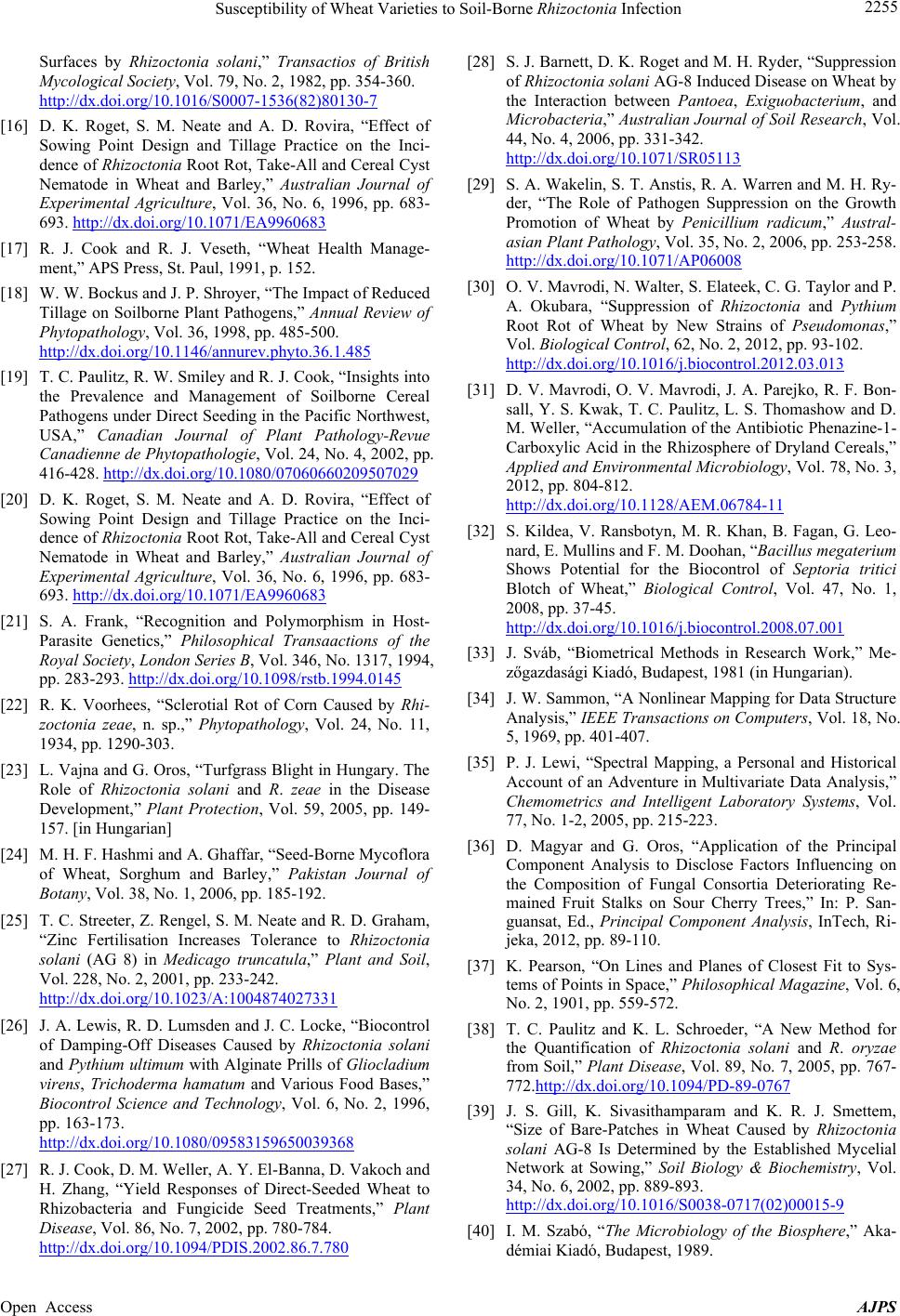 Susceptibility of Wheat Varieties to Soil-Borne Rhizoctonia Infection 2255 Surfaces by Rhizoctonia solani,” Transactios of British Mycological Society, Vol. 79, No. 2, 1982, pp. 354-360. http://dx.doi.org/10.1016/S0007-1536(82)80130-7 [16] D. K. Roget, S. M. Neate and A. D. Rovira, “Effect of Sowing Point Design and Tillage Practice on the Inci- dence of Rhizoctonia Root Rot, Take-All and Cereal Cyst Nematode in Wheat and Barley,” Australian Journal of Experimental Agriculture, Vol. 36, No. 6, 1996, pp. 683- 693. http://dx.doi.org/10.1071/EA9960683 [17] R. J. Cook and R. J. Veseth, “Wheat Health Manage- ment,” APS Press, St. Paul, 1991, p. 152. [18] W. W. Bockus and J. P. Shroyer, “The Impact of Reduced Tillage on Soilborne Plant Pathogens,” Annual Review of Phytopathology, Vol. 36, 1998, pp. 485-500. http://dx.doi.org/10.1146/annurev.phyto.36.1.485 [19] T. C. Paulitz, R. W. Smiley and R. J. Cook, “Insights into the Prevalence and Management of Soilborne Cereal Pathogens under Direct Seeding in the Pacific Northwest, USA,” Canadian Journal of Plant Pathology-Revue Canadienne de Phytopathologie, Vol. 24, No. 4, 2002, pp. 416-428. http://dx.doi.org/10.1080/07060660209507029 [20] D. K. Roget, S. M. Neate and A. D. Rovira, “Effect of Sowing Point Design and Tillage Practice on the Inci- dence of Rhizoctonia Root Rot, Take-All and Cereal Cyst Nematode in Wheat and Barley,” Australian Journal of Experimental Agriculture, Vol. 36, No. 6, 1996, pp. 683- 693. http://dx.doi.org/10.1071/EA9960683 [21] S. A. Frank, “Recognition and Polymorphism in Host- Parasite Genetics,” Philosophical Transaactions of the Royal Society, London Series B, Vol. 346, No. 1317, 1994, pp. 283-293. http://dx.doi.org/10.1098/rstb.1994.0145 [22] R. K. Voorhees, “Sclerotial Rot of Corn Caused by Rhi- zoctonia zeae, n. sp.,” Phytopathology, Vol. 24, No. 11, 1934, pp. 1290-303. [23] L. Vajna and G. Oros, “Turfgrass Blight in Hungary. The Role of Rhizoctonia solani and R. zeae in the Disease Development,” Plant Protection, Vol. 59, 2005, pp. 149- 157. [in Hungarian] [24] M. H. F. Hashmi and A. Ghaffar, “Seed-Borne Mycoflora of Wheat, Sorghum and Barley,” Pakistan Journal of Botany, Vol. 38, No. 1, 2006, pp. 185-192. [25] T. C. Streeter, Z. Rengel, S. M. Neate and R. D. Graham, “Zinc Fertilisation Increases Tolerance to Rhizoctonia solani (AG 8) in Medicago truncatula,” Plant and Soil, Vol. 228, No. 2, 2001, pp. 233-242. http://dx.doi.org/10.1023/A:1004874027331 [26] J. A. Lewis, R. D. Lumsden and J. C. Locke, “Biocontrol of Damping-Off Diseases Caused by Rhizoctonia solani and Pythium ultimum with Alginate Prills of Gliocladium virens, Trichoderma hamatum and Various Food Bases,” Biocontrol Science and Technology, Vol. 6, No. 2, 1996, pp. 163-173. http://dx.doi.org/10.1080/09583159650039368 [27] R. J. Cook, D. M. Weller, A. Y. El-Banna, D. Vakoch and H. Zhang, “Yield Responses of Direct-Seeded Wheat to Rhizobacteria and Fungicide Seed Treatments,” Plant Disease, Vol. 86, No. 7, 2002, pp. 780-784. http://dx.doi.org/10.1094/PDIS.2002.86.7.780 [28] S. J. Barnett, D. K. Roget and M. H. Ryder, “Suppression of Rhizoctonia solani AG-8 Induced Disease on Wheat by the Interaction between Pantoea, Exiguobacterium, and Microbacteria,” Australian Journal of Soil Research, Vol. 44, No. 4, 2006, pp. 331-342. http://dx.doi.org/10.1071/SR05113 [29] S. A. Wakelin, S. T. Anstis, R. A. Warren and M. H. Ry- der, “The Role of Pathogen Suppression on the Growth Promotion of Wheat by Penicillium radicum,” Austral- asian Plant Pathology, Vol. 35, No. 2, 2006, pp. 253-258. http://dx.doi.org/10.1071/AP06008 [30] O. V. Mavrodi, N. Walter, S. Elateek, C. G. Taylor and P. A. Okubara, “Suppression of Rhizoctonia and Pythium Root Rot of Wheat by New Strains of Pseudomonas,” Vol. Biological Control, 62, No. 2, 2012, pp. 93-102. http://dx.doi.org/10.1016/j.biocontrol.2012.03.013 [31] D. V. Mavrodi, O. V. Mavrodi, J. A. Parejko, R. F. Bon- sall, Y. S. Kwak, T. C. Paulitz, L. S. Thomashow and D. M. Weller, “Accumulation of the Antibiotic Phenazine-1- Carboxylic Acid in the Rhizosphere of Dryland Cereals,” Applied and Environmental Microbiology, Vol. 78, No. 3, 2012, pp. 804-812. http://dx.doi.org/10.1128/AEM.06784-11 [32] S. Kildea, V. Ransbotyn, M. R. Khan, B. Fagan, G. Leo- nard, E. Mullins and F. M. Doohan, “Bacillus megaterium Shows Potential for the Biocontrol of Septoria tritici Blotch of Wheat,” Biological Control, Vol. 47, No. 1, 2008, pp. 37-45. http://dx.doi.org/10.1016/j.biocontrol.2008.07.001 [33] J. Sváb, “Biometrical Methods in Research Work,” Me- zőgazdasági Kiadó, Budapest, 1981 (in Hungarian). [34] J. W. Sammon, “A Nonlinear Mapping for Data Structure Analysis,” IEEE Transactions on Computers, Vol. 18, No. 5, 1969, pp. 401-407. [35] P. J. Lewi, “Spectral Mapping, a Personal and Historical Account of an Adventure in Multivariate Data Analysis,” Chemometrics and Intelligent Laboratory Systems, Vol. 77, No. 1-2, 2005, pp. 215-223. [36] D. Magyar and G. Oros, “Application of the Principal Component Analysis to Disclose Factors Influencing on the Composition of Fungal Consortia Deteriorating Re- mained Fruit Stalks on Sour Cherry Trees,” In: P. San- guansat, Ed., Principal Component Analysis, InTech, Ri- jeka, 2012, pp. 89-110. [37] K. Pearson, “On Lines and Planes of Closest Fit to Sys- tems of Points in Space,” Philosophical Magazine, Vol. 6, No. 2, 1901, pp. 559-572. [38] T. C. Paulitz and K. L. Schroeder, “A New Method for the Quantification of Rhizoctonia solani and R. oryzae from Soil,” Plant Disease, Vol. 89, No. 7, 2005, pp. 767- 772.http://dx.doi.org/10.1094/PD-89-0767 [39] J. S. Gill, K. Sivasithamparam and K. R. J. Smettem, “Size of Bare-Patches in Wheat Caused by Rhizoctonia solani AG-8 Is Determined by the Established Mycelial Network at Sowing,” Soil Biology & Biochemistry, Vol. 34, No. 6, 2002, pp. 889-893. http://dx.doi.org/10.1016/S0038-0717(02)00015-9 [40] I. M. Szabó, “The Microbiology of the Biosphere,” Aka- démiai Kiadó, Budapest, 1989. Open Access AJPS
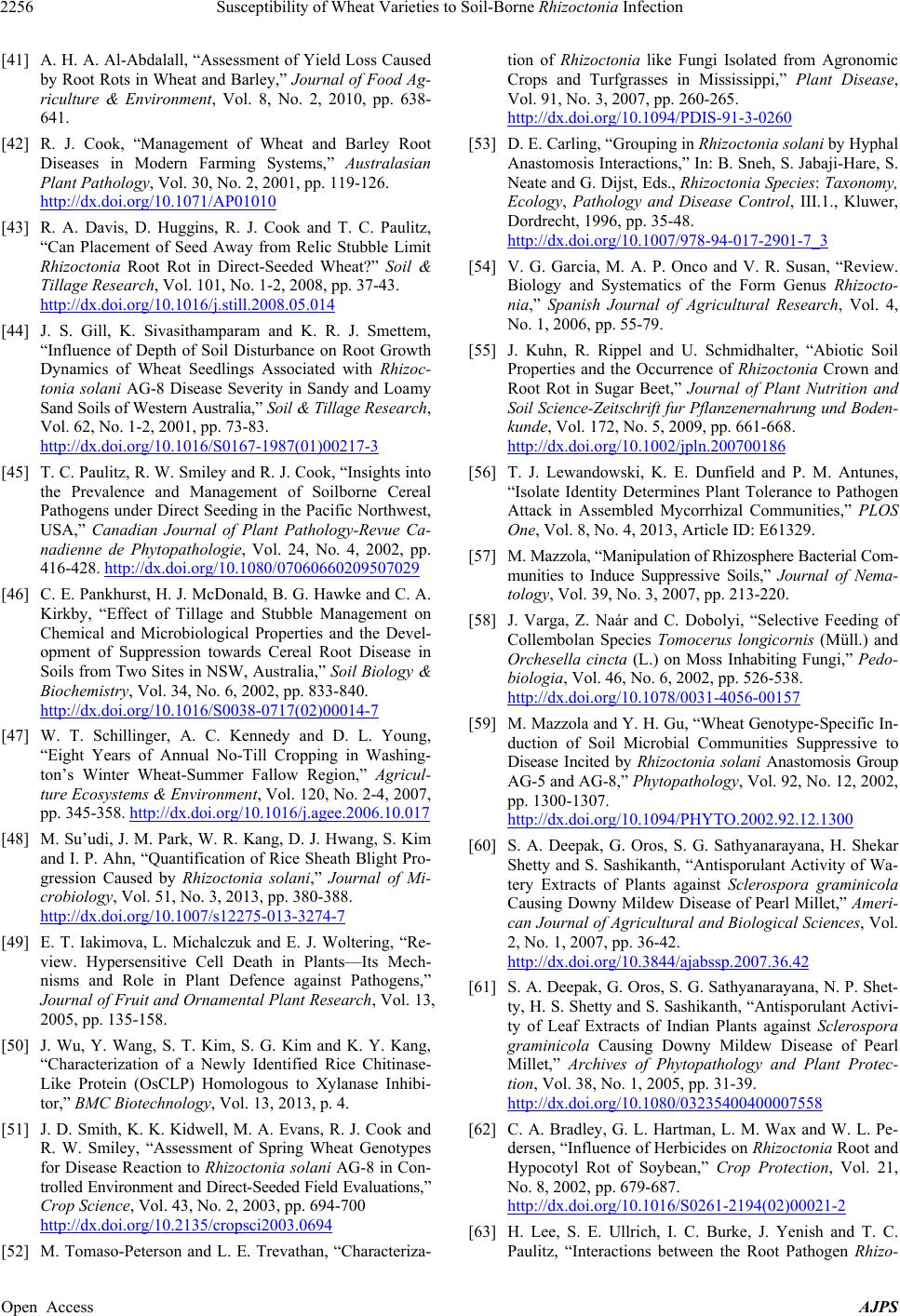 Susceptibility of Wheat Varieties to Soil-Borne Rhizoctonia Infection 2256 [41] A. H. A. Al-Abdalall, “Assessment of Yield Loss Caused by Root Rots in Wheat and Barley,” Journal of Food Ag- riculture & Environment, Vol. 8, No. 2, 2010, pp. 638- 641. [42] R. J. Cook, “Management of Wheat and Barley Root Diseases in Modern Farming Systems,” Australasian Plant Pathology, Vol. 30, No. 2, 2001, pp. 119-126. http://dx.doi.org/10.1071/AP01010 [43] R. A. Davis, D. Huggins, R. J. Cook and T. C. Paulitz, “Can Placement of Seed Away from Relic Stubble Limit Rhizoctonia Root Rot in Direct-Seeded Wheat?” Soil & Tillage Research, Vol. 101, No. 1-2, 2008, pp. 37-43. http://dx.doi.org/10.1016/j.still.2008.05.014 [44] J. S. Gill, K. Sivasithamparam and K. R. J. Smettem, “Influence of Depth of Soil Disturbance on Root Growth Dynamics of Wheat Seedlings Associated with Rhizoc- tonia solani AG-8 Disease Severity in Sandy and Loamy Sand Soils of Western Australia,” Soil & Tillage Research, Vol. 62, No. 1-2, 2001, pp. 73-83. http://dx.doi.org/10.1016/S0167-1987(01)00217-3 [45] T. C. Paulitz, R. W. Smiley and R. J. Cook, “Insights into the Prevalence and Management of Soilborne Cereal Pathogens under Direct Seeding in the Pacific Northwest, USA,” Canadian Journal of Plant Pathology-Revue Ca- nadienne de Phytopathologie, Vol. 24, No. 4, 2002, pp. 416-428. http://dx.doi.org/10.1080/07060660209507029 [46] C. E. Pankhurst, H. J. McDonald, B. G. Hawke and C. A. Kirkby, “Effect of Tillage and Stubble Management on Chemical and Microbiological Properties and the Devel- opment of Suppression towards Cereal Root Disease in Soils from Two Sites in NSW, Australia,” Soil Biology & Biochemistry, Vol. 34, No. 6, 2002, pp. 833-840. http://dx.doi.org/10.1016/S0038-0717(02)00014-7 [47] W. T. Schillinger, A. C. Kennedy and D. L. Young, “Eight Years of Annual No-Till Cropping in Washing- ton’s Winter Wheat-Summer Fallow Region,” Agricul- ture Ecosystems & Environment, Vol. 120, No. 2-4, 2007, pp. 345-358. http://dx.doi.org/10.1016/j.agee.2006.10.017 [48] M. Su’udi, J. M. Park, W. R. Kang, D. J. Hwang, S. Kim and I. P. Ahn, “Quantification of Rice Sheath Blight Pro- gression Caused by Rhizoctonia solani,” Journal of Mi- crobiology, Vol. 51, No. 3, 2013, pp. 380-388. http://dx.doi.org/10.1007/s12275-013-3274-7 [49] E. T. Iakimova, L. Michalczuk and E. J. Woltering, “Re- view. Hypersensitive Cell Death in Plants—Its Mech- nisms and Role in Plant Defence against Pathogens,” Journal of Fruit and Ornamental Plant Research, Vol. 13, 2005, pp. 135-158. [50] J. Wu, Y. Wang, S. T. Kim, S. G. Kim and K. Y. Kang, “Characterization of a Newly Identified Rice Chitinase- Like Protein (OsCLP) Homologous to Xylanase Inhibi- tor,” BMC Biotechnology, Vol. 13, 2013, p. 4. [51] J. D. Smith, K. K. Kidwell, M. A. Evans, R. J. Cook and R. W. Smiley, “Assessment of Spring Wheat Genotypes for Disease Reaction to Rhizoctonia solani AG-8 in Con- trolled Environment and Direct-Seeded Field Evaluations,” Crop Science, Vol. 43, No. 2, 2003, pp. 694-700 http://dx.doi.org/10.2135/cropsci2003.0694 [52] M. Tomaso-Peterson and L. E. Trevathan, “Characteriza- tion of Rhizoctonia like Fungi Isolated from Agronomic Crops and Turfgrasses in Mississippi,” Plant Disease, Vol. 91, No. 3, 2007, pp. 260-265. http://dx.doi.org/10.1094/PDIS-91-3-0260 [53] D. E. Carling, “Grouping in Rhizoctonia solani by Hyphal Anastomosis Interactions,” In: B. Sneh, S. Jabaji-Hare, S. Neate and G. Dijst, Eds., Rhizoctonia Species: Taxonomy, Ecology, Pathology and Disease Control, III.1., Kluwer, Dordrecht, 1996, pp. 35-48. http://dx.doi.org/10.1007/978-94-017-2901-7_3 [54] V. G. Garcia, M. A. P. Onco and V. R. Susan, “Review. Biology and Systematics of the Form Genus Rhizocto- nia,” Spanish Journal of Agricultural Research, Vol. 4, No. 1, 2006, pp. 55-79. [55] J. Kuhn, R. Rippel and U. Schmidhalter, “Abiotic Soil Properties and the Occurrence of Rhizoctonia Crown and Root Rot in Sugar Beet,” Journal of Plant Nutrition and Soil Science-Zeitschrift fur Pflanzenernahrung und Boden- kunde, Vol. 172, No. 5, 2009, pp. 661-668. http://dx.doi.org/10.1002/jpln.200700186 [56] T. J. Lewandowski, K. E. Dunfield and P. M. Antunes, “Isolate Identity Determines Plant Tolerance to Pathogen Attack in Assembled Mycorrhizal Communities,” PLOS One, Vol. 8, No. 4, 2013, Article ID: E61329. [57] M. Mazzola, “Manipulation of Rhizosphere Bacterial Com- munities to Induce Suppressive Soils,” Journal of Nema- tology, Vol. 39, No. 3, 2007, pp. 213-220. [58] J. Varga, Z. Naár and C. Dobolyi, “Selective Feeding of Collembolan Species Tomocerus longicornis (Müll.) and Orchesella cincta (L.) on Moss Inhabiting Fungi,” Pedo- biologia, Vol. 46, No. 6, 2002, pp. 526-538. http://dx.doi.org/10.1078/0031-4056-00157 [59] M. Mazzola and Y. H. Gu, “Wheat Genotype-Specific In- duction of Soil Microbial Communities Suppressive to Disease Incited by Rhizoctonia solani Anastomosis Group AG-5 and AG-8,” Phytopathology, Vol. 92, No. 12, 2002, pp. 1300-1307. http://dx.doi.org/10.1094/PHYTO.2002.92.12.1300 [60] S. A. Deepak, G. Oros, S. G. Sathyanarayana, H. Shekar Shetty and S. Sashikanth, “Antisporulant Activity of Wa- tery Extracts of Plants against Sclerospora graminicola Causing Downy Mildew Disease of Pearl Millet,” Ameri- can Journal of Agricultural and Biological Sciences, Vol. 2, No. 1, 2007, pp. 36-42. http://dx.doi.org/10.3844/ajabssp.2007.36.42 [61] S. A. Deepak, G. Oros, S. G. Sathyanarayana, N. P. Shet- ty, H. S. Shetty and S. Sashikanth, “Antisporulant Activi- ty of Leaf Extracts of Indian Plants against Sclerospora graminicola Causing Downy Mildew Disease of Pearl Millet,” Archives of Phytopathology and Plant Protec- tion, Vol. 38, No. 1, 2005, pp. 31-39. http://dx.doi.org/10.1080/03235400400007558 [62] C. A. Bradley, G. L. Hartman, L. M. Wax and W. L. Pe- dersen, “Influence of Herbicides on Rhizoctonia Root and Hypocotyl Rot of Soybean,” Crop Protection, Vol. 21, No. 8, 2002, pp. 679-687. http://dx.doi.org/10.1016/S0261-2194(02)00021-2 [63] H. Lee, S. E. Ullrich, I. C. Burke, J. Yenish and T. C. Paulitz, “Interactions between the Root Pathogen Rhizo- Open Access AJPS
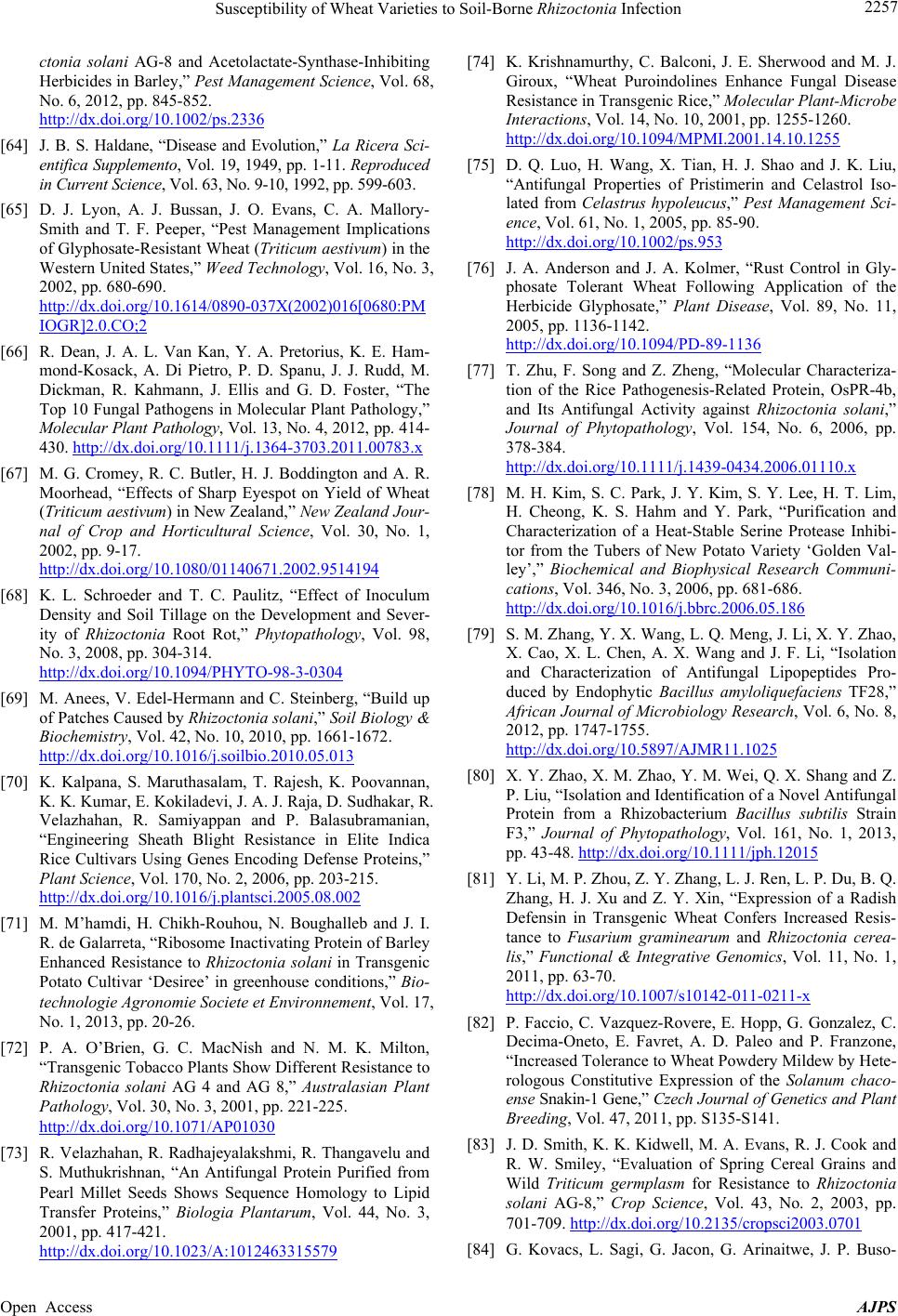 Susceptibility of Wheat Varieties to Soil-Borne Rhizoctonia Infection 2257 ctonia solani AG-8 and Acetolactate-Synthase-Inhibiting Herbicides in Barley,” Pest Management Science, Vol. 68, No. 6, 2012, pp. 845-852. http://dx.doi.org/10.1002/ps.2336 [64] J. B. S. Haldane, “Disease and Evolution,” La Ricera Sci- entifica Supplemento, Vol. 19, 1949, pp. 1-11. Reproduced in Current Science, Vol. 63, No. 9-10, 1992, pp. 599-603. [65] D. J. Lyon, A. J. Bussan, J. O. Evans, C. A. Mallory- Smith and T. F. Peeper, “Pest Management Implications of Glyphosate-Resistant Wheat (Triticum aestivum) in the Western United States,” Weed Technology, Vol. 16, No. 3, 2002, pp. 680-690. http://dx.doi.org/10.1614/0890-037X(2002)016[0680:PM IOGR]2.0.CO;2 [66] R. Dean, J. A. L. Van Kan, Y. A. Pretorius, K. E. Ham- mond-Kosack, A. Di Pietro, P. D. Spanu, J. J. Rudd, M. Dickman, R. Kahmann, J. Ellis and G. D. Foster, “The Top 10 Fungal Pathogens in Molecular Plant Pathology,” Molecular Plant Pathology, Vol. 13, No. 4, 2012, pp. 414- 430. http://dx.doi.org/10.1111/j.1364-3703.2011.00783.x [67] M. G. Cromey, R. C. Butler, H. J. Boddington and A. R. Moorhead, “Effects of Sharp Eyespot on Yield of Wheat (Triticum aestivum) in New Zealand,” New Zealand Jour- nal of Crop and Horticultural Science, Vol. 30, No. 1, 2002, pp. 9-17. http://dx.doi.org/10.1080/01140671.2002.9514194 [68] K. L. Schroeder and T. C. Paulitz, “Effect of Inoculum Density and Soil Tillage on the Development and Sever- ity of Rhizoctonia Root Rot,” Phytopathology, Vol. 98, No. 3, 2008, pp. 304-314. http://dx.doi.org/10.1094/PHYTO-98-3-0304 [69] M. Anees, V. Edel-Hermann and C. Steinberg, “Build up of Patches Caused by Rhizoctonia solani,” Soil Biology & Biochemistry, Vol. 42, No. 10, 2010, pp. 1661-1672. http://dx.doi.org/10.1016/j.soilbio.2010.05.013 [70] K. Kalpana, S. Maruthasalam, T. Rajesh, K. Poovannan, K. K. Kumar, E. Kokiladevi, J. A. J. Raja, D. Sudhakar, R. Velazhahan, R. Samiyappan and P. Balasubramanian, “Engineering Sheath Blight Resistance in Elite Indica Rice Cultivars Using Genes Encoding Defense Proteins,” Plant Science, Vol. 170, No. 2, 2006, pp. 203-215. http://dx.doi.org/10.1016/j.plantsci.2005.08.002 [71] M. M’hamdi, H. Chikh-Rouhou, N. Boughalleb and J. I. R. de Galarreta, “Ribosome Inactivating Protein of Barley Enhanced Resistance to Rhizoctonia solani in Transgenic Potato Cultivar ‘Desiree’ in greenhouse conditions,” Bio- technologie Agronomie Societe et Environnement, Vol. 17, No. 1, 2013, pp. 20-26. [72] P. A. O’Brien, G. C. MacNish and N. M. K. Milton, “Transgenic Tobacco Plants Show Different Resistance to Rhizoctonia solani AG 4 and AG 8,” Australasian Plant Pathology, Vol. 30, No. 3, 2001, pp. 221-225. http://dx.doi.org/10.1071/AP01030 [73] R. Velazhahan, R. Radhajeyalakshmi, R. Thangavelu and S. Muthukrishnan, “An Antifungal Protein Purified from Pearl Millet Seeds Shows Sequence Homology to Lipid Transfer Proteins,” Biologia Plantarum, Vol. 44, No. 3, 2001, pp. 417-421. http://dx.doi.org/10.1023/A:1012463315579 [74] K. Krishnamurthy, C. Balconi, J. E. Sherwood and M. J. Giroux, “Wheat Puroindolines Enhance Fungal Disease Resistance in Transgenic Rice,” Molecular Plant-Microbe Interactions, Vol. 14, No. 10, 2001, pp. 1255-1260. http://dx.doi.org/10.1094/MPMI.2001.14.10.1255 [75] D. Q. Luo, H. Wang, X. Tian, H. J. Shao and J. K. Liu, “Antifungal Properties of Pristimerin and Celastrol Iso- lated from Celastrus hypoleucus,” Pest Management Sci- ence, Vol. 61, No. 1, 2005, pp. 85-90. http://dx.doi.org/10.1002/ps.953 [76] J. A. Anderson and J. A. Kolmer, “Rust Control in Gly- phosate Tolerant Wheat Following Application of the Herbicide Glyphosate,” Plant Disease, Vol. 89, No. 11, 2005, pp. 1136-1142. http://dx.doi.org/10.1094/PD-89-1136 [77] T. Zhu, F. Song and Z. Zheng, “Molecular Characteriza- tion of the Rice Pathogenesis-Related Protein, OsPR-4b, and Its Antifungal Activity against Rhizoctonia solani,” Journal of Phytopathology, Vol. 154, No. 6, 2006, pp. 378-384. http://dx.doi.org/10.1111/j.1439-0434.2006.01110.x [78] M. H. Kim, S. C. Park, J. Y. Kim, S. Y. Lee, H. T. Lim, H. Cheong, K. S. Hahm and Y. Park, “Purification and Characterization of a Heat-Stable Serine Protease Inhibi- tor from the Tubers of New Potato Variety ‘Golden Val- ley’,” Biochemical and Biophysical Research Communi- cations, Vol. 346, No. 3, 2006, pp. 681-686. http://dx.doi.org/10.1016/j.bbrc.2006.05.186 [79] S. M. Zhang, Y. X. Wang, L. Q. Meng, J. Li, X. Y. Zhao, X. Cao, X. L. Chen, A. X. Wang and J. F. Li, “Isolation and Characterization of Antifungal Lipopeptides Pro- duced by Endophytic Bacillus amyloliquefaciens TF28,” African Journal of Microbiology Research, Vol. 6, No. 8, 2012, pp. 1747-1755. http://dx.doi.org/10.5897/AJMR11.1025 [80] X. Y. Zhao, X. M. Zhao, Y. M. Wei, Q. X. Shang and Z. P. Liu, “Isolation and Identification of a Novel Antifungal Protein from a Rhizobacterium Bacillus subtilis Strain F3,” Journal of Phytopathology, Vol. 161, No. 1, 2013, pp. 43-48. http://dx.doi.org/10.1111/jph.12015 [81] Y. Li, M. P. Zhou, Z. Y. Zhang, L. J. Ren, L. P. Du, B. Q. Zhang, H. J. Xu and Z. Y. Xin, “Expression of a Radish Defensin in Transgenic Wheat Confers Increased Resis- tance to Fusarium graminearum and Rhizoctonia cerea- lis,” Functional & Integrative Genomics, Vol. 11, No. 1, 2011, pp. 63-70. http://dx.doi.org/10.1007/s10142-011-0211-x [82] P. Faccio, C. Vazquez-Rovere, E. Hopp, G. Gonzalez, C. Decima-Oneto, E. Favret, A. D. Paleo and P. Franzone, “Increased Tolerance to Wheat Powdery Mildew by Hete- rologous Constitutive Expression of the Solanum chaco- ense Snakin-1 Gene,” Czech Journal of Genetics and Plant Breeding, Vol. 47, 2011, pp. S135-S141. [83] J. D. Smith, K. K. Kidwell, M. A. Evans, R. J. Cook and R. W. Smiley, “Evaluation of Spring Cereal Grains and Wild Triticum germplasm for Resistance to Rhizoctonia solani AG-8,” Crop Science, Vol. 43, No. 2, 2003, pp. 701-709. http://dx.doi.org/10.2135/cropsci2003.0701 [84] G. Kovacs, L. Sagi, G. Jacon, G. Arinaitwe, J. P. Buso- Open Access AJPS
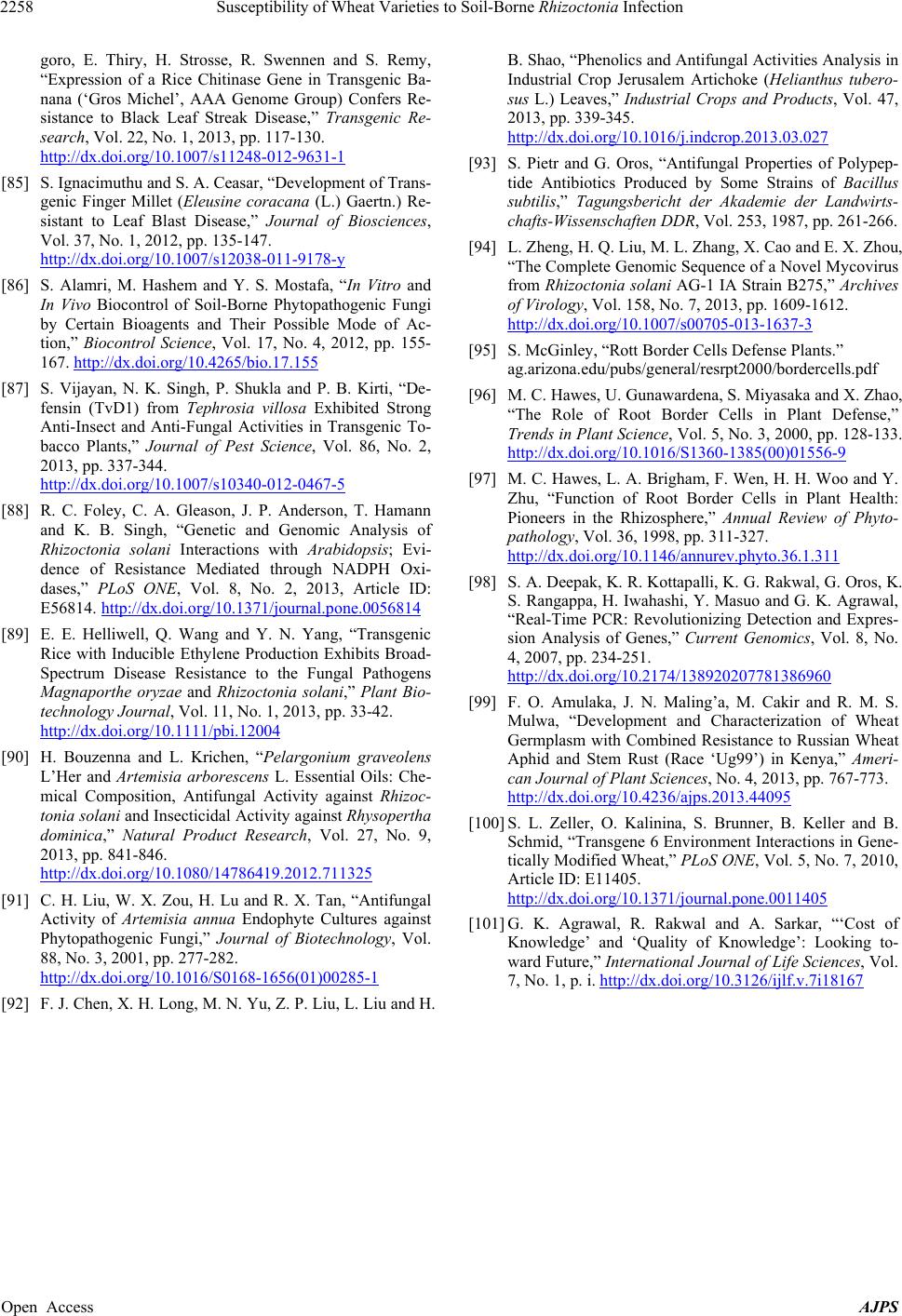 Susceptibility of Wheat Varieties to Soil-Borne Rhizoctonia Infection Open Access AJPS 2258 goro, E. Thiry, H. Strosse, R. Swennen and S. Remy, “Expression of a Rice Chitinase Gene in Transgenic Ba- nana (‘Gros Michel’, AAA Genome Group) Confers Re- sistance to Black Leaf Streak Disease,” Transgenic Re- search, Vol. 22, No. 1, 2013, pp. 117-130. http://dx.doi.org/10.1007/s11248-012-9631-1 [85] S. Ignacimuthu and S. A. Ceasar, “Development of Trans- genic Finger Millet (Eleusine coracana (L.) Gaertn.) Re- sistant to Leaf Blast Disease,” Journal of Biosciences, Vol. 37, No. 1, 2012, pp. 135-147. http://dx.doi.org/10.1007/s12038-011-9178-y [86] S. Alamri, M. Hashem and Y. S. Mostafa, “In Vitro and In Vivo Biocontrol of Soil-Borne Phytopathogenic Fungi by Certain Bioagents and Their Possible Mode of Ac- tion,” Biocontrol Science, Vol. 17, No. 4, 2012, pp. 155- 167. http://dx.doi.org/10.4265/bio.17.155 [87] S. Vijayan, N. K. Singh, P. Shukla and P. B. Kirti, “De- fensin (TvD1) from Tephrosia villosa Exhibited Strong Anti-Insect and Anti-Fungal Activities in Transgenic To- bacco Plants,” Journal of Pest Science, Vol. 86, No. 2, 2013, pp. 337-344. http://dx.doi.org/10.1007/s10340-012-0467-5 [88] R. C. Foley, C. A. Gleason, J. P. Anderson, T. Hamann and K. B. Singh, “Genetic and Genomic Analysis of Rhizoctonia solani Interactions with Arabidopsis; Evi- dence of Resistance Mediated through NADPH Oxi- dases,” PLoS ONE, Vol. 8, No. 2, 2013, Article ID: E56814.http://dx.doi.org/10.1371/journal.pone.0056814 [89] E. E. Helliwell, Q. Wang and Y. N. Yang, “Transgenic Rice with Inducible Ethylene Production Exhibits Broad- Spectrum Disease Resistance to the Fungal Pathogens Magnaporthe oryzae and Rhizoctonia solani,” Plant Bio- technology Journal, Vol. 11, No. 1, 2013, pp. 33-42. http://dx.doi.org/10.1111/pbi.12004 [90] H. Bouzenna and L. Krichen, “Pelargonium graveolens L’Her and Artemisia arborescens L. Essential Oils: Che- mical Composition, Antifungal Activity against Rhizoc- tonia solani and Insecticidal Activity against Rhysopertha dominica,” Natural Product Research, Vol. 27, No. 9, 2013, pp. 841-846. http://dx.doi.org/10.1080/14786419.2012.711325 [91] C. H. Liu, W. X. Zou, H. Lu and R. X. Tan, “Antifungal Activity of Artemisia annua Endophyte Cultures against Phytopathogenic Fungi,” Journal of Biotechnology, Vol. 88, No. 3, 2001, pp. 277-282. http://dx.doi.org/10.1016/S0168-1656(01)00285-1 [92] F. J. Chen, X. H. Long, M. N. Yu, Z. P. Liu, L. Liu and H. B. Shao, “Phenolics and Antifungal Activities Analysis in Industrial Crop Jerusalem Artichoke (Helianthus tubero- sus L.) Leaves,” Industrial Crops and Products, Vol. 47, 2013, pp. 339-345. http://dx.doi.org/10.1016/j.indcrop.2013.03.027 [93] S. Pietr and G. Oros, “Antifungal Properties of Polypep- tide Antibiotics Produced by Some Strains of Bacillus subtilis,” Tagungsbericht der Akademie der Landwirts- chafts-Wissenschaften DDR, Vol. 253, 1987, pp. 261-266. [94] L. Zheng, H. Q. Liu, M. L. Zhang, X. Cao and E. X. Zhou, “The Complete Genomic Sequence of a Novel Mycovirus from Rhizoctonia solani AG-1 IA Strain B275,” Archives of Virology, Vol. 158, No. 7, 2013, pp. 1609-1612. http://dx.doi.org/10.1007/s00705-013-1637-3 [95] S. McGinley, “Rott Border Cells Defense Plants.” ag.arizona.edu/pubs/general/resrpt2000/bordercells.pdf [96] M. C. Hawes, U. Gunawardena, S. Miyasaka and X. Zhao, “The Role of Root Border Cells in Plant Defense,” Trends in Plant Science, Vol. 5, No. 3, 2000, pp. 128-133. http://dx.doi.org/10.1016/S1360-1385(00)01556-9 [97] M. C. Hawes, L. A. Brigham, F. Wen, H. H. Woo and Y. Zhu, “Function of Root Border Cells in Plant Health: Pioneers in the Rhizosphere,” Annual Review of Phyto- pathology, Vol. 36, 1998, pp. 311-327. http://dx.doi.org/10.1146/annurev.phyto.36.1.311 [98] S. A. Deepak, K. R. Kottapalli, K. G. Rakwal, G. Oros, K. S. Rangappa, H. Iwahashi, Y. Masuo and G. K. Agrawal, “Real-Time PCR: Revolutionizing Detection and Expres- sion Analysis of Genes,” Current Genomics, Vol. 8, No. 4, 2007, pp. 234-251. http://dx.doi.org/10.2174/138920207781386960 [99] F. O. Amulaka, J. N. Maling’a, M. Cakir and R. M. S. Mulwa, “Development and Characterization of Wheat Germplasm with Combined Resistance to Russian Wheat Aphid and Stem Rust (Race ‘Ug99’) in Kenya,” Ameri- can Journal of Plant Sciences, No. 4, 2013, pp. 767-773. http://dx.doi.org/10.4236/ajps.2013.44095 [100] S. L. Zeller, O. Kalinina, S. Brunner, B. Keller and B. Schmid, “Transgene 6 Environment Interactions in Gene- tically Modified Wheat,” PLoS ONE, Vol. 5, No. 7, 2010, Article ID: E11405. http://dx.doi.org/10.1371/journal.pone.0011405 [101] G. K. Agrawal, R. Rakwal and A. Sarkar, “‘Cost of Knowledge’ and ‘Quality of Knowledge’: Looking to- ward Future,” International Journal of Life Sciences, Vol. 7, No. 1, p. i. http://dx.doi.org/10.3126/ijlf.v.7i18167
|