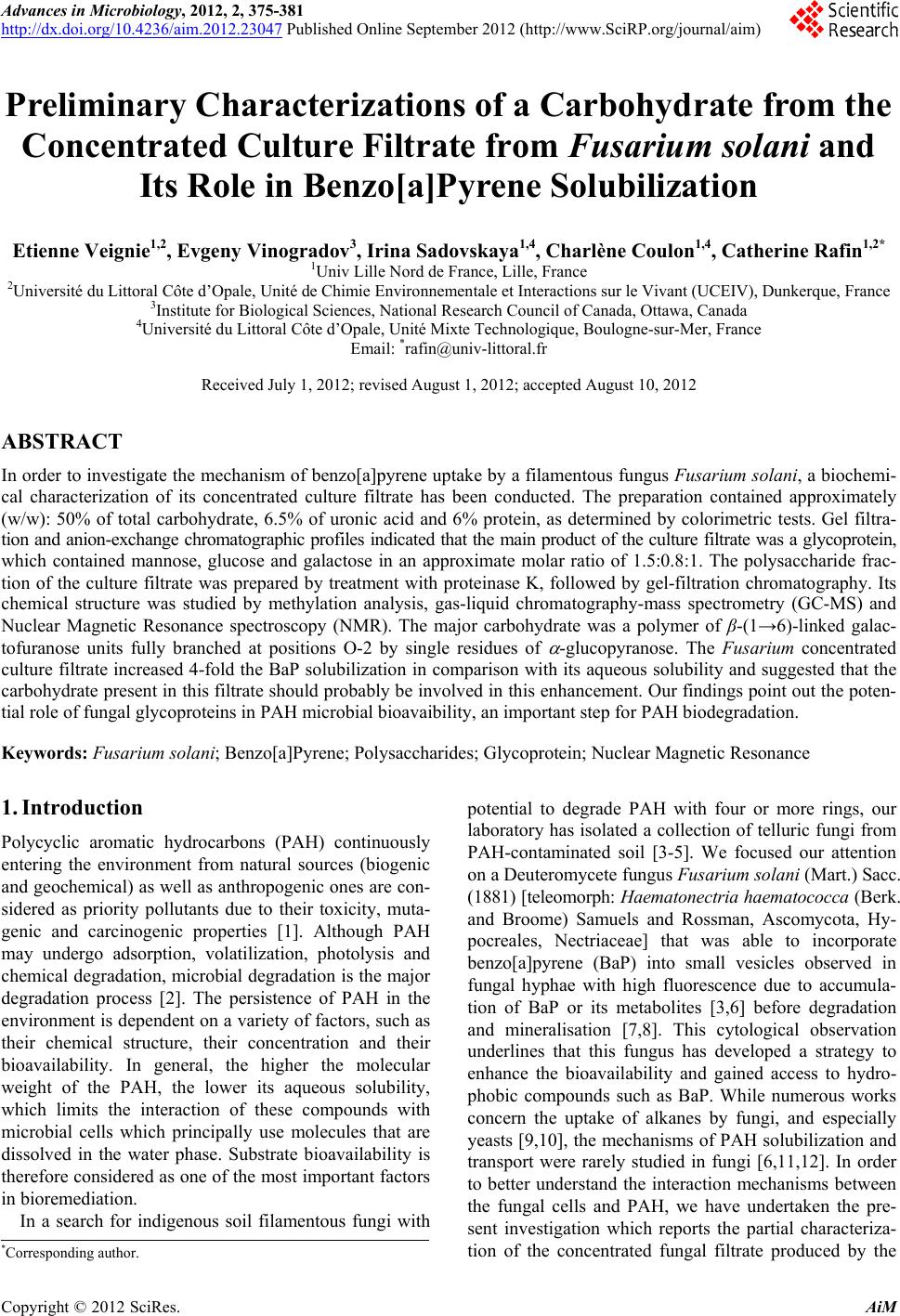 Advances in Microbiology, 2012, 2, 375-381 http://dx.doi.org/10.4236/aim.2012.23047 Published Online September 2012 (http://www.SciRP.org/journal/aim) Preliminary Characterizations of a Carbohydrate from the Concentrated Culture Filtrate from Fusarium solani and Its Role in Benzo[a]Pyrene Solubilization Etienne Veignie1,2, Evgeny Vinogradov3, Irina Sadovskaya1,4, Charlène Coulon1,4, Catherine Rafin1,2* 1Univ Lille Nord de France, Lille, France 2Université du Littoral Côte d’Opale, Unité de Chimie Environnementale et Interactions sur le Vivant (UCEIV), Dunkerque, France 3Institute for Biological Sciences, National Research Council of Canada, Ottawa, Canada 4Université du Littoral Côte d’Opale, Unité Mixte Technologique, Boulogne-sur-Mer, France Email: *rafin@univ-littoral.fr Received July 1, 2012; revised August 1, 2012; accepted August 10, 2012 ABSTRACT In order to investigate the mechanism of benzo[a]pyrene uptake by a filamentous fungus Fusarium solani, a biochemi- cal characterization of its concentrated culture filtrate has been conducted. The preparation contained approximately (w/w): 50% of total carbohydrate, 6.5% of uronic acid and 6% protein, as determined by colorimetric tests. Gel filtra- tion and anion-exchange chromatographic profiles indicated that the main product of the culture filtrate was a glycoprotein, which contained mannose, glucose and galactose in an approximate molar ratio of 1.5:0.8:1. The polysaccharide frac- tion of the culture filtrate was prepared by treatment with proteinase K, followed by gel-filtration chromatography. Its chemical structure was studied by methylation analysis, gas-liquid chromatography-mass spectrometry (GC-MS) and Nuclear Magnetic Resonance spectroscopy (NMR). The major carbohydrate was a polymer of β-(1→6)-linked galac- tofuranose units fully branched at positions O-2 by single residues of -glucopyranose. The Fusarium concentrated culture filtrate increased 4-fold the BaP solubilization in comparison with its aqueous solubility and suggested that the carbohydrate present in this filtrate should probably be involved in this enhancement. Our findings point out the poten- tial role of fungal glycoproteins in PAH microbial bioavaibility, an important step for PAH biodegradation. Keywords: Fusarium solani; Benzo[a]Pyrene; Polysaccharides; Glycoprotein; Nuclear Magnetic Resonance 1. Introduction Polycyclic aromatic hydrocarbons (PAH) continuously entering the environment from natural sources (biogenic and geochemical) as well as anthropogenic ones are con- sidered as priority pollutants due to their toxicity, muta- genic and carcinogenic properties [1]. Although PAH may undergo adsorption, volatilization, photolysis and chemical degradation, microbial degradation is the major degradation process [2]. The persistence of PAH in the environment is dependent on a variety of factors, such as their chemical structure, their concentration and their bioavailability. In general, the higher the molecular weight of the PAH, the lower its aqueous solubility, which limits the interaction of these compounds with microbial cells which principally use molecules that are dissolved in the water phase. Substrate bioavailability is therefore considered as one of the most important factors in bioremediation. In a search for indigenous soil filamentous fungi with potential to degrade PAH with four or more rings, our laboratory has isolated a collection of telluric fungi from PAH-contaminated soil [3-5]. We focused our attention on a Deuteromycete fungus Fusarium solani (Mart.) Sacc. (1881) [teleomorph: Haematonectria haematococca (Berk. and Broome) Samuels and Rossman, Ascomycota, Hy- pocreales, Nectriaceae] that was able to incorporate benzo[a]pyrene (BaP) into small vesicles observed in fungal hyphae with high fluorescence due to accumula- tion of BaP or its metabolites [3,6] before degradation and mineralisation [7,8]. This cytological observation underlines that this fungus has developed a strategy to enhance the bioavailability and gained access to hydro- phobic compounds such as BaP. While numerous works concern the uptake of alkanes by fungi, and especially yeasts [9,10], the mechanisms of PAH solubilization and transport were rarely studied in fungi [6,11,12]. In order to better understand the interaction mechanisms between the fungal cells and PAH, we have undertaken the pre- sent investigation which reports the partial characteriza- tion of the concentrated fungal filtrate produced by the *Corresponding author. C opyright © 2012 SciRes. AiM
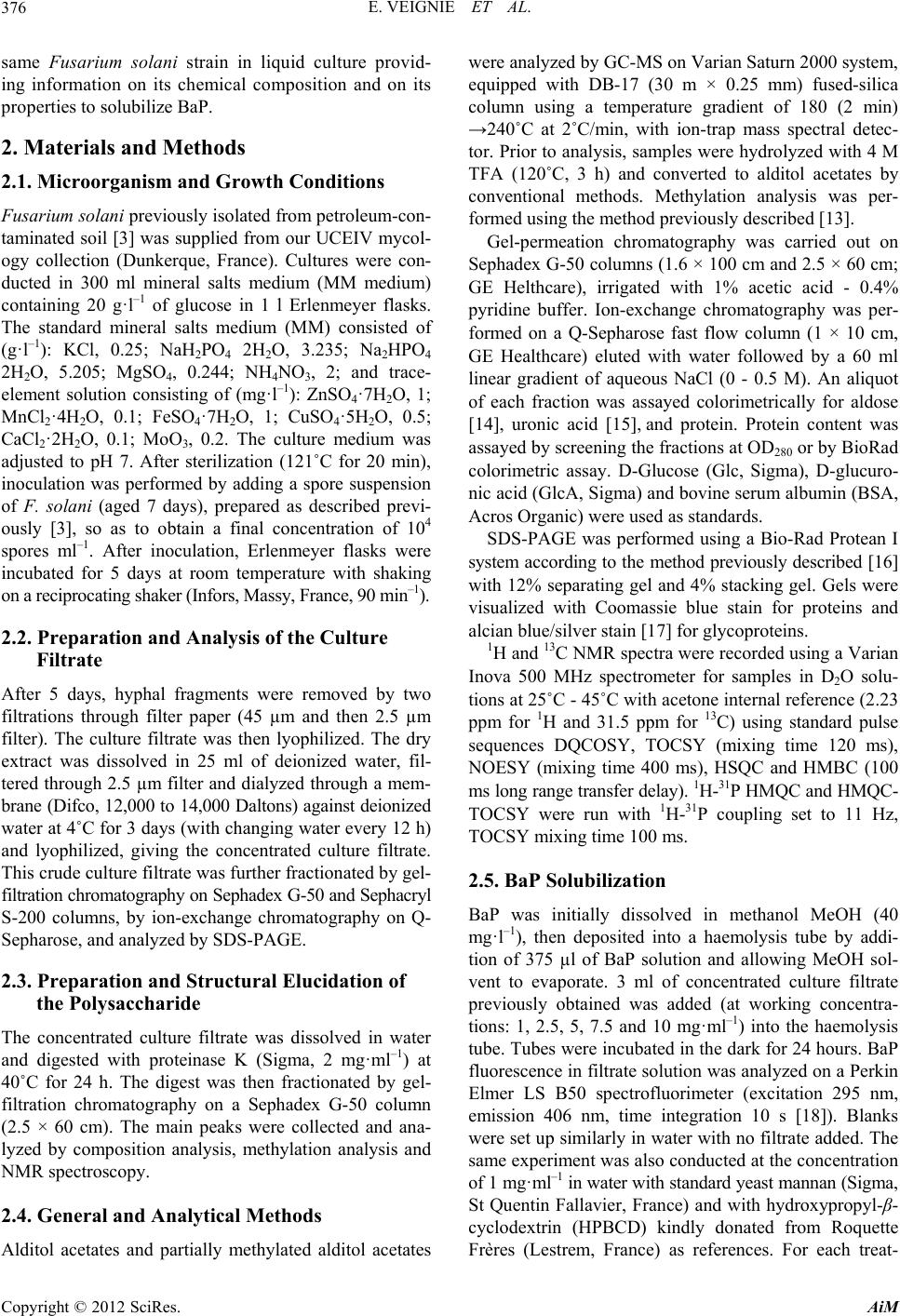 E. VEIGNIE ET AL. 376 same Fusarium solani strain in liquid culture provid- ing information on its chemical composition and on its properties to solubilize BaP. 2. Materials and Methods 2.1. Microorganism and Growth Conditions Fusarium solani previously isolated from petroleum-con- taminated soil [3] was supplied from our UCEIV mycol- ogy collection (Dunkerque, France). Cultures were con- ducted in 300 ml mineral salts medium (MM medium) containing 20 g·l–1 of glucose in 1 l Erlenmeyer flasks. The standard mineral salts medium (MM) consisted of (g·l–1): KCl, 0.25; NaH2PO4 2H2O, 3.235; Na2HPO4 2H2O, 5.205; MgSO4, 0.244; NH4NO3, 2; and trace- element solution consisting of (mg·l–1): ZnSO4·7H2O, 1; MnCl2·4H2O, 0.1; FeSO4·7H2O, 1; CuSO4·5H2O, 0.5; CaCl2·2H2O, 0.1; MoO3, 0.2. The culture medium was adjusted to pH 7. After sterilization (121˚C for 20 min), inoculation was performed by adding a spore suspension of F. solani (aged 7 days), prepared as described previ- ously [3], so as to obtain a final concentration of 104 spores ml–1. After inoculation, Erlenmeyer flasks were incubated for 5 days at room temperature with shaking on a reciprocating shaker (Infors, Massy, France, 90 min–1). 2.2. Preparation and Analysis of the Culture Filtrate After 5 days, hyphal fragments were removed by two filtrations through filter paper (45 µm and then 2.5 µm filter). The culture filtrate was then lyophilized. The dry extract was dissolved in 25 ml of deionized water, fil- tered through 2.5 µm filter and dialyzed through a mem- brane (Difco, 12,000 to 14,000 Daltons) against deionized water at 4˚C for 3 days (with changing water every 12 h) and lyophilized, giving the concentrated culture filtrate. This crude culture filtrate was further fractionated by gel- filtration chromatography on Sephadex G-50 and Sephacryl S-200 columns, by ion-exchange chromatography on Q- Sepharose, and analyzed by SDS-PAGE. 2.3. Preparation and Structural Elucidation of the Polysaccharide The concentrated culture filtrate was dissolved in water and digested with proteinase K (Sigma, 2 mg·ml–1) at 40˚C for 24 h. The digest was then fractionated by gel- filtration chromatography on a Sephadex G-50 column (2.5 × 60 cm). The main peaks were collected and ana- lyzed by composition analysis, methylation analysis and NMR spectroscopy. 2.4. General and Analytical Methods Alditol acetates and partially methylated alditol acetates were analyzed by GC-MS on Varian Saturn 2000 system, equipped with DB-17 (30 m × 0.25 mm) fused-silica column using a temperature gradient of 180 (2 min) →240˚C at 2˚C/min, with ion-trap mass spectral detec- tor. Prior to analysis, samples were hydrolyzed with 4 M TFA (120˚C, 3 h) and converted to alditol acetates by conventional methods. Methylation analysis was per- formed using the method previously described [13]. Gel-permeation chromatography was carried out on Sephadex G-50 columns (1.6 × 100 cm and 2.5 × 60 cm; GE Helthcare), irrigated with 1% acetic acid - 0.4% pyridine buffer. Ion-exchange chromatography was per- formed on a Q-Sepharose fast flow column (1 × 10 cm, GE Healthcare) eluted with water followed by a 60 ml linear gradient of aqueous NaCl (0 - 0.5 M). An aliquot of each fraction was assayed colorimetrically for aldose [14], uronic acid [15], and protein. Protein content was assayed by screening the fractions at OD280 or by BioRad colorimetric assay. D-Glucose (Glc, Sigma), D-glucuro- nic acid (GlcA, Sigma) and bovine serum albumin (BSA, Acros Organic) were used as standards. SDS-PAGE was performed using a Bio-Rad Protean I system according to the method previously described [16] with 12% separating gel and 4% stacking gel. Gels were visualized with Coomassie blue stain for proteins and alcian blue/silver stain [17] for glycoproteins. 1H and 13C NMR spectra were recorded using a Varian Inova 500 MHz spectrometer for samples in D2O solu- tions at 25˚C - 45˚C with acetone internal reference (2.23 ppm for 1H and 31.5 ppm for 13C) using standard pulse sequences DQCOSY, TOCSY (mixing time 120 ms), NOESY (mixing time 400 ms), HSQC and HMBC (100 ms long range transfer delay). 1H-31P HMQC and HMQC- TOCSY were run with 1H-31P coupling set to 11 Hz, TOCSY mixing time 100 ms. 2.5. BaP Solubilization BaP was initially dissolved in methanol MeOH (40 mg· l–1), then deposited into a haemolysis tube by addi- tion of 375 µl of BaP solution and allowing MeOH sol- vent to evaporate. 3 ml of concentrated culture filtrate previously obtained was added (at working concentra- tions: 1, 2.5, 5, 7.5 and 10 mg·ml–1) into the haemolysis tube. Tubes were incubated in the dark for 24 hours. BaP fluorescence in filtrate solution was analyzed on a Perkin Elmer LS B50 spectrofluorimeter (excitation 295 nm, emission 406 nm, time integration 10 s [18]). Blanks were set up similarly in water with no filtrate added. The same experiment was also conducted at the concentration of 1 mg·ml–1 in water with standard yeast mannan (Sigma, St Quentin Fallavier, France) and with hydroxypropyl-β- cyclodextrin (HPBCD) kindly donated from Roquette Frères (Lestrem, France) as references. For each treat- Copyright © 2012 SciRes. AiM
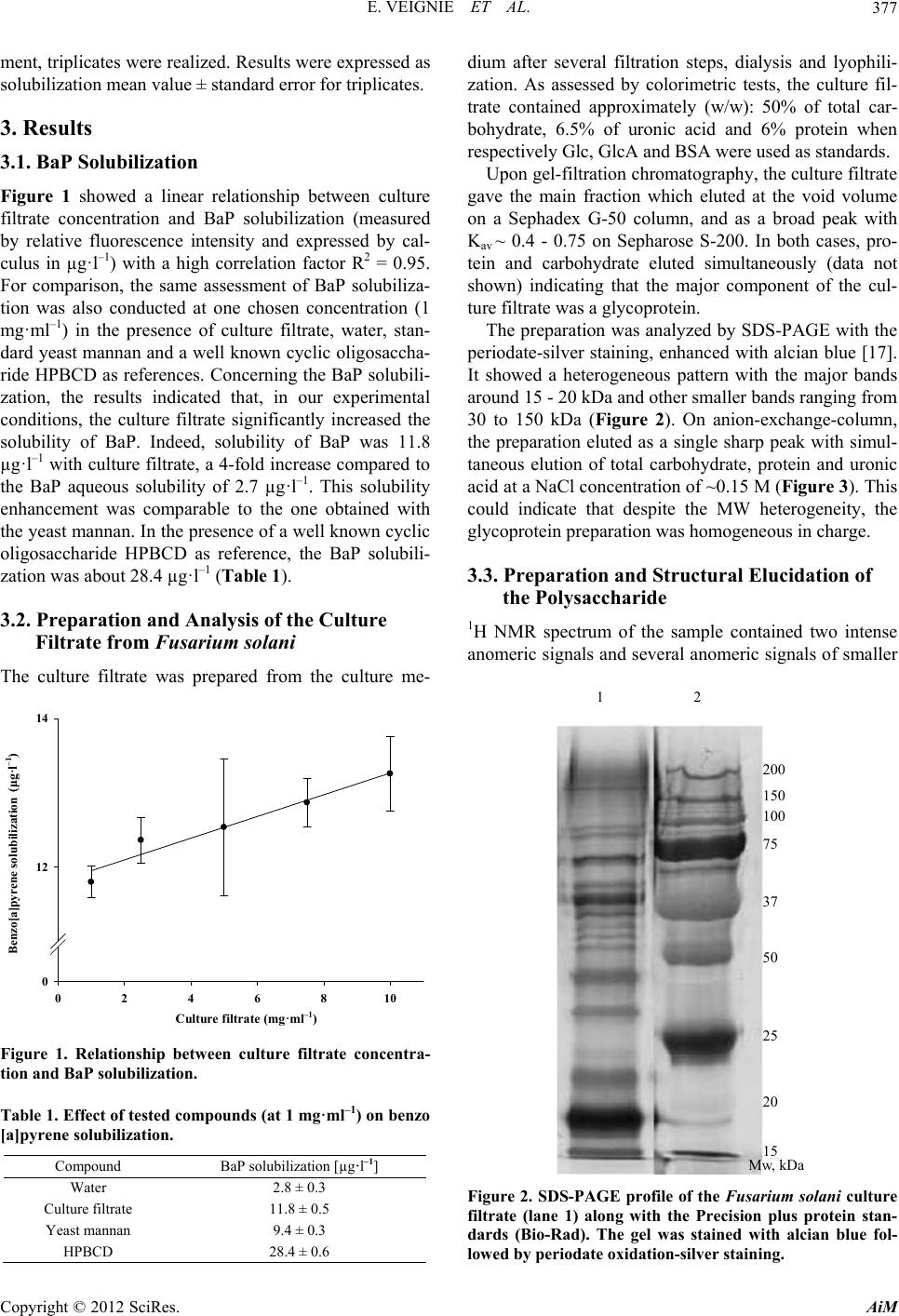 E. VEIGNIE ET AL. 377 ment, triplicates were realized. Results were expressed as solubilization mean value ± standard error for triplicates. 3. Results 3.1. BaP Solubilization Figure 1 showed a linear relationship between culture filtrate concentration and BaP solubilization (measured by relative fluorescence intensity and expressed by cal- culus in µg·l–1) with a high correlation factor R2 = 0.95. For comparison, the same assessment of BaP solubiliza- tion was also conducted at one chosen concentration (1 mg· ml –1) in the presence of culture filtrate, water, stan- dard yeast mannan and a well known cyclic oligosaccha- ride HPBCD as references. Concerning the BaP solubili- zation, the results indicated that, in our experimental conditions, the culture filtrate significantly increased the solubility of BaP. Indeed, solubility of BaP was 11.8 µg·l–1 with culture filtrate, a 4-fold increase compared to the BaP aqueous solubility of 2.7 µg·l–1. This solubility enhancement was comparable to the one obtained with the yeast mannan. In the presence of a well known cyclic oligosaccharide HPBCD as reference, the BaP solubili- zation was about 28.4 µg·l–1 (Table 1). 3.2. Preparation and Analysis of the Culture Filtrate from Fusarium solani The culture filtrate was prepared from the culture me- Benzo[a]pyrene solubilization (µg l -1 ) y = 0.1464 x + 5.0127 R2 = 0.95 Culture filtrate (mg ml -1 ) 0246810 0 12 14 Culture filtrate (mg·ml –1 ) (μg·l –1 ) Figure 1. Relationship between culture filtrate concentra- tion and BaP solubilization. Table 1. Effect of tested compounds (at 1 mg·ml–1) on benzo [a]pyrene solubilization. Compound BaP solubilization [µg·l–1] Water 2.8 ± 0.3 Culture filtrate 11.8 ± 0.5 Yeast mannan 9.4 ± 0.3 HPBCD 28.4 ± 0.6 dium after several filtration steps, dialysis and lyophili- zation. As assessed by colorimetric tests, the culture fil- trate contained approximately (w/w): 50% of total car- bohydrate, 6.5% of uronic acid and 6% protein when respectively Glc, GlcA and BSA were used as standards. Upon gel-filtration chromatography, the culture filtrate gave the main fraction which eluted at the void volume on a Sephadex G-50 column, and as a broad peak with Kav ~ 0.4 - 0.75 on Sepharose S-200. In both cases, pro- tein and carbohydrate eluted simultaneously (data not shown) indicating that the major component of the cul- ture filtrate was a glycoprotein. The preparation was analyzed by SDS-PAGE with the periodate-silver staining, enhanced with alcian blue [17]. It showed a heterogeneous pattern with the major bands around 15 - 20 kDa and other smaller bands ranging from 30 to 150 kDa (Figure 2). On anion-exchange-column, the preparation eluted as a single sharp peak with simul- taneous elution of total carbohydrate, protein and uronic acid at a NaCl concentration of ~0.15 M (Figure 3). This could indicate that despite the MW heterogeneity, the glycoprotein preparation was homogeneous in charge. 3.3. Preparation and Structural Elucidation of the Polysaccharide 1H NMR spectrum of the sample contained two intense anomeric signals and several anomeric signals of smaller 1 2 200 150 100 75 37 50 25 20 15 Mw, kDa Figure 2. SDS-PAGE profile of the Fusarium solani culture filtrate (lane 1) along with the Precision plus protein stan- dards (Bio-Rad). The gel was stained with alcian blue fol- lowed by periodate oxidation-silver staining. Copyright © 2012 SciRes. AiM
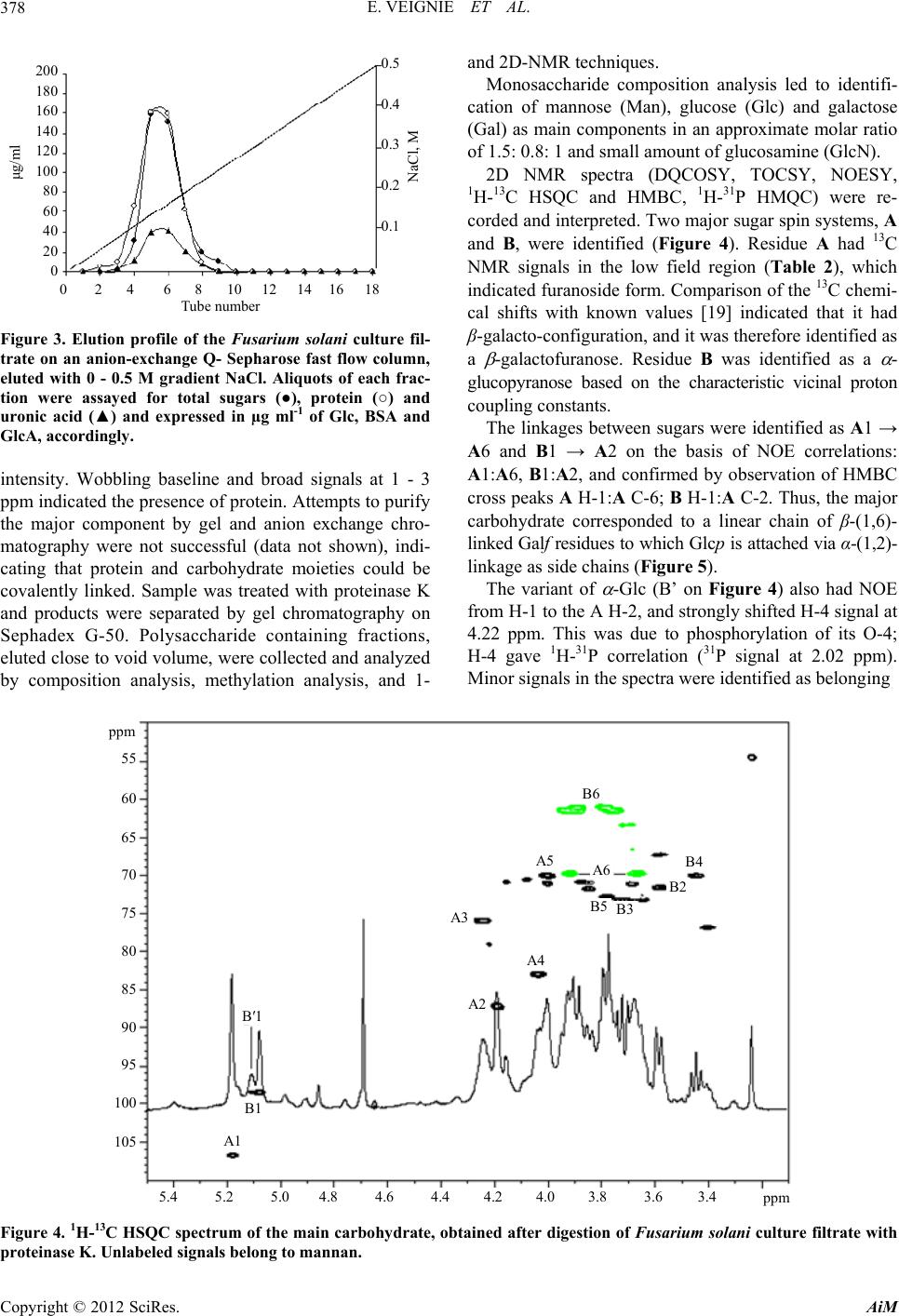 E. VEIGNIE ET AL. Copyright © 2012 SciRes. AiM 378 and 2D-NMR techniques. μg/ml 0.5 0.4 0.3 0.2 0.1 NaCl, M 0 2 4 6 8 10 12 14 16 18 Tube number 200 180 160 140 120 100 80 60 40 20 0 Monosaccharide composition analysis led to identifi- cation of mannose (Man), glucose (Glc) and galactose (Gal) as main components in an approximate molar ratio of 1.5: 0.8: 1 and small amount of glucosamine (GlcN). 2D NMR spectra (DQCOSY, TOCSY, NOESY, 1H-13C HSQC and HMBC, 1H-31P HMQC) were re- corded and interpreted. Two major sugar spin systems, A and B, were identified (Figure 4). Residue A had 13C NMR signals in the low field region (Table 2), which indicated furanoside form. Comparison of the 13C chemi- cal shifts with known values [19] indicated that it had β-galacto-configuration, and it was therefore identified as a -galactofuranose. Residue B was identified as a - glucopyranose based on the characteristic vicinal proton coupling constants. Figure 3. Elution profile of the Fusarium solani culture fil- trate on an anion-exchange Q- Sepharose fast flow column, eluted with 0 - 0.5 M gradient NaCl. Aliquots of each frac- tion were assayed for total sugars (●), protein (○) and uronic acid (▲) and expressed in µg ml-1 of Glc, BSA and GlcA, accordingly. The linkages between sugars were identified as A1 → A6 and B1 → A2 on the basis of NOE correlations: A1:A6, B1:A2, and confirmed by observation of HMBC cross peaks A H-1:A C-6; B H-1:A C-2. Thus, the major carbohydrate corresponded to a linear chain of β-(1,6)- linked Galf residues to which Glcp is attached via α-(1,2)- linkage as side chains (Figure 5). intensity. Wobbling baseline and broad signals at 1 - 3 ppm indicated the presence of protein. Attempts to purify the major component by gel and anion exchange chro- matography were not successful (data not shown), indi- cating that protein and carbohydrate moieties could be covalently linked. Sample was treated with proteinase K and products were separated by gel chromatography on Sephadex G-50. Polysaccharide containing fractions, eluted close to void volume, were collected and analyzed by composition analysis, methylation analysis, and 1- The variant of -Glc (B’ on Figure 4) also had NOE from H-1 to the A H-2, and strongly shifted H-4 signal at 4.22 ppm. This was due to phosphorylation of its O-4; H-4 gave 1H-31P correlation (31P signal at 2.02 ppm). Minor signals in the spectra were identified as belonging ppm 55 60 65 70 75 80 85 90 95 100 105 B6 5.4 5.2 5.0 4.8 4.6 4.4 4.2 4.0 3.8 3.6 3.4 A1 ppm A2 A3 A4 A5 B4 A6 B2 B5 B3 B 1 B1 Figure 4. 1H-13C HSQC spectrum of the main carbohydrate, obtained after digestion of Fusarium solani culture filtrate with proteinase K. Unlabeled signals belong to mannan.
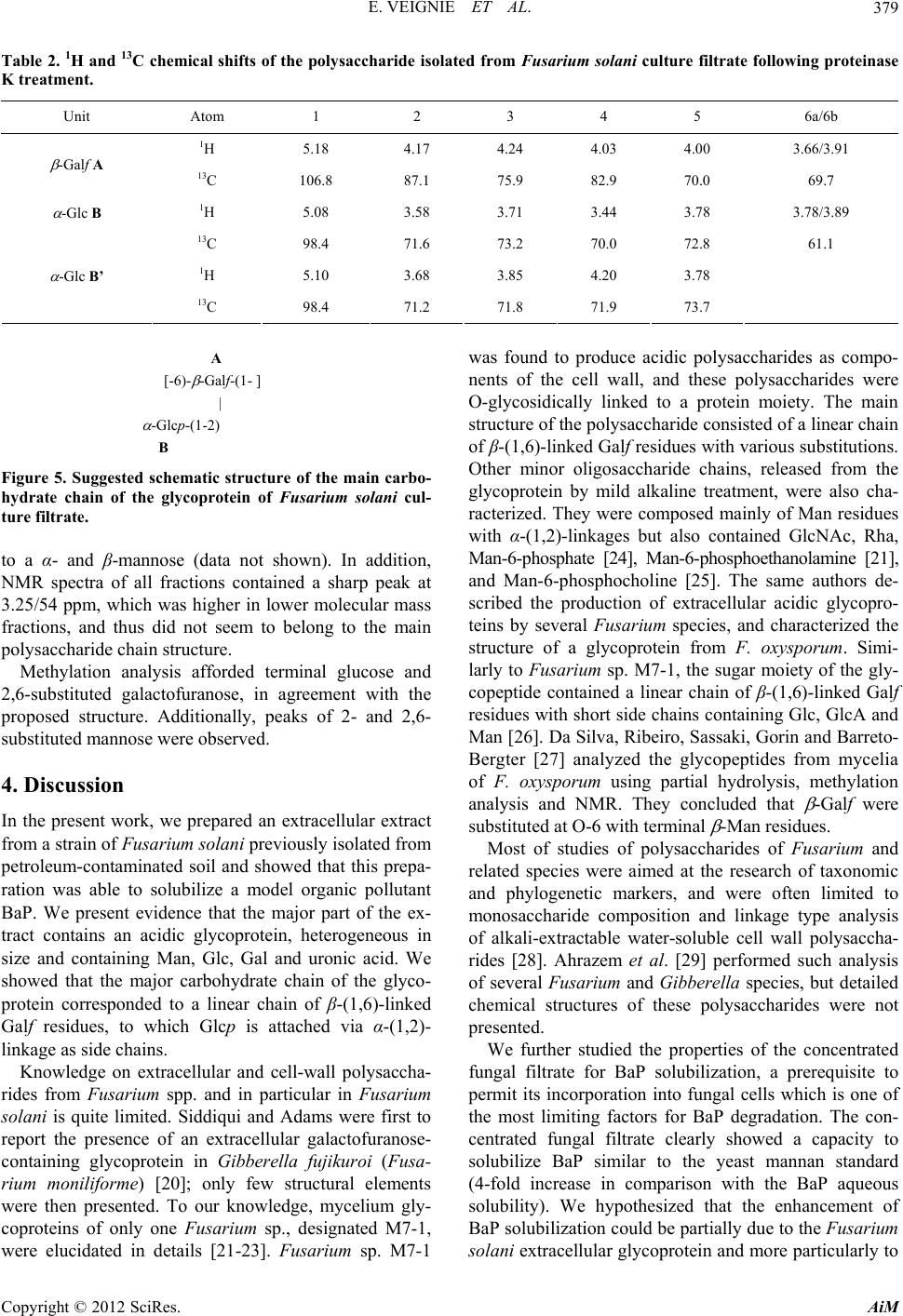 E. VEIGNIE ET AL. 379 Table 2. 1H and 13C chemical shifts of the polysaccharide isolated from Fusarium solani culture filtrate following proteinase K treatment. Unit Atom 1 2 3 4 5 6a/6b 1H 5.18 4.17 4.24 4.03 4.00 3.66/3.91 -Galf A 13C 106.8 87.1 75.9 82.9 70.0 69.7 -Glc B 1H 5.08 3.58 3.71 3.44 3.78 3.78/3.89 13C 98.4 71.6 73.2 70.0 72.8 61.1 1H 5.10 3.68 3.85 4.20 3.78 -Glc B’ 13C 98.4 71.2 71.8 71.9 73.7 A [-6)- -Galf-(1- ] | -Glcp-(1-2) B Figure 5. Suggested schematic structure of the main carbo- hydrate chain of the glycoprotein of Fusarium solani cul- ture filtrate. to a α- and β-mannose (data not shown). In addition, NMR spectra of all fractions contained a sharp peak at 3.25/54 ppm, which was higher in lower molecular mass fractions, and thus did not seem to belong to the main polysaccharide chain structure. Methylation analysis afforded terminal glucose and 2,6-substituted galactofuranose, in agreement with the proposed structure. Additionally, peaks of 2- and 2,6- substituted mannose were observed. 4. Discussion In the present work, we prepared an extracellular extract from a strain of Fusarium solani previously isolated from petroleum-contaminated soil and showed that this prepa- ration was able to solubilize a model organic pollutant BaP. We present evidence that the major part of the ex- tract contains an acidic glycoprotein, heterogeneous in size and containing Man, Glc, Gal and uronic acid. We showed that the major carbohydrate chain of the glyco- protein corresponded to a linear chain of β-(1,6)-linked Galf residues, to which Glcp is attached via α-(1,2)- linkage as side chains. Knowledge on extracellular and cell-wall polysaccha- rides from Fusarium spp. and in particular in Fusarium solani is quite limited. Siddiqui and Adams were first to report the presence of an extracellular galactofuranose- containing glycoprotein in Gibberella fujikuroi (Fusa- rium moniliforme) [20]; only few structural elements were then presented. To our knowledge, mycelium gly- coproteins of only one Fusarium sp., designated M7-1, were elucidated in details [21-23]. Fusarium sp. M7-1 was found to produce acidic polysaccharides as compo- nents of the cell wall, and these polysaccharides were O-glycosidically linked to a protein moiety. The main structure of the polysaccharide consisted of a linear chain of β-(1,6)-linked Galf residues with various substitutions. Other minor oligosaccharide chains, released from the glycoprotein by mild alkaline treatment, were also cha- racterized. They were composed mainly of Man residues with α-(1,2)-linkages but also contained GlcNAc, Rha, Man-6-phosphate [24], Man-6-phosphoethanolamine [21], and Man-6-phosphocholine [25]. The same authors de- scribed the production of extracellular acidic glycopro- teins by several Fusarium species, and characterized the structure of a glycoprotein from F. oxysporum. Simi- larly to Fusar ium sp. M7-1, the sugar moiety of the gly- copeptide contained a linear chain of β-(1,6)-linked Galf residues with short side chains containing Glc, GlcA and Man [26]. Da Silva, Ribeiro, Sassaki, Gorin and Barreto- Bergter [27] analyzed the glycopeptides from mycelia of F. oxysporum using partial hydrolysis, methylation analysis and NMR. They concluded that -Galf were substituted at O-6 with terminal -Man residues. Most of studies of polysaccharides of Fusa rium and related species were aimed at the research of taxonomic and phylogenetic markers, and were often limited to monosaccharide composition and linkage type analysis of alkali-extractable water-soluble cell wall polysaccha- rides [28]. Ahrazem et al. [29] performed such analysis of several Fusarium and Gibberella species, but detailed chemical structures of these polysaccharides were not presented. We further studied the properties of the concentrated fungal filtrate for BaP solubilization, a prerequisite to permit its incorporation into fungal cells which is one of the most limiting factors for BaP degradation. The con- centrated fungal filtrate clearly showed a capacity to solubilize BaP similar to the yeast mannan standard (4-fold increase in comparison with the BaP aqueous solubility). We hypothesized that the enhancement of BaP solubilization could be partially due to the Fusarium solani extracellular glycoprotein and more particularly to Copyright © 2012 SciRes. AiM
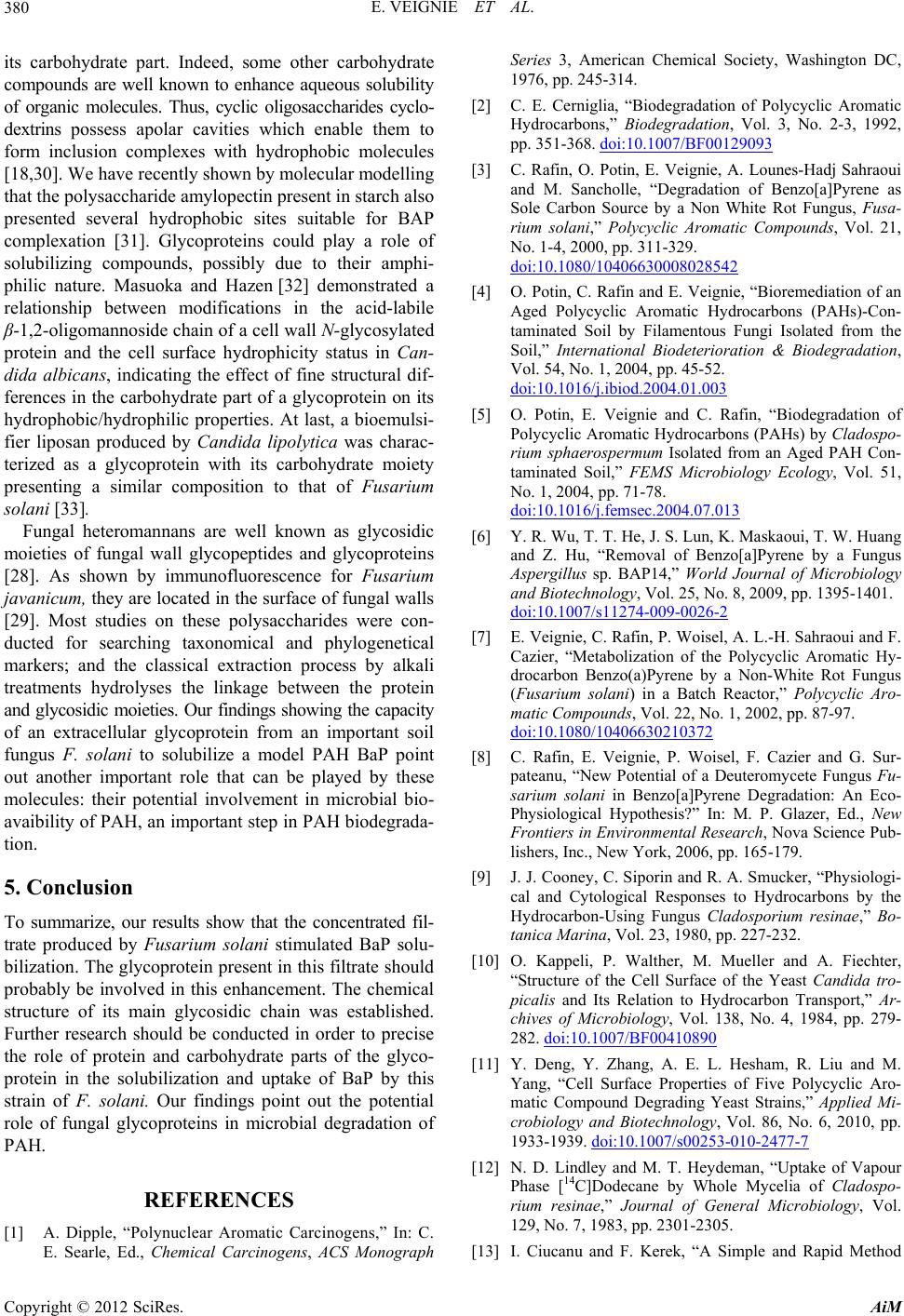 E. VEIGNIE ET AL. 380 its carbohydrate part. Indeed, some other carbohydrate compounds are well known to enhance aqueous solubility of organic molecules. Thus, cyclic oligosaccharides cyclo- dextrins possess apolar cavities which enable them to form inclusion complexes with hydrophobic molecules [18,30]. We have recently shown by molecular modelling that the polysaccharide amylopectin present in starch also presented several hydrophobic sites suitable for BAP complexation [31]. Glycoproteins could play a role of solubilizing compounds, possibly due to their amphi- philic nature. Masuoka and Hazen [32] demonstrated a relationship between modifications in the acid-labile β-1,2-oligomannoside chain of a cell wall N-glycosylated protein and the cell surface hydrophicity status in Can- dida albicans, indicating the effect of fine structural dif- ferences in the carbohydrate part of a glycoprotein on its hydrophobic/hydrophilic properties. At last, a bioemulsi- fier liposan produced by Candida lipolytica was charac- terized as a glycoprotein with its carbohydrate moiety presenting a similar composition to that of Fusarium solani [33]. Fungal heteromannans are well known as glycosidic moieties of fungal wall glycopeptides and glycoproteins [28]. As shown by immunofluorescence for Fusarium javanicum, they are located in the surface of fungal walls [29]. Most studies on these polysaccharides were con- ducted for searching taxonomical and phylogenetical markers; and the classical extraction process by alkali treatments hydrolyses the linkage between the protein and glycosidic moieties. Our findings showing the capacity of an extracellular glycoprotein from an important soil fungus F. solani to solubilize a model PAH BaP point out another important role that can be played by these molecules: their potential involvement in microbial bio- avaibility of PAH, an important step in PAH biodegrada- tion. 5. Conclusion To summarize, our results show that the concentrated fil- trate produced by Fusarium solani stimulated BaP solu- bilization. The glycoprotein present in this filtrate should probably be involved in this enhancement. The chemical structure of its main glycosidic chain was established. Further research should be conducted in order to precise the role of protein and carbohydrate parts of the glyco- protein in the solubilization and uptake of BaP by this strain of F. solani. Our findings point out the potential role of fungal glycoproteins in microbial degradation of PAH. REFERENCES [1] A. Dipple, “Polynuclear Aromatic Carcinogens,” In: C. E. Searle, Ed., Chemical Carcinogens, ACS Monograph Series 3, American Chemical Society, Washington DC, 1976, pp. 245-314. [2] C. E. Cerniglia, “Biodegradation of Polycyclic Aromatic Hydrocarbons,” Biodegradation, Vol. 3, No. 2-3, 1992, pp. 351-368. doi:10.1007/BF00129093 [3] C. Rafin, O. Potin, E. Veignie, A. Lounes-Hadj Sahraoui and M. Sancholle, “Degradation of Benzo[a]Pyrene as Sole Carbon Source by a Non White Rot Fungus, Fusa- rium solani,” Polycyclic Aromatic Compounds, Vol. 21, No. 1-4, 2000, pp. 311-329. doi:10.1080/10406630008028542 [4] O. Potin, C. Rafin and E. Veignie, “Bioremediation of an Aged Polycyclic Aromatic Hydrocarbons (PAHs)-Con- taminated Soil by Filamentous Fungi Isolated from the Soil,” International Biodeterioration & Biodegradation, Vol. 54, No. 1, 2004, pp. 45-52. doi:10.1016/j.ibiod.2004.01.003 [5] O. Potin, E. Veignie and C. Rafin, “Biodegradation of Polycyclic Aromatic Hydrocarbons (PAHs) by Cladospo- rium sphaerospermum Isolated from an Aged PAH Con- taminated Soil,” FEMS Microbiology Ecology, Vol. 51, No. 1, 2004, pp. 71-78. doi:10.1016/j.femsec.2004.07.013 [6] Y. R. Wu, T. T. He, J. S. Lun, K. Maskaoui, T. W. Huang and Z. Hu, “Removal of Benzo[a]Pyrene by a Fungus Aspergillus sp. BAP14,” World Journal of Microbiology and Biotechnology, Vol. 25, No. 8, 2009, pp. 1395-1401. doi:10.1007/s11274-009-0026-2 [7] E. Veignie, C. Rafin, P. Woisel, A. L.-H. Sahraoui and F. Cazier, “Metabolization of the Polycyclic Aromatic Hy- drocarbon Benzo(a)Pyrene by a Non-White Rot Fungus (Fusarium solani) in a Batch Reactor,” Polycyclic Aro- matic Compounds, Vol. 22, No. 1, 2002, pp. 87-97. doi:10.1080/10406630210372 [8] C. Rafin, E. Veignie, P. Woisel, F. Cazier and G. Sur- pateanu, “New Potential of a Deuteromycete Fungus Fu- sarium solani in Benzo[a]Pyrene Degradation: An Eco- Physiological Hypothesis?” In: M. P. Glazer, Ed., New Frontiers in Environmental Research, Nova Science Pub- lishers, Inc., New York, 2006, pp. 165-179. [9] J. J. Cooney, C. Siporin and R. A. Smucker, “Physiologi- cal and Cytological Responses to Hydrocarbons by the Hydrocarbon-Using Fungus Cladosporium resinae,” Bo- tanica Marina, Vol. 23, 1980, pp. 227-232. [10] O. Kappeli, P. Walther, M. Mueller and A. Fiechter, “Structure of the Cell Surface of the Yeast Candida tro- picalis and Its Relation to Hydrocarbon Transport,” Ar- chives of Microbiology, Vol. 138, No. 4, 1984, pp. 279- 282. doi:10.1007/BF00410890 [11] Y. Deng, Y. Zhang, A. E. L. Hesham, R. Liu and M. Yang, “Cell Surface Properties of Five Polycyclic Aro- matic Compound Degrading Yeast Strains,” Applied Mi- crobiology and Biotechnology, Vol. 86, No. 6, 2010, pp. 1933-1939. doi:10.1007/s00253-010-2477-7 [12] N. D. Lindley and M. T. Heydeman, “Uptake of Vapour Phase [14C]Dodecane by Whole Mycelia of Cladospo- rium resinae,” Journal of General Microbiology, Vol. 129, No. 7, 1983, pp. 2301-2305. [13] I. Ciucanu and F. Kerek, “A Simple and Rapid Method Copyright © 2012 SciRes. AiM
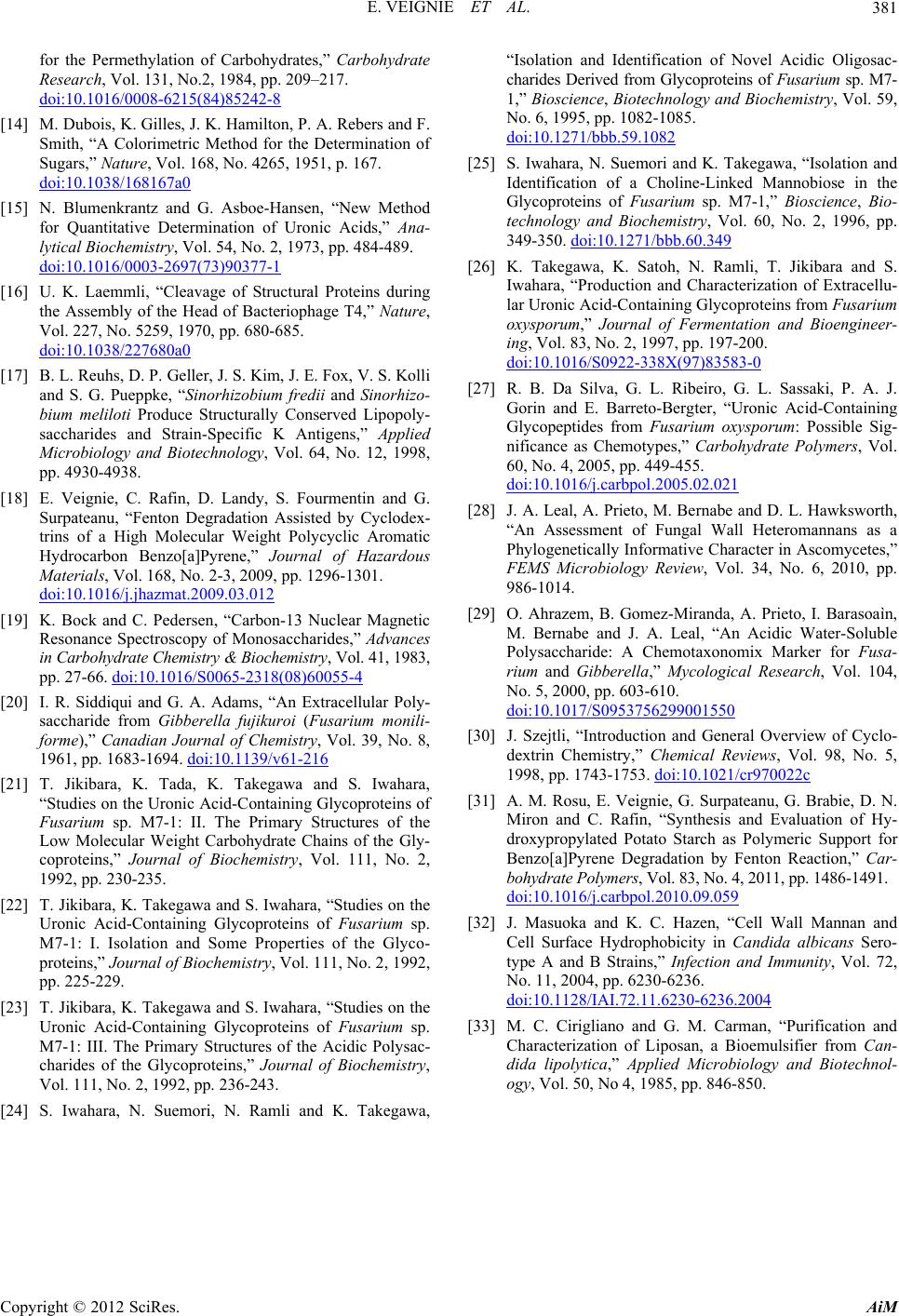 E. VEIGNIE ET AL. Copyright © 2012 SciRes. AiM 381 for the Permethylation of Carbohydrates,” Carbohydrate Research, Vol. 131, No.2, 1984, pp. 209–217. doi:10.1016/0008-6215(84)85242-8 [14] M. Dubois, K. Gilles, J. K. Hamilton, P. A. Rebers and F. Smith, “A Colorimetric Method for the Determination of Sugars,” Nature, Vol. 168, No. 4265, 1951, p. 167. doi:10.1038/168167a0 [15] N. Blumenkrantz and G. Asboe-Hansen, “New Method for Quantitative Determination of Uronic Acids,” Ana- lytical Biochemistry, Vol. 54, No. 2, 1973, pp. 484-489. doi:10.1016/0003-2697(73)90377-1 [16] U. K. Laemmli, “Cleavage of Structural Proteins during the Assembly of the Head of Bacteriophage T4,” Nature, Vol. 227, No. 5259, 1970, pp. 680-685. doi:10.1038/227680a0 [17] B. L. Reuhs, D. P. Geller, J. S. Kim, J. E. Fox, V. S. Kolli and S. G. Pueppke, “Sinorhizobium fredii and Sinorhizo- bium meliloti Produce Structurally Conserved Lipopoly- saccharides and Strain-Specific K Antigens,” Applied Microbiology and Biotechnology, Vol. 64, No. 12, 1998, pp. 4930-4938. [18] E. Veignie, C. Rafin, D. Landy, S. Fourmentin and G. Surpateanu, “Fenton Degradation Assisted by Cyclodex- trins of a High Molecular Weight Polycyclic Aromatic Hydrocarbon Benzo[a]Pyrene,” Journal of Hazardous Materials, Vol. 168, No. 2-3, 2009, pp. 1296-1301. doi:10.1016/j.jhazmat.2009.03.012 [19] K. Bock and C. Pedersen, “Carbon-13 Nuclear Magnetic Resonance Spectroscopy of Monosaccharides,” Advances in Carbohydrate Chemistry & Biochemistry, Vol. 41, 1983, pp. 27-66. doi:10.1016/S0065-2318(08)60055-4 [20] I. R. Siddiqui and G. A. Adams, “An Extracellular Poly- saccharide from Gibberella fujikuroi (Fusarium monili- forme),” Canadian Journal of Chemistry, Vol. 39, No. 8, 1961, pp. 1683-1694. doi:10.1139/v61-216 [21] T. Jikibara, K. Tada, K. Takegawa and S. Iwahara, “Studies on the Uronic Acid-Containing Glycoproteins of Fusarium sp. M7-1: II. The Primary Structures of the Low Molecular Weight Carbohydrate Chains of the Gly- coproteins,” Journal of Biochemistry, Vol. 111, No. 2, 1992, pp. 230-235. [22] T. Jikibara, K. Takegawa and S. Iwahara, “Studies on the Uronic Acid-Containing Glycoproteins of Fusarium sp. M7-1: I. Isolation and Some Properties of the Glyco- proteins,” Journal of Biochemistry, Vol. 111, No. 2, 1992, pp. 225-229. [23] T. Jikibara, K. Takegawa and S. Iwahara, “Studies on the Uronic Acid-Containing Glycoproteins of Fusarium sp. M7-1: III. The Primary Structures of the Acidic Polysac- charides of the Glycoproteins,” Journal of Biochemistry, Vol. 111, No. 2, 1992, pp. 236-243. [24] S. Iwahara, N. Suemori, N. Ramli and K. Takegawa, “Isolation and Identification of Novel Acidic Oligosac- charides Derived from Glycoproteins of Fusarium sp. M7- 1,” Bioscience, Biotechnology and Biochemistry, Vol. 59, No. 6, 1995, pp. 1082-1085. doi:10.1271/bbb.59.1082 [25] S. Iwahara, N. Suemori and K. Takegawa, “Isolation and Identification of a Choline-Linked Mannobiose in the Glycoproteins of Fusarium sp. M7-1,” Bioscience, Bio- technology and Biochemistry, Vol. 60, No. 2, 1996, pp. 349-350. doi:10.1271/bbb.60.349 [26] K. Takegawa, K. Satoh, N. Ramli, T. Jikibara and S. Iwahara, “Production and Characterization of Extracellu- lar Uronic Acid-Containing Glycoproteins from Fusarium oxysporum,” Journal of Fermentation and Bioengineer- ing, Vol. 83, No. 2, 1997, pp. 197-200. doi:10.1016/S0922-338X(97)83583-0 [27] R. B. Da Silva, G. L. Ribeiro, G. L. Sassaki, P. A. J. Gorin and E. Barreto-Bergter, “Uronic Acid-Containing Glycopeptides from Fusarium oxysporum: Possible Sig- nificance as Chemotypes,” Carbohydrate Polymers, Vol. 60, No. 4, 2005, pp. 449-455. doi:10.1016/j.carbpol.2005.02.021 [28] J. A. Leal, A. Prieto, M. Bernabe and D. L. Hawksworth, “An Assessment of Fungal Wall Heteromannans as a Phylogenetically Informative Character in Ascomycetes,” FEMS Microbiology Review, Vol. 34, No. 6, 2010, pp. 986-1014. [29] O. Ahrazem, B. Gomez-Miranda, A. Prieto, I. Barasoaìn, M. Bernabe and J. A. Leal, “An Acidic Water-Soluble Polysaccharide: A Chemotaxonomix Marker for Fusa- rium and Gibberella,” Mycological Research, Vol. 104, No. 5, 2000, pp. 603-610. doi:10.1017/S0953756299001550 [30] J. Szejtli, “Introduction and General Overview of Cyclo- dextrin Chemistry,” Chemical Reviews, Vol. 98, No. 5, 1998, pp. 1743-1753. doi:10.1021/cr970022c [31] A. M. Rosu, E. Veignie, G. Surpateanu, G. Brabie, D. N. Miron and C. Rafin, “Synthesis and Evaluation of Hy- droxypropylated Potato Starch as Polymeric Support for Benzo[a]Pyrene Degradation by Fenton Reaction,” Car- bohydrate Polymers, Vol. 83, No. 4, 2011, pp. 1486-1491. doi:10.1016/j.carbpol.2010.09.059 [32] J. Masuoka and K. C. Hazen, “Cell Wall Mannan and Cell Surface Hydrophobicity in Candida albicans Sero- type A and B Strains,” Infection and Immunity, Vol. 72, No. 11, 2004, pp. 6230-6236. doi:10.1128/IAI.72.11.6230-6236.2004 [33] M. C. Cirigliano and G. M. Carman, “Purification and Characterization of Liposan, a Bioemulsifier from Can- dida lipolytica,” Applied Microbiology and Biotechnol- ogy, Vol. 50, No 4, 1985, pp. 846-850.
|