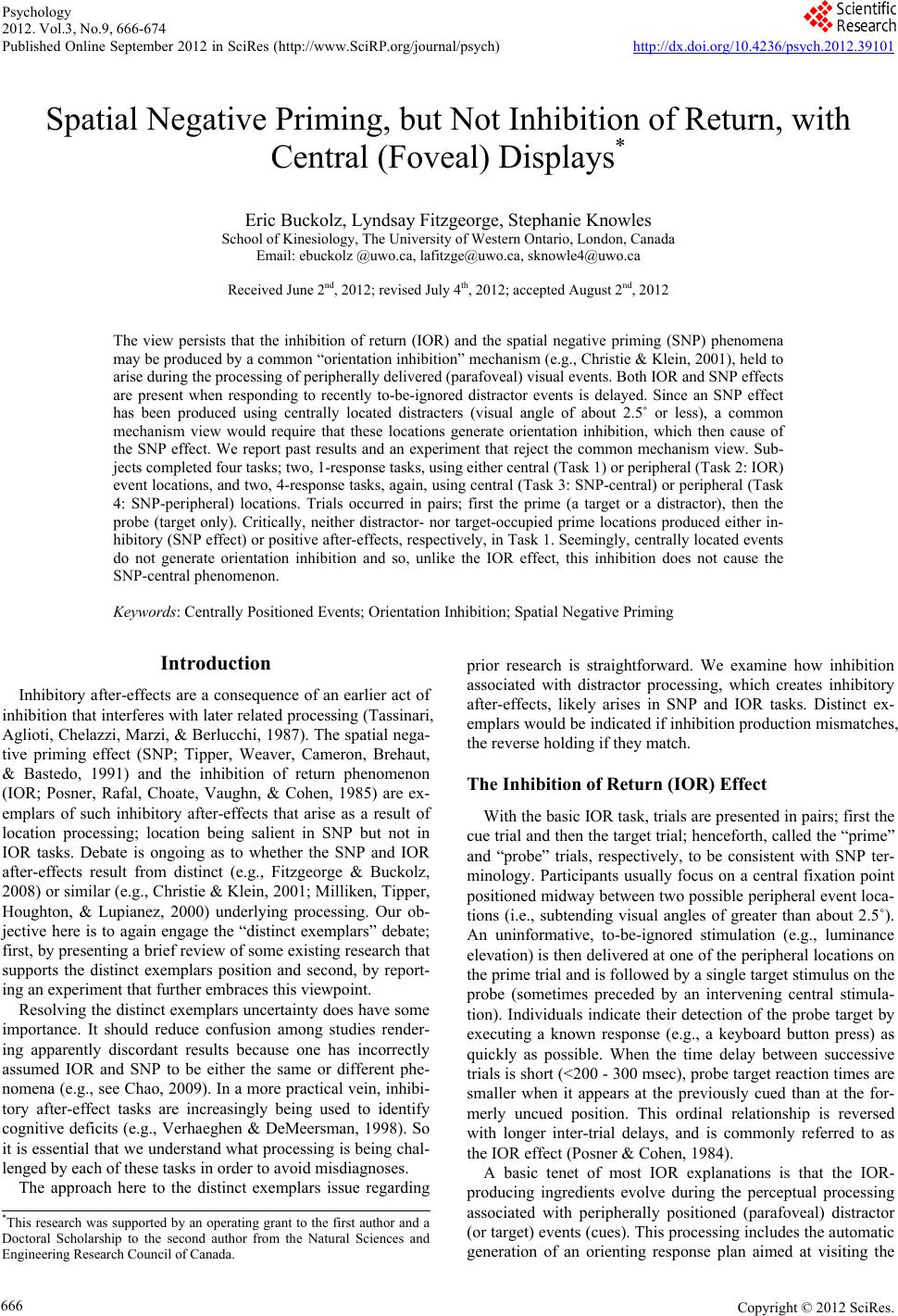 Psychology 2012. Vol.3, No.9, 666-674 Published Online September 2012 in SciRes (http://www.SciRP.org/journal/psych) http://dx.doi.org/10.4236/psych.2012.39101 Copyright © 2012 SciRes. 666 Spatial Negative Priming, but Not Inhibition of Return, with Central (Foveal) Displays* Eric Buckolz, Lyndsay Fitzgeorge, Stephanie Knowles School of Kinesiology, The University of Western Ontario, London, Canada Email: ebuckolz @uwo.ca, lafitzge@uwo.ca, sknowle4@uwo.ca Received June 2nd, 2012; revised July 4th, 2012; accepted August 2nd, 2012 The view persists that the inhibition of return (IOR) and the spatial negative priming (SNP) phenomena may be produced by a common “orientation inhibition” mechanism (e.g., Christie & Klein, 2001), held to arise during the processing of peripherally delivered (parafoveal) visual events. Both IOR and SNP effects are present when responding to recently to-be-ignored distractor events is delayed. Since an SNP effect has been produced using centrally located distracters (visual angle of about 2.5˚ or less), a common mechanism view would require that these locations generate orientation inhibition, which then cause of the SNP effect. We report past results and an experiment that reject the common mechanism view. Sub- jects completed four tasks; two, 1-response tasks, using either central (Task 1) or peripheral (Task 2: IOR) event locations, and two, 4-response tasks, again, using central (Task 3: SNP-central) or peripheral (Task 4: SNP-peripheral) locations. Trials occurred in pairs; first the prime (a target or a distractor), then the probe (target only). Critically, neither distractor- nor target-occupied prime locations produced either in- hibitory (SNP effect) or positive after-effects, respectively, in Task 1. Seemingly, centrally located events do not generate orientation inhibition and so, unlike the IOR effect, this inhibition does not cause the SNP-central phenomenon. Keywords: Centrally Positioned Events; Orientation Inhibition; Spatial Negative Priming Introduction Inhibitory after-effects are a consequence of an earlier act of inhibition that interferes with later related processing (Tassinari, Aglioti, Chelazzi, Marzi, & Berlucchi, 1987). The spatial nega- tive priming effect (SNP; Tipper, Weaver, Cameron, Brehaut, & Bastedo, 1991) and the inhibition of return phenomenon (IOR; Posner, Rafal, Choate, Vaughn, & Cohen, 1985) are ex- emplars of such inhibitory after-effects that arise as a result of location processing; location being salient in SNP but not in IOR tasks. Debate is ongoing as to whether the SNP and IOR after-effects result from distinct (e.g., Fitzgeorge & Buckolz, 2008) or similar (e.g., Christie & Klein, 2001; Milliken, Tipper, Houghton, & Lupianez, 2000) underlying processing. Our ob- jective here is to again engage the “distinct exemplars” debate; first, by presenting a brief review of some existing research that supports the distinct exemplars position and second, by report- ing an experiment that further embraces this viewpoint. Resolving the distinct exemplars uncertainty does have some importance. It should reduce confusion among studies render- ing apparently discordant results because one has incorrectly assumed IOR and SNP to be either the same or different phe- nomena (e.g., see Chao, 2009). In a more practical vein, inhibi- tory after-effect tasks are increasingly being used to identify cognitive deficits (e.g., Verhaeghen & DeMeersman, 1998). So it is essential that we understand what processing is being chal- lenged by each of these tasks in order to avoid misdiagnoses. The approach here to the distinct exemplars issue regarding prior research is straightforward. We examine how inhibition associated with distractor processing, which creates inhibitory after-effects, likely arises in SNP and IOR tasks. Distinct ex- emplars would be indicated if inhibition production mismatches, the reverse holding if they match. The Inhibition of Return (IOR) Effect With the basic IOR task, trials are presented in pairs; first the cue trial and then the target trial; henceforth, called the “prime” and “probe” trials, respectively, to be consistent with SNP ter- minology. Participants usually focus on a central fixation point positioned midway between two possible peripheral event loca- tions (i.e., subtending visual angles of greater than about 2.5˚). An uninformative, to-be-ignored stimulation (e.g., luminance elevation) is then delivered at one of the peripheral locations on the prime trial and is followed by a single target stimulus on the probe (sometimes preceded by an intervening central stimula- tion). Individuals indicate their detection of the probe target by executing a known response (e.g., a keyboard button press) as quickly as possible. When the time delay between successive trials is short (<200 - 300 msec), probe target reaction times are smaller when it appears at the previously cued than at the for- merly uncued position. This ordinal relationship is reversed with longer inter-trial delays, and is commonly referred to as the IOR effect (Posner & Cohen, 1984). A basic tenet of most IOR explanations is that the IOR- producing ingredients evolve during the perceptual processing associated with peripherally positioned (parafoveal) distractor (or target) events (cues). This processing includes the automatic generation of an orienting response plan aimed at visiting the *This research was supported by an operating grant to the first author and a Doctoral Scholarship to the second author from the Natural Sciences and En ineerin Research Council of Canada.
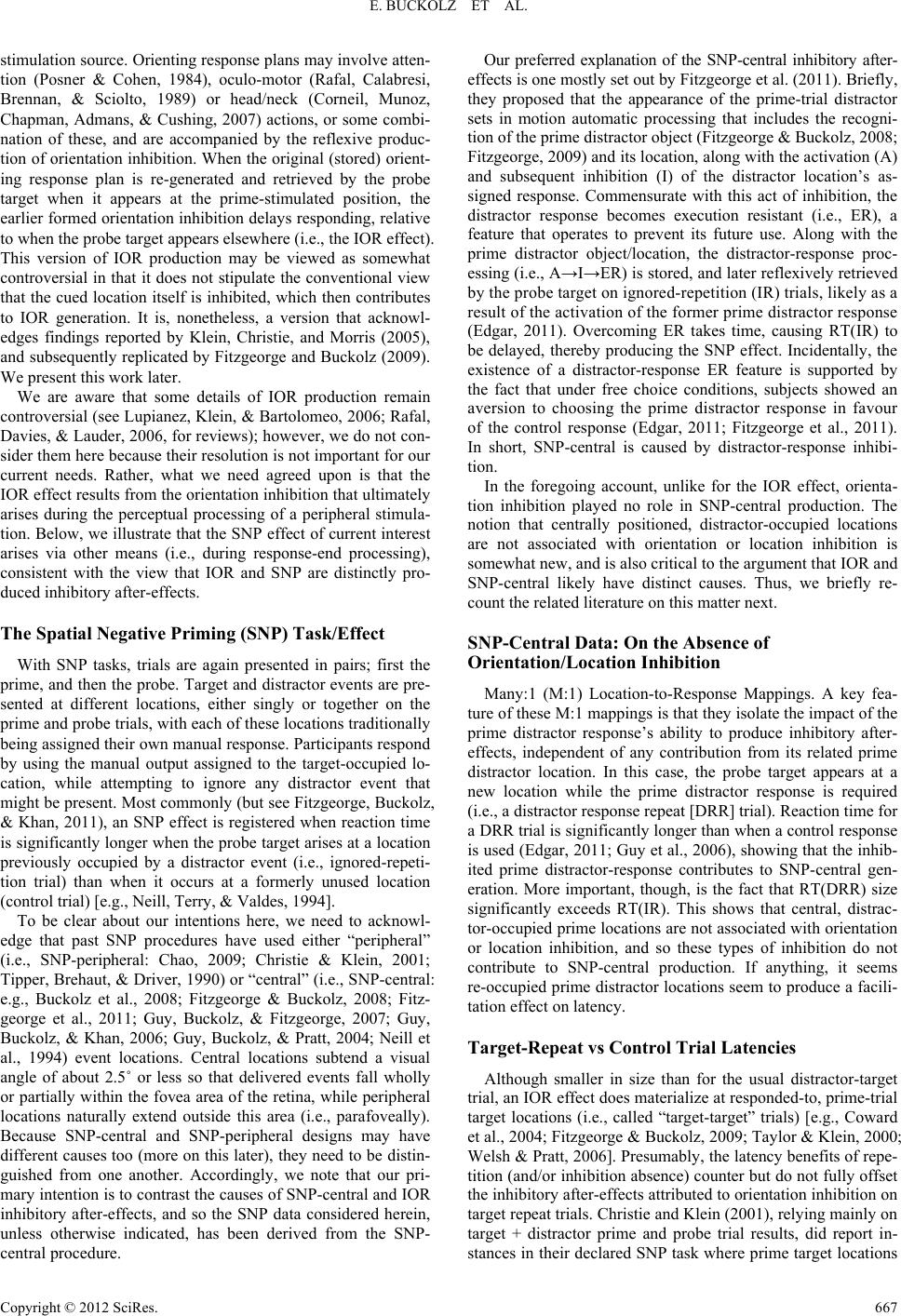 E. BUCKOLZ ET AL. stimulation source. Orienting response plans may involve atten- tion (Posner & Cohen, 1984), oculo-motor (Rafal, Calabresi, Brennan, & Sciolto, 1989) or head/neck (Corneil, Munoz, Chapman, Admans, & Cushing, 2007) actions, or some combi- nation of these, and are accompanied by the reflexive produc- tion of orientation inhibition. When the original (stored) orient- ing response plan is re-generated and retrieved by the probe target when it appears at the prime-stimulated position, the earlier formed orientation inhibition delays responding, relative to when the probe target appears elsewhere (i.e., the IOR effect). This version of IOR production may be viewed as somewhat controversial in that it does not stipulate the conventional view that the cued location itself is inhibited, which then contributes to IOR generation. It is, nonetheless, a version that acknowl- edges findings reported by Klein, Christie, and Morris (2005), and subsequently replicated by Fitzgeorge and Buckolz (2009). We present this work later. We are aware that some details of IOR production remain controversial (see Lupianez, Klein, & Bartolomeo, 2006; Rafal, Davies, & Lauder, 2006, for reviews); however, we do not con- sider them here because their resolution is not important for our current needs. Rather, what we need agreed upon is that the IOR effect results from the orientation inhibition that ultimately arises during the perceptual processing of a peripheral stimula- tion. Below, we illustrate that the SNP effect of current interest arises via other means (i.e., during response-end processing), consistent with the view that IOR and SNP are distinctly pro- duced inhibitory after-effects. The Spatial Negative Priming (SNP) Task/Effect With SNP tasks, trials are again presented in pairs; first the prime, and then the probe. Target and distractor events are pre- sented at different locations, either singly or together on the prime and probe trials, with each of these locations traditionally being assigned their own manual response. Participants respond by using the manual output assigned to the target-occupied lo- cation, while attempting to ignore any distractor event that might be present. Most commonly (but see Fitzgeorge, Buckolz, & Khan, 2011), an SNP effect is registered when reaction time is significantly longer when the probe target arises at a location previously occupied by a distractor event (i.e., ignored-repeti- tion trial) than when it occurs at a formerly unused location (control trial) [e.g., Neill, Terry, & Valdes, 1994]. To be clear about our intentions here, we need to acknowl- edge that past SNP procedures have used either “peripheral” (i.e., SNP-peripheral: Chao, 2009; Christie & Klein, 2001; Tipper, Brehaut, & Driver, 1990) or “central” (i.e., SNP-central: e.g., Buckolz et al., 2008; Fitzgeorge & Buckolz, 2008; Fitz- george et al., 2011; Guy, Buckolz, & Fitzgeorge, 2007; Guy, Buckolz, & Khan, 2006; Guy, Buckolz, & Pratt, 2004; Neill et al., 1994) event locations. Central locations subtend a visual angle of about 2.5˚ or less so that delivered events fall wholly or partially within the fovea area of the retina, while peripheral locations naturally extend outside this area (i.e., parafoveally). Because SNP-central and SNP-peripheral designs may have different causes too (more on this later), they need to be distin- guished from one another. Accordingly, we note that our pri- mary intention is to contrast the causes of SNP-central and IOR inhibitory after-effects, and so the SNP data considered herein, unless otherwise indicated, has been derived from the SNP- central procedure. Our preferred explanation of the SNP-central inhibitory after- effects is one mostly set out by Fitzgeorge et al. (2011). Briefly, they proposed that the appearance of the prime-trial distractor sets in motion automatic processing that includes the recogni- tion of the prime distractor object (Fitzgeorge & Buckolz, 2008; Fitzgeorge, 2009) and its location, along with the activation (A) and subsequent inhibition (I) of the distractor location’s as- signed response. Commensurate with this act of inhibition, the distractor response becomes execution resistant (i.e., ER), a feature that operates to prevent its future use. Along with the prime distractor object/location, the distractor-response proc- essing (i.e., A→I→ER) is stored, and later reflexively retrieved by the probe target on ignored-repetition (IR) trials, likely as a result of the activation of the former prime distractor response (Edgar, 2011). Overcoming ER takes time, causing RT(IR) to be delayed, thereby producing the SNP effect. Incidentally, the existence of a distractor-response ER feature is supported by the fact that under free choice conditions, subjects showed an aversion to choosing the prime distractor response in favour of the control response (Edgar, 2011; Fitzgeorge et al., 2011). In short, SNP-central is caused by distractor-response inhibi- tion. In the foregoing account, unlike for the IOR effect, orienta- tion inhibition played no role in SNP-central production. The notion that centrally positioned, distractor-occupied locations are not associated with orientation or location inhibition is somewhat new, and is also critical to the argument that IOR and SNP-central likely have distinct causes. Thus, we briefly re- count the related literature on this matter next. SNP-Central Data: On th e Absence of Orientation/Location Inhibition Many:1 (M:1) Location-to-Response Mappings. A key fea- ture of these M:1 mappings is that they isolate the impact of the prime distractor response’s ability to produce inhibitory after- effects, independent of any contribution from its related prime distractor location. In this case, the probe target appears at a new location while the prime distractor response is required (i.e., a distractor response repeat [DRR] trial). Reaction time for a DRR trial is significantly longer than when a control response is used (Edgar, 2011; Guy et al., 2006), showing that the inhib- ited prime distractor-response contributes to SNP-central gen- eration. More important, though, is the fact that RT(DRR) size significantly exceeds RT(IR). This shows that central, distrac- tor-occupied prime locations are not associated with orientation or location inhibition, and so these types of inhibition do not contribute to SNP-central production. If anything, it seems re-occupied prime distractor locations seem to produce a facili- tation effect on latency. Target-Repeat vs Control Trial Latencies Although smaller in size than for the usual distractor-target trial, an IOR effect does materialize at responded-to, prime-trial target locations (i.e., called “target-target” trials) [e.g., Coward et al., 2004; Fitzgeorge & Buckolz, 2009; Taylor & Klein, 2000; Welsh & Pratt, 2006]. Presumably, the latency benefits of repe- tition (and/or inhibition absence) counter but do not fully offset the inhibitory after-effects attributed to orientation inhibition on target repeat trials. Christie and Klein (2001), relying mainly on target + distractor prime and probe trial results, did report in- stances in their declared SNP task where prime target locations Copyright © 2012 SciRes. 667
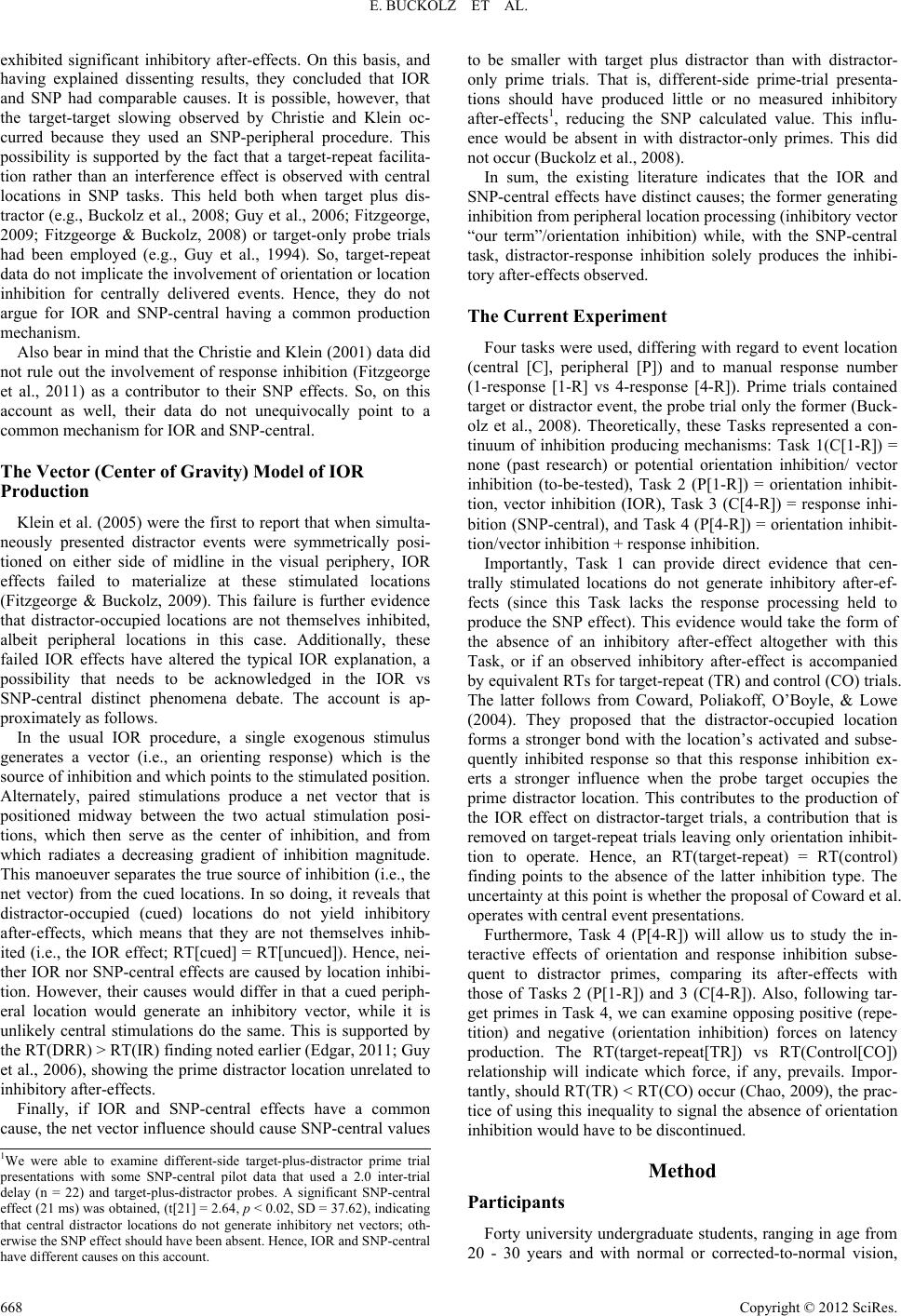 E. BUCKOLZ ET AL. exhibited significant inhibitory after-effects. On this basis, and having explained dissenting results, they concluded that IOR and SNP had comparable causes. It is possible, however, that the target-target slowing observed by Christie and Klein oc- curred because they used an SNP-peripheral procedure. This possibility is supported by the fact that a target-repeat facilita- tion rather than an interference effect is observed with central locations in SNP tasks. This held both when target plus dis- tractor (e.g., Buckolz et al., 2008; Guy et al., 2006; Fitzgeorge, 2009; Fitzgeorge & Buckolz, 2008) or target-only probe trials had been employed (e.g., Guy et al., 1994). So, target-repeat data do not implicate the involvement of orientation or location inhibition for centrally delivered events. Hence, they do not argue for IOR and SNP-central having a common production mechanism. Also bear in mind that the Christie and Klein (2001) data did not rule out the involvement of response inhibition (Fitzgeorge et al., 2011) as a contributor to their SNP effects. So, on this account as well, their data do not unequivocally point to a common mechanism for IOR and SNP-central. The Vector (Center of Gravity) Model of IOR Production Klein et al. (2005) were the first to report that when simulta- neously presented distractor events were symmetrically posi- tioned on either side of midline in the visual periphery, IOR effects failed to materialize at these stimulated locations (Fitzgeorge & Buckolz, 2009). This failure is further evidence that distractor-occupied locations are not themselves inhibited, albeit peripheral locations in this case. Additionally, these failed IOR effects have altered the typical IOR explanation, a possibility that needs to be acknowledged in the IOR vs SNP-central distinct phenomena debate. The account is ap- proximately as follows. In the usual IOR procedure, a single exogenous stimulus generates a vector (i.e., an orienting response) which is the source of inhibition and which points to the stimulated position. Alternately, paired stimulations produce a net vector that is positioned midway between the two actual stimulation posi- tions, which then serve as the center of inhibition, and from which radiates a decreasing gradient of inhibition magnitude. This manoeuver separates the true source of inhibition (i.e., the net vector) from the cued locations. In so doing, it reveals that distractor-occupied (cued) locations do not yield inhibitory after-effects, which means that they are not themselves inhib- ited (i.e., the IOR effect; RT[cued] = RT[uncued]). Hence, nei- ther IOR nor SNP-central effects are caused by location inhibi- tion. However, their causes would differ in that a cued periph- eral location would generate an inhibitory vector, while it is unlikely central stimulations do the same. This is supported by the RT(DRR) > RT(IR) finding noted earlier (Edgar, 2011; Guy et al., 2006), showing the prime distractor location unrelated to inhibitory after-effects. Finally, if IOR and SNP-central effects have a common cause, the net vector influence should cause SNP-central values to be smaller with target plus distractor than with distractor- only prime trials. That is, different-side prime-trial presenta- tions should have produced little or no measured inhibitory after-effects1, reducing the SNP calculated value. This influ- ence would be absent in with distractor-only primes. This did not occur (Buckolz et al., 2008). In sum, the existing literature indicates that the IOR and SNP-central effects have distinct causes; the former generating inhibition from peripheral location processing (inhibitory vector “our term”/orientation inhibition) while, with the SNP-central task, distractor-response inhibition solely produces the inhibi- tory after-effects observed. The Current Experiment Four tasks were used, differing with regard to event location (central [C], peripheral [P]) and to manual response number (1-response [1-R] vs 4-response [4-R]). Prime trials contained target or distractor event, the probe trial only the former (Buck- olz et al., 2008). Theoretically, these Tasks represented a con- tinuum of inhibition producing mechanisms: Task 1(C[1-R]) = none (past research) or potential orientation inhibition/ vector inhibition (to-be-tested), Task 2 (P[1-R]) = orientation inhibit- tion, vector inhibition (IOR), Task 3 (C[4-R]) = response inhi- bition (SNP-central), and Task 4 (P[4-R]) = orientation inhibit- tion/vector inhibition + response inhibition. Importantly, Task 1 can provide direct evidence that cen- trally stimulated locations do not generate inhibitory after-ef- fects (since this Task lacks the response processing held to produce the SNP effect). This evidence would take the form of the absence of an inhibitory after-effect altogether with this Task, or if an observed inhibitory after-effect is accompanied by equivalent RTs for target-repeat (TR) and control (CO) trials. The latter follows from Coward, Poliakoff, O’Boyle, & Lowe (2004). They proposed that the distractor-occupied location forms a stronger bond with the location’s activated and subse- quently inhibited response so that this response inhibition ex- erts a stronger influence when the probe target occupies the prime distractor location. This contributes to the production of the IOR effect on distractor-target trials, a contribution that is removed on target-repeat trials leaving only orientation inhibit- tion to operate. Hence, an RT(target-repeat) = RT(control) finding points to the absence of the latter inhibition type. The uncertainty at this point is whether the proposal of Coward et al. operates with central event presentations. Furthermore, Task 4 (P[4-R]) will allow us to study the in- teractive effects of orientation and response inhibition subse- quent to distractor primes, comparing its after-effects with those of Tasks 2 (P[1-R]) and 3 (C[4-R]). Also, following tar- get primes in Task 4, we can examine opposing positive (repe- tition) and negative (orientation inhibition) forces on latency production. The RT(target-repeat[TR]) vs RT(Control[CO]) relationship will indicate which force, if any, prevails. Impor- tantly, should RT(TR) < RT(CO) occur (Chao, 2009), the prac- tice of using this inequality to signal the absence of orientation inhibition would have to be discontinued. 1We were able to examine different-side target-plus-distractor prime trial presentations with some SNP-central pilot data that used a 2.0 inter-trial delay (n = 22) and target-plus-distractor probes. A significant SNP-central effect (21 ms) was obtained, (t[21] = 2.64, p < 0.02, SD = 37.62), indicating that central distractor locations do not generate inhibitory net vectors; oth- erwise the SNP effect should have been absent. Hence, IOR and SNP-central have different causes on this account. Method Participants Forty university undergraduate students, ranging in age from 20 - 30 years and with normal or corrected-to-normal vision, Copyright © 2012 SciRes. 668
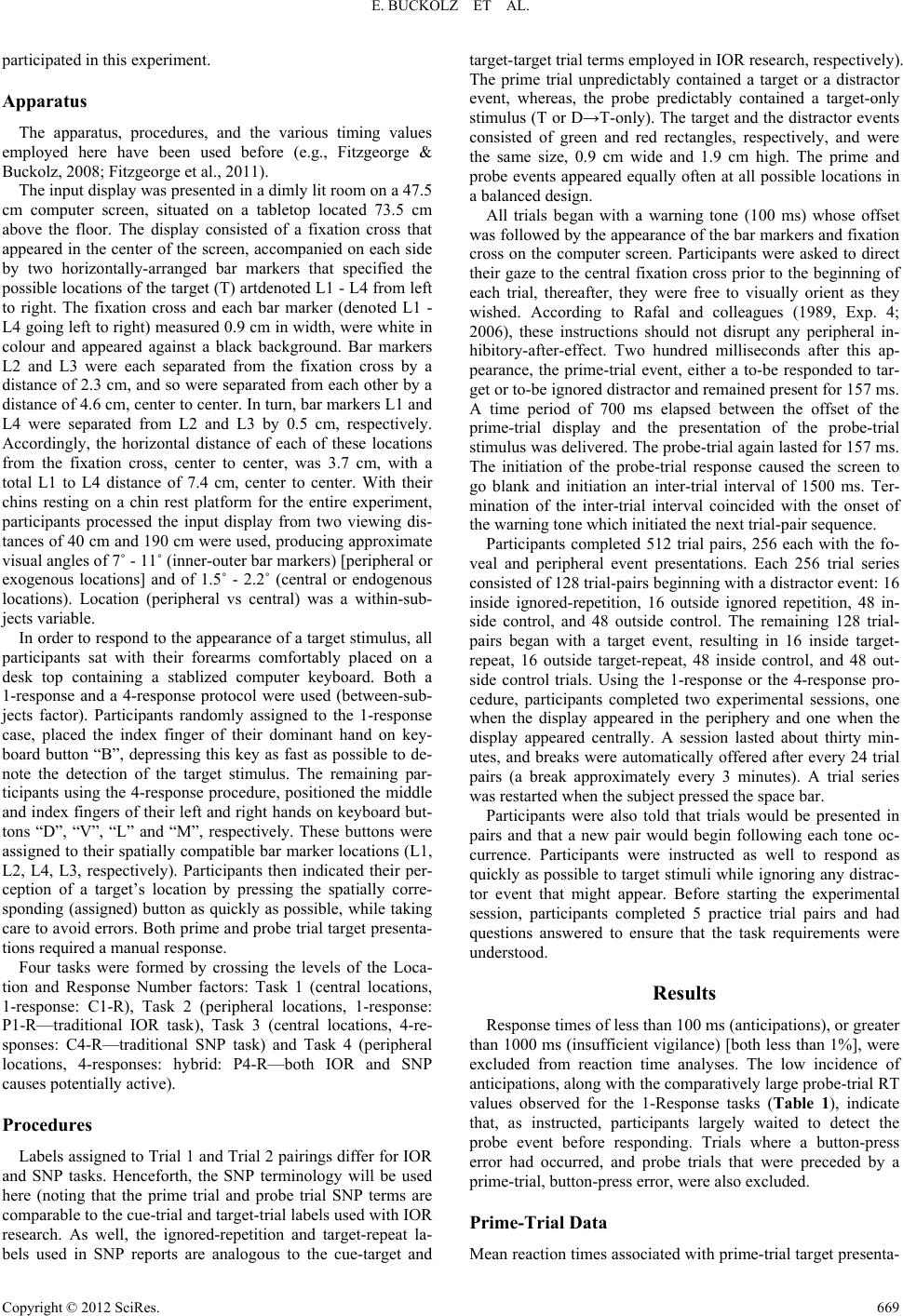 E. BUCKOLZ ET AL. participated in this experiment. Apparatus The apparatus, procedures, and the various timing values employed here have been used before (e.g., Fitzgeorge & Buckolz, 2008; Fitzgeorge et al., 2011). The input display was presented in a dimly lit room on a 47.5 cm computer screen, situated on a tabletop located 73.5 cm above the floor. The display consisted of a fixation cross that appeared in the center of the screen, accompanied on each side by two horizontally-arranged bar markers that specified the possible locations of the target (T) artdenoted L1 - L4 from left to right. The fixation cross and each bar marker (denoted L1 - L4 going left to right) measured 0.9 cm in width, were white in colour and appeared against a black background. Bar markers L2 and L3 were each separated from the fixation cross by a distance of 2.3 cm, and so were separated from each other by a distance of 4.6 cm, center to center. In turn, bar markers L1 and L4 were separated from L2 and L3 by 0.5 cm, respectively. Accordingly, the horizontal distance of each of these locations from the fixation cross, center to center, was 3.7 cm, with a total L1 to L4 distance of 7.4 cm, center to center. With their chins resting on a chin rest platform for the entire experiment, participants processed the input display from two viewing dis- tances of 40 cm and 190 cm were used, producing approximate visual angles of 7˚ - 11˚ (inner-outer bar markers) [peripheral or exogenous locations] and of 1.5˚ - 2.2˚ (central or endogenous locations). Location (peripheral vs central) was a within-sub- jects variable. In order to respond to the appearance of a target stimulus, all participants sat with their forearms comfortably placed on a desk top containing a stablized computer keyboard. Both a 1-response and a 4-response protocol were used (between-sub- jects factor). Participants randomly assigned to the 1-response case, placed the index finger of their dominant hand on key- board button “B”, depressing this key as fast as possible to de- note the detection of the target stimulus. The remaining par- ticipants using the 4-response procedure, positioned the middle and index fingers of their left and right hands on keyboard but- tons “D”, “V”, “L” and “M”, respectively. These buttons were assigned to their spatially compatible bar marker locations (L1, L2, L4, L3, respectively). Participants then indicated their per- ception of a target’s location by pressing the spatially corre- sponding (assigned) button as quickly as possible, while taking care to avoid errors. Both prime and probe trial target presenta- tions required a manual response. Four tasks were formed by crossing the levels of the Loca- tion and Response Number factors: Task 1 (central locations, 1-response: C1-R), Task 2 (peripheral locations, 1-response: P1-R—traditional IOR task), Task 3 (central locations, 4-re- sponses: C4-R—traditional SNP task) and Task 4 (peripheral locations, 4-responses: hybrid: P4-R—both IOR and SNP causes potentially active). Procedures Labels assigned to Trial 1 and Trial 2 pairings differ for IOR and SNP tasks. Henceforth, the SNP terminology will be used here (noting that the prime trial and probe trial SNP terms are comparable to the cue-trial and target-trial labels used with IOR research. As well, the ignored-repetition and target-repeat la- bels used in SNP reports are analogous to the cue-target and target-target trial terms employed in IOR research, respectively). The prime trial unpredictably contained a target or a distractor event, whereas, the probe predictably contained a target-only stimulus (T or D→T-only). The target and the distractor events consisted of green and red rectangles, respectively, and were the same size, 0.9 cm wide and 1.9 cm high. The prime and probe events appeared equally often at all possible locations in a balanced design. All trials began with a warning tone (100 ms) whose offset was followed by the appearance of the bar markers and fixation cross on the computer screen. Participants were asked to direct their gaze to the central fixation cross prior to the beginning of each trial, thereafter, they were free to visually orient as they wished. According to Rafal and colleagues (1989, Exp. 4; 2006), these instructions should not disrupt any peripheral in- hibitory-after-effect. Two hundred milliseconds after this ap- pearance, the prime-trial event, either a to-be responded to tar- get or to-be ignored distractor and remained present for 157 ms. A time period of 700 ms elapsed between the offset of the prime-trial display and the presentation of the probe-trial stimulus was delivered. The probe-trial again lasted for 157 ms. The initiation of the probe-trial response caused the screen to go blank and initiation an inter-trial interval of 1500 ms. Ter- mination of the inter-trial interval coincided with the onset of the warning tone which initiated the next trial-pair sequence. Participants completed 512 trial pairs, 256 each with the fo- veal and peripheral event presentations. Each 256 trial series consisted of 128 trial-pairs beginning with a distractor event: 16 inside ignored-repetition, 16 outside ignored repetition, 48 in- side control, and 48 outside control. The remaining 128 trial- pairs began with a target event, resulting in 16 inside target- repeat, 16 outside target-repeat, 48 inside control, and 48 out- side control trials. Using the 1-response or the 4-response pro- cedure, participants completed two experimental sessions, one when the display appeared in the periphery and one when the display appeared centrally. A session lasted about thirty min- utes, and breaks were automatically offered after every 24 trial pairs (a break approximately every 3 minutes). A trial series was restarted when the subject pressed the space bar. Participants were also told that trials would be presented in pairs and that a new pair would begin following each tone oc- currence. Participants were instructed as well to respond as quickly as possible to target stimuli while ignoring any distrac- tor event that might appear. Before starting the experimental session, participants completed 5 practice trial pairs and had questions answered to ensure that the task requirements were understood. Results Response times of less than 100 ms (anticipations), or greater than 1000 ms (insufficient vigilance) [both less than 1%], were excluded from reaction time analyses. The low incidence of anticipations, along with the comparatively large probe-trial RT values observed for the 1-Response tasks (Table 1), indicate that, as instructed, participants largely waited to detect the probe event before responding. Trials where a button-press error had occurred, and probe trials that were preceded by a prime-trial, button-press error, were also excluded. Prime-Trial Data Mean reaction times associated with prime-trial target presenta- Copyright © 2012 SciRes. 669
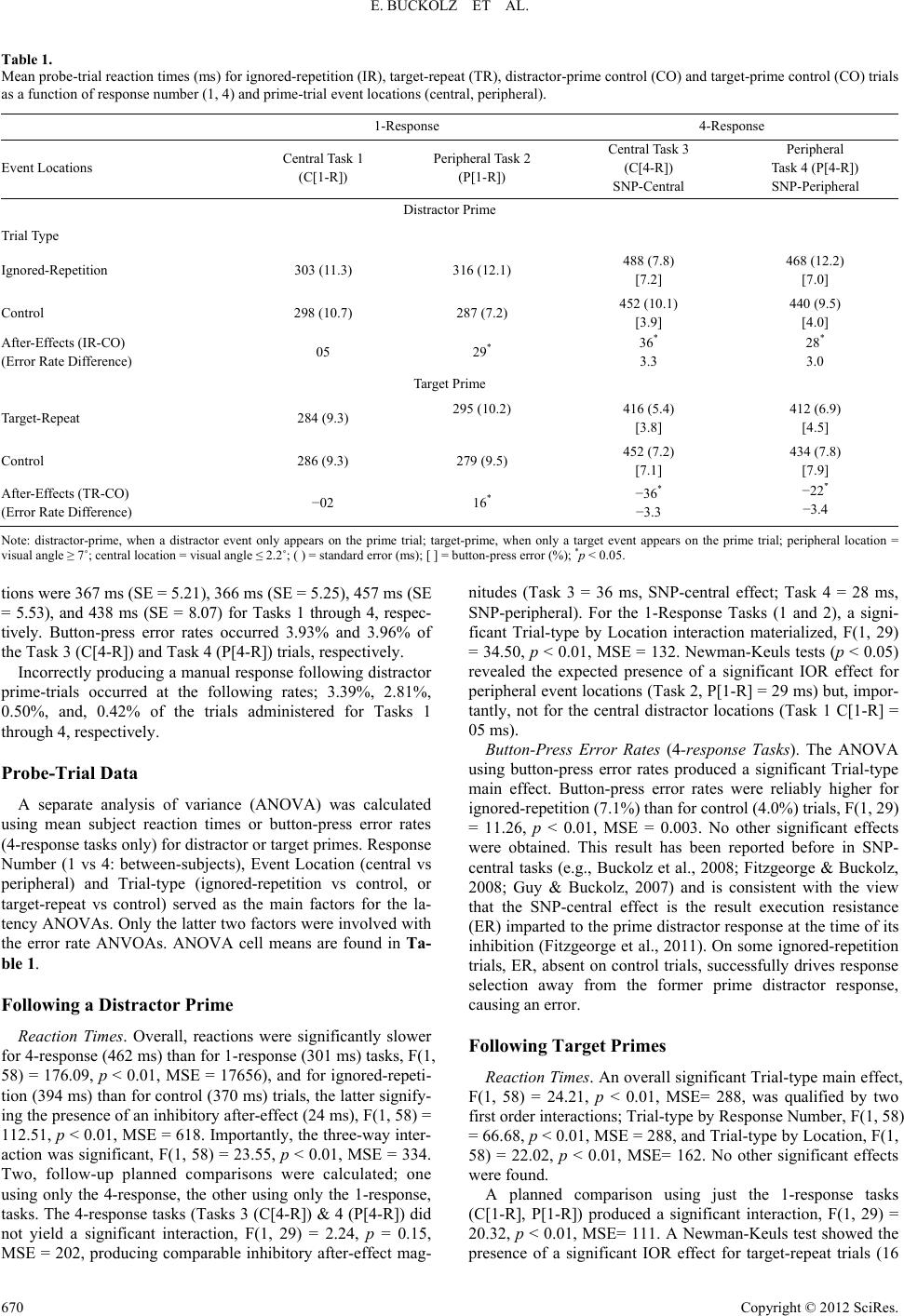 E. BUCKOLZ ET AL. Copyright © 2012 SciRes. 670 Table 1. Mean probe-trial reaction times (ms) for ignored-repetition (IR), target-repeat (TR), distractor-prime control (CO) and target-prime control (CO) trials as a function of response number (1, 4) and prime-trial event locations (central, peripheral). 1-Response 4-Response Event Locations Central Task 1 (C[1-R]) Peripheral Task 2 (P[1-R]) Central Task 3 (C[4-R]) SNP-Central Peripheral Task 4 (P[4-R]) SNP-Peripheral Distractor Prime Trial Type Ignored-Repetition 303 (11.3) 316 (12.1) 488 (7.8) [7.2] 468 (12.2) [7.0] Control 298 (10.7) 287 (7.2) 452 (10.1) [3.9] 440 (9.5) [4.0] After-Effects (IR-CO) (Error Rate Difference) 05 29* 36* 3.3 28* 3.0 Target Prime Target-Repeat 284 (9.3) 295 (10.2) 416 (5.4) [3.8] 412 (6.9) [4.5] Control 286 (9.3) 279 (9.5) 452 (7.2) [7.1] 434 (7.8) [7.9] After-Effects (TR-CO) (Error Rate Difference) −02 16* −36* −3.3 −22 −3.4 Note: distractor-prime, when a distractor event only appears on the prime trial; target-prime, when only a target event appears on the prime trial; peripheral location = visual angle ≥ 7˚; central location = visual angle ≤ 2.2˚; ( ) = standard error (ms); [ ] = button-press error (%); *p < 0.05. tions were 367 ms (SE = 5.21), 366 ms (SE = 5.25), 457 ms (SE = 5.53), and 438 ms (SE = 8.07) for Tasks 1 through 4, respec- tively. Button-press error rates occurred 3.93% and 3.96% of the Task 3 (C[4-R]) and Task 4 (P[4-R]) trials, respectively. Incorrectly producing a manual response following distractor prime-trials occurred at the following rates; 3.39%, 2.81%, 0.50%, and, 0.42% of the trials administered for Tasks 1 through 4, respectively. Probe-Trial Data A separate analysis of variance (ANOVA) was calculated using mean subject reaction times or button-press error rates (4-response tasks only) for distractor or target primes. Response Number (1 vs 4: between-subjects), Event Location (central vs peripheral) and Trial-type (ignored-repetition vs control, or target-repeat vs control) served as the main factors for the la- tency ANOVAs. Only the latter two factors were involved with the error rate ANVOAs. ANOVA cell means are found in Ta- ble 1. Following a Distractor Prime Reaction Times. Overall, reactions were significantly slower for 4-response (462 ms) than for 1-response (301 ms) tasks, F(1, 58) = 176.09, p < 0.01, MSE = 17656), and for ignored-repeti- tion (394 ms) than for control (370 ms) trials, the latter signify- ing the presence of an inhibitory after-effect (24 ms), F(1, 58) = 112.51, p < 0.01, MSE = 618. Importantly, the three-way inter- action was significant, F(1, 58) = 23.55, p < 0.01, MSE = 334. Two, follow-up planned comparisons were calculated; one using only the 4-response, the other using only the 1-response, tasks. The 4-response tasks (Tasks 3 (C[4-R]) & 4 (P[4-R]) did not yield a significant interaction, F(1, 29) = 2.24, p = 0.15, MSE = 202, producing comparable inhibitory after-effect mag- nitudes (Task 3 = 36 ms, SNP-central effect; Task 4 = 28 ms, SNP-peripheral). For the 1-Response Tasks (1 and 2), a signi- ficant Trial-type by Location interaction materialized, F(1, 29) = 34.50, p < 0.01, MSE = 132. Newman-Keuls tests (p < 0.05) revealed the expected presence of a significant IOR effect for peripheral event locations (Task 2, P[1-R] = 29 ms) but, impor- tantly, not for the central distractor locations (Task 1 C[1-R] = 05 ms). Button-Press Error Rates (4-response Tasks). The ANOVA using button-press error rates produced a significant Trial-type main effect. Button-press error rates were reliably higher for ignored-repetition (7.1%) than for control (4.0%) trials, F(1, 29) = 11.26, p < 0.01, MSE = 0.003. No other significant effects were obtained. This result has been reported before in SNP- central tasks (e.g., Buckolz et al., 2008; Fitzgeorge & Buckolz, 2008; Guy & Buckolz, 2007) and is consistent with the view that the SNP-central effect is the result execution resistance (ER) imparted to the prime distractor response at the time of its inhibition (Fitzgeorge et al., 2011). On some ignored-repetition trials, ER, absent on control trials, successfully drives response selection away from the former prime distractor response, causing an error. Following Target Primes Reaction Times. An overall significant Trial-type main effect, F(1, 58) = 24.21, p < 0.01, MSE= 288, was qualified by two first order interactions; Trial-type by Response Number, F(1, 58) = 66.68, p < 0.01, MSE = 288, and Trial-type by Location, F(1, 58) = 22.02, p < 0.01, MSE= 162. No other significant effects were found. A planned comparison using just the 1-response tasks (C[1-R], P[1-R]) produced a significant interaction, F(1, 29) = 20.32, p < 0.01, MSE= 111. A Newman-Keuls test showed the presence of a significant IOR effect for target-repeat trials (16
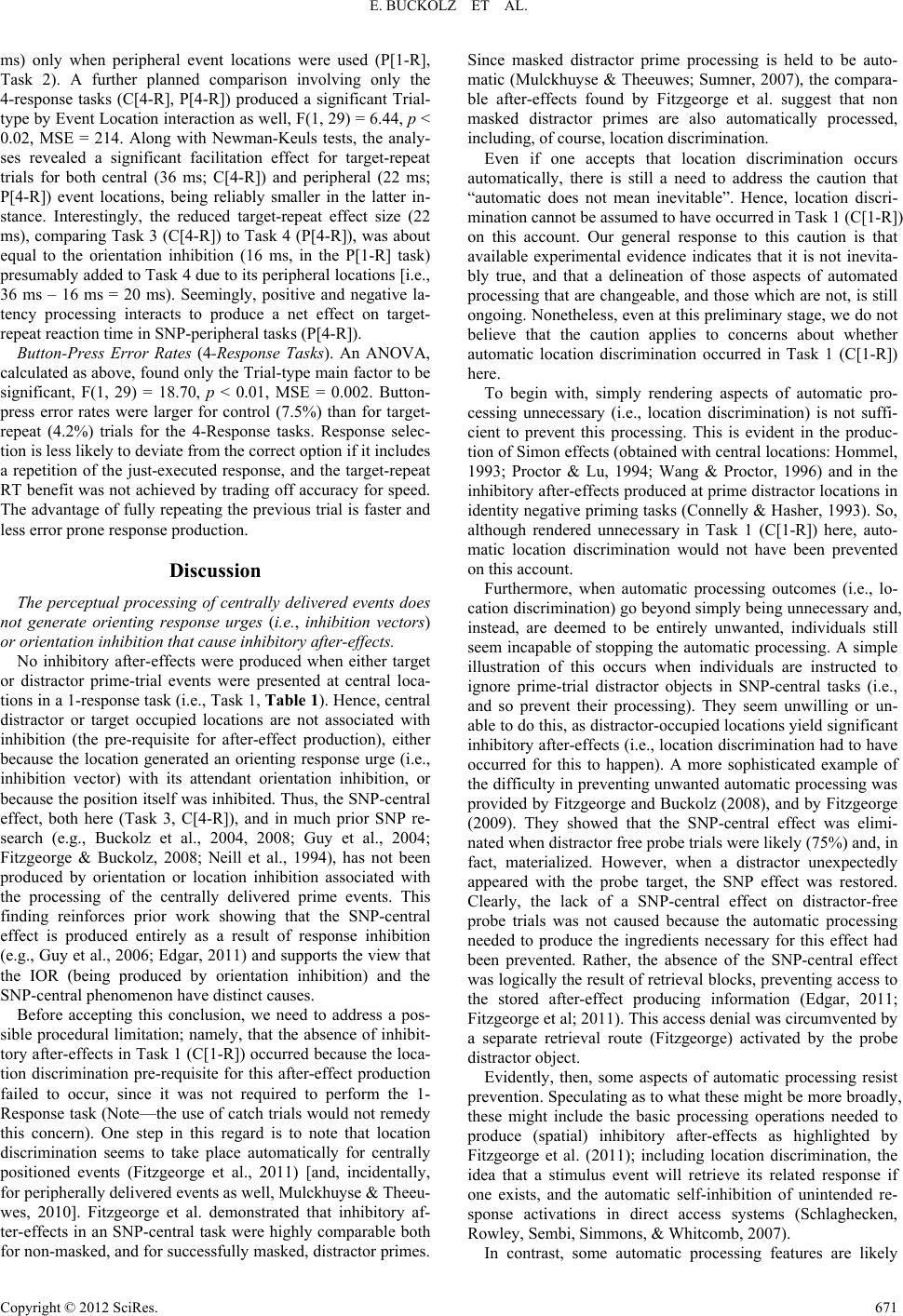 E. BUCKOLZ ET AL. ms) only when peripheral event locations were used (P[1-R], Task 2). A further planned comparison involving only the 4-response tasks (C[4-R], P[4-R]) produced a significant Trial- type by Event Location interaction as well, F(1, 29) = 6.44, p < 0.02, MSE = 214. Along with Newman-Keuls tests, the analy- ses revealed a significant facilitation effect for target-repeat trials for both central (36 ms; C[4-R]) and peripheral (22 ms; P[4-R]) event locations, being reliably smaller in the latter in- stance. Interestingly, the reduced target-repeat effect size (22 ms), comparing Task 3 (C[4-R]) to Task 4 (P[4-R]), was about equal to the orientation inhibition (16 ms, in the P[1-R] task) presumably added to Task 4 due to its peripheral locations [i.e., 36 ms – 16 ms = 20 ms). Seemingly, positive and negative la- tency processing interacts to produce a net effect on target- repeat reaction time in SNP-peripheral tasks (P[4-R]). Button-Press Error Rates (4-Response Tasks). An ANOVA, calculated as above, found only the Trial-type main factor to be significant, F(1, 29) = 18.70, p < 0.01, MSE = 0.002. Button- press error rates were larger for control (7.5%) than for target- repeat (4.2%) trials for the 4-Response tasks. Response selec- tion is less likely to deviate from the correct option if it includes a repetition of the just-executed response, and the target-repeat RT benefit was not achieved by trading off accuracy for speed. The advantage of fully repeating the previous trial is faster and less error prone response production. Discussion The perceptual processing of centrally delivered events does not generate orienting response urges (i.e., inhibition vectors) or orientation inhibition that cause inhibitory after-effects. No inhibitory after-effects were produced when either target or distractor prime-trial events were presented at central loca- tions in a 1-response task (i.e., Task 1, Table 1). Hence, central distractor or target occupied locations are not associated with inhibition (the pre-requisite for after-effect production), either because the location generated an orienting response urge (i.e., inhibition vector) with its attendant orientation inhibition, or because the position itself was inhibited. Thus, the SNP-central effect, both here (Task 3, C[4-R]), and in much prior SNP re- search (e.g., Buckolz et al., 2004, 2008; Guy et al., 2004; Fitzgeorge & Buckolz, 2008; Neill et al., 1994), has not been produced by orientation or location inhibition associated with the processing of the centrally delivered prime events. This finding reinforces prior work showing that the SNP-central effect is produced entirely as a result of response inhibition (e.g., Guy et al., 2006; Edgar, 2011) and supports the view that the IOR (being produced by orientation inhibition) and the SNP-central phenomenon have distinct causes. Before accepting this conclusion, we need to address a pos- sible procedural limitation; namely, that the absence of inhibit- tory after-effects in Task 1 (C[1-R]) occurred because the loca- tion discrimination pre-requisite for this after-effect production failed to occur, since it was not required to perform the 1- Response task (Note—the use of catch trials would not remedy this concern). One step in this regard is to note that location discrimination seems to take place automatically for centrally positioned events (Fitzgeorge et al., 2011) [and, incidentally, for peripherally delivered events as well, Mulckhuyse & Theeu- wes, 2010]. Fitzgeorge et al. demonstrated that inhibitory af- ter-effects in an SNP-central task were highly comparable both for non-masked, and for successfully masked, distractor primes. Since masked distractor prime processing is held to be auto- matic (Mulckhuyse & Theeuwes; Sumner, 2007), the compara- ble after-effects found by Fitzgeorge et al. suggest that non masked distractor primes are also automatically processed, including, of course, location discrimination. Even if one accepts that location discrimination occurs automatically, there is still a need to address the caution that “automatic does not mean inevitable”. Hence, location discri- mination cannot be assumed to have occurred in Task 1 (C[1-R]) on this account. Our general response to this caution is that available experimental evidence indicates that it is not inevita- bly true, and that a delineation of those aspects of automated processing that are changeable, and those which are not, is still ongoing. Nonetheless, even at this preliminary stage, we do not believe that the caution applies to concerns about whether automatic location discrimination occurred in Task 1 (C[1-R]) here. To begin with, simply rendering aspects of automatic pro- cessing unnecessary (i.e., location discrimination) is not suffi- cient to prevent this processing. This is evident in the produc- tion of Simon effects (obtained with central locations: Hommel, 1993; Proctor & Lu, 1994; Wang & Proctor, 1996) and in the inhibitory after-effects produced at prime distractor locations in identity negative priming tasks (Connelly & Hasher, 1993). So, although rendered unnecessary in Task 1 (C[1-R]) here, auto- matic location discrimination would not have been prevented on this account. Furthermore, when automatic processing outcomes (i.e., lo- cation discrimination) go beyond simply being unnecessary and, instead, are deemed to be entirely unwanted, individuals still seem incapable of stopping the automatic processing. A simple illustration of this occurs when individuals are instructed to ignore prime-trial distractor objects in SNP-central tasks (i.e., and so prevent their processing). They seem unwilling or un- able to do this, as distractor-occupied locations yield significant inhibitory after-effects (i.e., location discrimination had to have occurred for this to happen). A more sophisticated example of the difficulty in preventing unwanted automatic processing was provided by Fitzgeorge and Buckolz (2008), and by Fitzgeorge (2009). They showed that the SNP-central effect was elimi- nated when distractor free probe trials were likely (75%) and, in fact, materialized. However, when a distractor unexpectedly appeared with the probe target, the SNP effect was restored. Clearly, the lack of a SNP-central effect on distractor-free probe trials was not caused because the automatic processing needed to produce the ingredients necessary for this effect had been prevented. Rather, the absence of the SNP-central effect was logically the result of retrieval blocks, preventing access to the stored after-effect producing information (Edgar, 2011; Fitzgeorge et al; 2011). This access denial was circumvented by a separate retrieval route (Fitzgeorge) activated by the probe distractor object. Evidently, then, some aspects of automatic processing resist prevention. Speculating as to what these might be more broadly, these might include the basic processing operations needed to produce (spatial) inhibitory after-effects as highlighted by Fitzgeorge et al. (2011); including location discrimination, the idea that a stimulus event will retrieve its related response if one exists, and the automatic self-inhibition of unintended re- sponse activations in direct access systems (Schlaghecken, Rowley, Sembi, Simmons, & Whitcomb, 2007). In contrast, some automatic processing features are likely Copyright © 2012 SciRes. 671
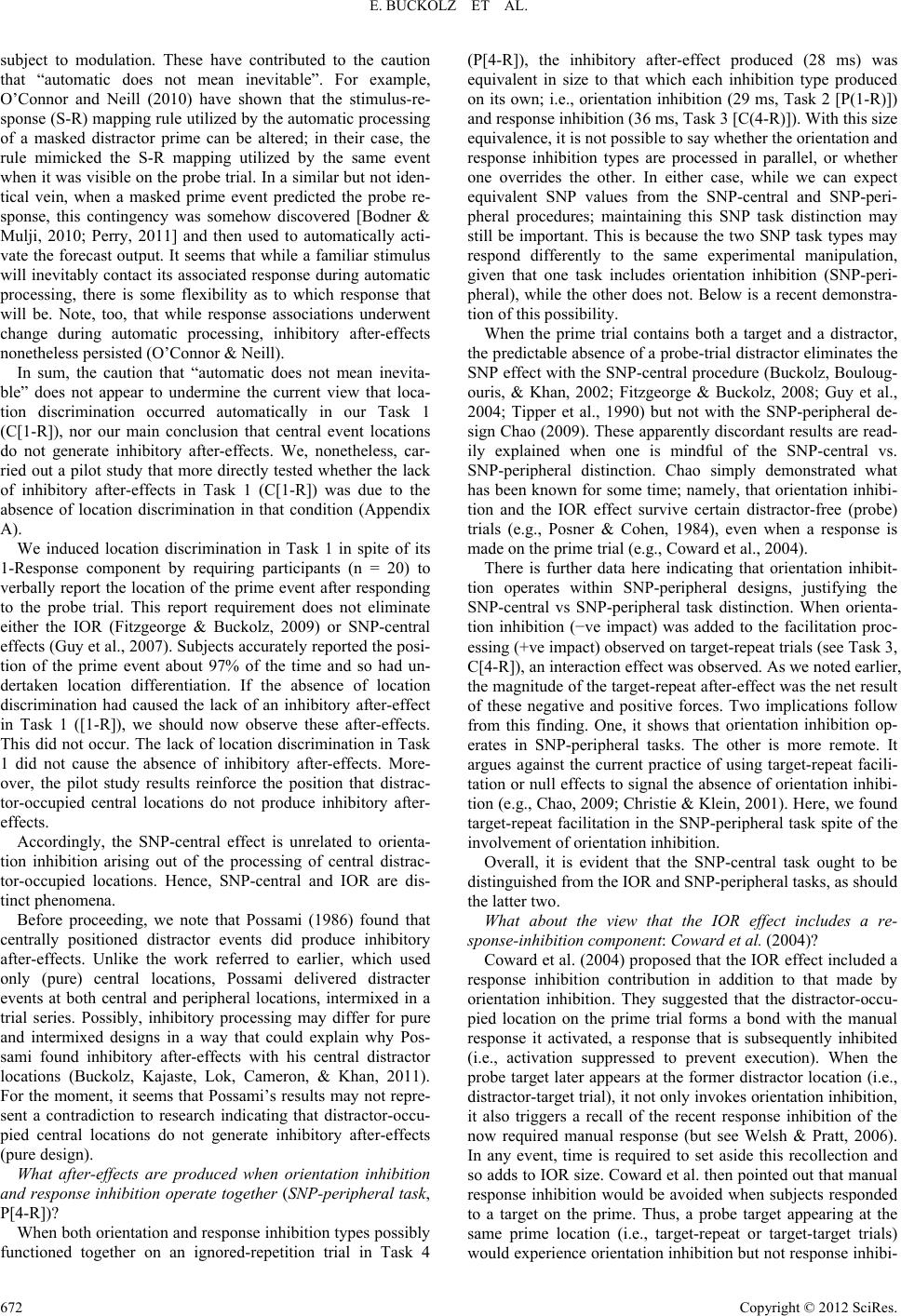 E. BUCKOLZ ET AL. subject to modulation. These have contributed to the caution that “automatic does not mean inevitable”. For example, O’Connor and Neill (2010) have shown that the stimulus-re- sponse (S-R) mapping rule utilized by the automatic processing of a masked distractor prime can be altered; in their case, the rule mimicked the S-R mapping utilized by the same event when it was visible on the probe trial. In a similar but not iden- tical vein, when a masked prime event predicted the probe re- sponse, this contingency was somehow discovered [Bodner & Mulji, 2010; Perry, 2011] and then used to automatically acti- vate the forecast output. It seems that while a familiar stimulus will inevitably contact its associated response during automatic processing, there is some flexibility as to which response that will be. Note, too, that while response associations underwent change during automatic processing, inhibitory after-effects nonetheless persisted (O’Connor & Neill). In sum, the caution that “automatic does not mean inevita- ble” does not appear to undermine the current view that loca- tion discrimination occurred automatically in our Task 1 (C[1-R]), nor our main conclusion that central event locations do not generate inhibitory after-effects. We, nonetheless, car- ried out a pilot study that more directly tested whether the lack of inhibitory after-effects in Task 1 (C[1-R]) was due to the absence of location discrimination in that condition (Appendix A). We induced location discrimination in Task 1 in spite of its 1-Response component by requiring participants (n = 20) to verbally report the location of the prime event after responding to the probe trial. This report requirement does not eliminate either the IOR (Fitzgeorge & Buckolz, 2009) or SNP-central effects (Guy et al., 2007). Subjects accurately reported the posi- tion of the prime event about 97% of the time and so had un- dertaken location differentiation. If the absence of location discrimination had caused the lack of an inhibitory after-effect in Task 1 ([1-R]), we should now observe these after-effects. This did not occur. The lack of location discrimination in Task 1 did not cause the absence of inhibitory after-effects. More- over, the pilot study results reinforce the position that distrac- tor-occupied central locations do not produce inhibitory after- effects. Accordingly, the SNP-central effect is unrelated to orienta- tion inhibition arising out of the processing of central distrac- tor-occupied locations. Hence, SNP-central and IOR are dis- tinct phenomena. Before proceeding, we note that Possami (1986) found that centrally positioned distractor events did produce inhibitory after-effects. Unlike the work referred to earlier, which used only (pure) central locations, Possami delivered distracter events at both central and peripheral locations, intermixed in a trial series. Possibly, inhibitory processing may differ for pure and intermixed designs in a way that could explain why Pos- sami found inhibitory after-effects with his central distractor locations (Buckolz, Kajaste, Lok, Cameron, & Khan, 2011). For the moment, it seems that Possami’s results may not repre- sent a contradiction to research indicating that distractor-occu- pied central locations do not generate inhibitory after-effects (pure design). What after-effects are produced when orientation inhibition and response inhibition operate together (SNP-peripheral task, P[4-R])? When both orientation and response inhibition types possibly functioned together on an ignored-repetition trial in Task 4 (P[4-R]), the inhibitory after-effect produced (28 ms) was equivalent in size to that which each inhibition type produced on its own; i.e., orientation inhibition (29 ms, Task 2 [P(1-R)]) and response inhibition (36 ms, Task 3 [C(4-R)]). With this size equivalence, it is not possible to say whether the orientation and response inhibition types are processed in parallel, or whether one overrides the other. In either case, while we can expect equivalent SNP values from the SNP-central and SNP-peri- pheral procedures; maintaining this SNP task distinction may still be important. This is because the two SNP task types may respond differently to the same experimental manipulation, given that one task includes orientation inhibition (SNP-peri- pheral), while the other does not. Below is a recent demonstra- tion of this possibility. When the prime trial contains both a target and a distractor, the predictable absence of a probe-trial distractor eliminates the SNP effect with the SNP-central procedure (Buckolz, Bouloug- ouris, & Khan, 2002; Fitzgeorge & Buckolz, 2008; Guy et al., 2004; Tipper et al., 1990) but not with the SNP-peripheral de- sign Chao (2009). These apparently discordant results are read- ily explained when one is mindful of the SNP-central vs. SNP-peripheral distinction. Chao simply demonstrated what has been known for some time; namely, that orientation inhibi- tion and the IOR effect survive certain distractor-free (probe) trials (e.g., Posner & Cohen, 1984), even when a response is made on the prime trial (e.g., Coward et al., 2004). There is further data here indicating that orientation inhibit- tion operates within SNP-peripheral designs, justifying the SNP-central vs SNP-peripheral task distinction. When orienta- tion inhibition (−ve impact) was added to the facilitation proc- essing (+ve impact) observed on target-repeat trials (see Task 3, C[4-R]), an interaction effect was observed. As we noted earlier, the magnitude of the target-repeat after-effect was the net result of these negative and positive forces. Two implications follow from this finding. One, it shows that orientation inhibition op- erates in SNP-peripheral tasks. The other is more remote. It argues against the current practice of using target-repeat facili- tation or null effects to signal the absence of orientation inhibi- tion (e.g., Chao, 2009; Christie & Klein, 2001). Here, we found target-repeat facilitation in the SNP-peripheral task spite of the involvement of orientation inhibition. Overall, it is evident that the SNP-central task ought to be distinguished from the IOR and SNP-peripheral tasks, as should the latter two. What about the view that the IOR effect includes a re- sponse-inhibition component: Coward et al. (2004)? Coward et al. (2004) proposed that the IOR effect included a response inhibition contribution in addition to that made by orientation inhibition. They suggested that the distractor-occu- pied location on the prime trial forms a bond with the manual response it activated, a response that is subsequently inhibited (i.e., activation suppressed to prevent execution). When the probe target later appears at the former distractor location (i.e., distractor-target trial), it not only invokes orientation inhibition, it also triggers a recall of the recent response inhibition of the now required manual response (but see Welsh & Pratt, 2006). In any event, time is required to set aside this recollection and so adds to IOR size. Coward et al. then pointed out that manual response inhibition would be avoided when subjects responded to a target on the prime. Thus, a probe target appearing at the same prime location (i.e., target-repeat or target-target trials) would experience orientation inhibition but not response inhibi- Copyright © 2012 SciRes. 672
 E. BUCKOLZ ET AL. tion. Consistent with this reasoning, Coward et al. found IOR to be significantly smaller for target-target than for distractor- target locations. We replicated this result (i.e., IOR was smaller for target-target [16] ms) than for distractor-target [29 ms] = trials) in Task 2, but not for Task 1. Possibly, central and pe- ripheral event location processing differ in another way; the latter, but not the former, causes a response inhibition IOR component. Conclusion Centrally-positioned, distractor-occupied locations do not cause inhibitory after-effects, showing, among other things, that such locations do not invoke “orientation inhibition”, held to primarily or exclusively cause the inhibition-of-return (IOR) phenomenon (e.g., Posner & Cohen, 1984). Accordingly, the production of spatial negative priming effect observed with central distractor events (SNP-central) has a cause that is dis- tinct from that of the IOR effect, a cause thought to result from the inhibitory after-effects resulting from the inhibition of the prime-trial distractor response (Guy et al., 2006; Edgar, 2011). REFERENCES Bodner, G. E., & Mulji, R. (2010). Prime proportion affects masked priming of fixed and free-choice responses. Experimental Psychol- ogy, 67, 360-366. Buckolz, E., Avramidis, C., & Fitzgeorge, L. (2008). Prime-trial de- mands and their impact on distractor processing in a spatial negative priming task. Psychological Research, 72, 235-248. doi:10.1007/s00426-007-0107-5 Buckolz, E., Boulougouris, A., & Khan, M. (2002). The influence of probe-trial selection requirements on the location negative priming effect. Canadian Journal of Experimental Psycho l og y, 56, 2774-283. doi:10.1037/h0087403 Buckolz, E., Goldfarb, A., & Khan, M. (2004). The use of a distrac- tor-assigned response slows later responding in a location negative priming task. Perception & Psychophysics, 66, 837-845. doi:10.3758/BF03194977 Buckolz, E., Kajaste, B., Lok, M., Edgar, C., & Khan, M. (2011). Do centrally presented stimulations cause orientation inhibition? Pre- sented to the North American Society for Psychology of Sport and Physical Activity. Burlington, Vermont. Chao, H. F. (2009). Revisiting the role of probe distracters in negative priming: Location negative priming is observed when probe distrac- ters are consistently absent. Attention, Perception, & Psychophysics, 71, 1072-1082. doi:10.3758/APP.71.5.1072 Christie, J., & Klein, R. (2001). Negative priming for spatial location? Canadian Journal of Experimental Psychology, 55, 24-38. doi:10.1037/h0087350 Connelly, S. L., & Hasher, L. (1993). Aging and the inhibition of spa- tial location. Journal of Experimental Psychology: Human Percep- tion and Performance, 19, 1238-1250. doi:10.1037/0096-1523.19.6.1238 Corneil, B. D., Munoz, D. P., Chapman, B. B., Admans, T., & Cushing, S. L. (2007). Neuromuscular consequences of reflexive covert ori- enting. Nature Neurosc i e n c e , 155, 13-15. Coward, R. S., Poliakoff, E., O’Boyle, D. J., & Lowe, C. L. (2004). The contribution of non-ocular response inhibition to visual inhibition of return. Experimental Brain Research, 155, 124-128. doi:10.1007/s00221-003-1803-z Edgar, C. (2011). Preventing response-based inhibition processing re- trieval: SNP disengagement. Masters Thesis, London: The University of Western Ontario. Fitzgeorge, L. (2009). Cognitive inhibition: Inhibitory after-effects. Doctoral Thesis, London: The University of Western Ontario. Fitzgeorge, L., & Buckolz, E. (2008). Spatial negative priming modula- tion: The influence of probe-trial target cueing, distractor presence and an intervening response. European Journal of Cognitive Psy- chology, 20, 994-1026. doi:10.1080/09541440701686250 Fitzgeorge, L., & Buckolz, E. (2009). Automatic versus volitional ori- enting and the production of the inhibition-of-return effect. Canadian Journal of Experimental Psychol og y, 63, 94-102. doi:10.1037/a0013700 Fitzgeorge, L., Buckolz, E., & Khan, M. (2011). Recently inhibited responses are avoided for both masked and non-masked primes in a spatial negative priming task. Attention, Perception, & Psychophys- ics, 73, 1435-1452. doi:10.3758/s1341-011-0125-7 Guy, S., & Buckolz, E. (2007). The locus and modulation of the loca- tion negative priming effect. Psychological Research, 71, 178-191. doi:10.1007/s00426-005-0003-9 Guy, S., Buckolz, E., & Fitzgeorge, L. (2007). Disengaging the location negative priming effect: The influence of an intervening response. European Journal of Cognitive Psychology, 19, 789-812. doi:10.1080/09541440600959287 Guy, S., Buckolz, E., & Khan, M. (2006). The locus of location repeti- tion latency effects. Canadian Journal of Experimental Psychology, 60, 307-318. doi:10.1037/cjep2006028 Guy, S., Buckolz, E., & Pratt, J. (2004). The influence of distractor- only prime trials on the location negative priming mechanism. Ex- perimental Psychology, 51, 4-14. doi:10.1027/1618-3169.51.1.4 Hommel, B. (1993). The role of attention for the Simon effect. Psycho- logical Research, 55, 208-222. doi:10.1007/BF00419608 Klein, R., Christie, J., & Morris, E. P. (2005). Vector averaging of inhibition of return. Psychonomic Bulletin & Review, 12, 295-300. doi:10.3758/BF03196375 Lupianez, J., Klein, R., & Bartolomeo, P. (2006). Inhibition of return: Twenty years after. Cognitive Neuropsychology, 23, 1003-1014. doi:10.1080/02643290600588095 Milliken, B., Tipper, S. P., Houghton, G., & Lupianez, J. (2000). At- tending, ignoring, and repetition: On the relation between negative priming and inhibition of return. Perception & Psychophysics, 62, 1289-1296. doi:10.3758/BF03212130 Mulckhuyse, M., & Theeuwes, J. (2010). Unconscious attentional ori- enting to exogenous cues: A review of the literature. Acta Psy- chologica, 134, 200-309. doi:10.1016/j.actpsy.2010.03.002 Neill, W. T., Terry, K. M., & Valdes, L. A. (1994). Negative priming without probe selection. Psychonomic Bulletin and Review, 1, 119- 121. doi:10.3758/BF03200767 O’Connor, P. A., & Neill, W. T. (2010). Does subliminal priming of free response choices depend on taskset or automatic response acti- vation? Conscious nes s a n d Cognition, 20, 280-287. doi:10.1016/j.concog.2010.08.007 Perry, J. (2011). An investigation of masked priming mechanisms in binary classification tasks. Ph.D. Thesis, London: The University of Western Ontario. Posner, M. I., & Cohen, Y. (1984). Components of visual orienting. In H. Bouma, & D. G. Bouwhuis (Eds.), Attention and performance X (pp. 531-556). Hillsdale, NJ: Lawrence Earlbaum Assoc. Posner, M. I., Rafal, R. D., Choate, L. S., & Vaughan, J. (1985). Inhibi- tion of return: Neural basis and function. Cognitive Neuropsychology, 2, 211-228. doi:10.1080/02643298508252866 Proctor, R. W., & Lu, C.-H. (1994). Referential coding and atten- tion-shifting accounts of the Simon effect. Psychological Research, 56, 185-195. doi:10.1007/BF00419706 Possami, C.-A. (1986). Relationship between inhibition and facilitation following a visual cue. Acta Psychologica, 61, 243-258. doi:10.1016/0001-6918(86)90084-3 Rafal, R., Calabresi, P., Brennan, C., & Sciolto, T. (1989). Saccade preparation inhibits reorienting to recently attended locations. Jour- nal of Experimental Psychology: Human Perception and Perform- ance, 15, 673-685. doi:10.1037/0096-1523.15.4.673 Rafal, R., Davies, J., & Lauder, J. (2006). Inhibitory tagging at subse- quently fixated locations: Generation of “inhibition of return” with- out saccade inhibition. Visual Cognition, 13, 308-323. doi:10.1080/13506280544000011 Schlaghecken, F., Rowley, L., Sembi, S., Simmons, R., & Whitcomb, D. (2007). The negative compatibility effect: A case for self-inhibition. Copyright © 2012 SciRes. 673
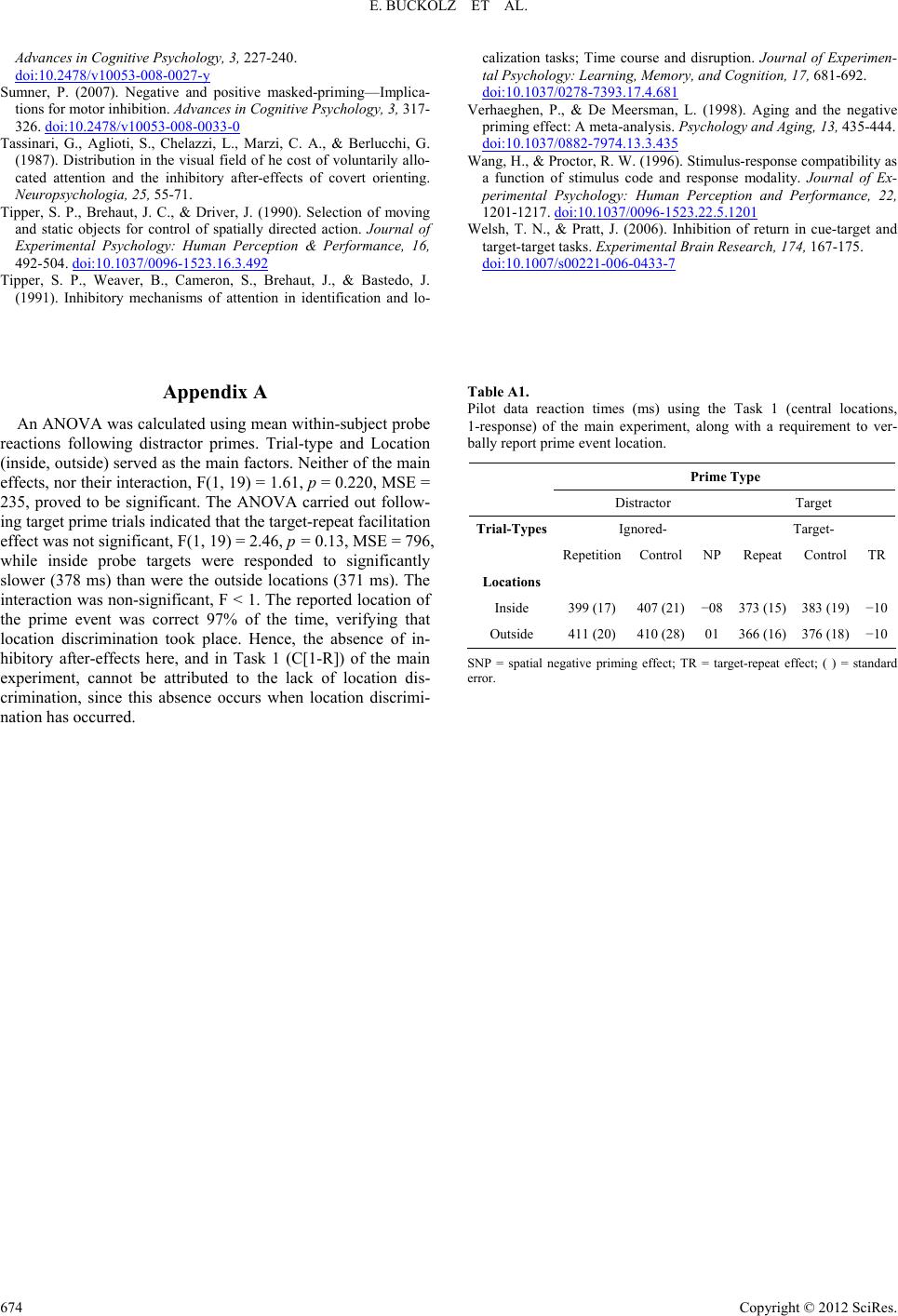 E. BUCKOLZ ET AL. Copyright © 2012 SciRes. 674 Advances in Cognitive Psychology, 3, 227-240. doi:10.2478/v10053-008-0027-y Sumner, P. (2007). Negative and positive masked-priming—Implica- tions for motor inhibition. Advances in Cognitive Psychology, 3, 317- 326. doi:10.2478/v10053-008-0033-0 Tassinari, G., Aglioti, S., Chelazzi, L., Marzi, C. A., & Berlucchi, G. (1987). Distribution in the visual field of he cost of voluntarily allo- cated attention and the inhibitory after-effects of covert orienting. Neuropsychologia, 25, 55-71. Tipper, S. P., Brehaut, J. C., & Driver, J. (1990). Selection of moving and static objects for control of spatially directed action. Journal of Experimental Psychology: Human Perception & Performance, 16, 492-504. doi:10.1037/0096-1523.16.3.492 Tipper, S. P., Weaver, B., Cameron, S., Brehaut, J., & Bastedo, J. (1991). Inhibitory mechanisms of attention in identification and lo- calization tasks; Time course and disruption. Journal of Experimen- tal Psychology: Learning, Memory, and Cognition, 17, 681-692. doi:10.1037/0278-7393.17.4.681 Verhaeghen, P., & De Meersman, L. (1998). Aging and the negative priming effect: A meta-analysis. Psychology an d Aging, 13, 435-444. doi:10.1037/0882-7974.13.3.435 Wang, H., & Proctor, R. W. (1996). Stimulus-response compatibility as a function of stimulus code and response modality. Journal of Ex- perimental Psychology: Human Perception and Performance, 22, 1201-1217. doi:10.1037/0096-1523.22.5.1201 Welsh, T. N., & Pratt, J. (2006). Inhibition of return in cue-target and target-target tasks. Experimental Brain Research, 174, 167-175. doi:10.1007/s00221-006-0433-7 Appendix A An ANOVA was calculated using mean within-subject probe reactions following distractor primes. Trial-type and Location (inside, outside) served as the main factors. Neither of the main effects, nor their interaction, F(1, 19) = 1.61, p = 0.220, MSE = 235, proved to be significant. The ANOVA carried out follow- ing target prime trials indicated that the target-repeat facilitation effect was not significant, F(1, 19) = 2.46, p = 0.13, MSE = 796, while inside probe targets were responded to significantly slower (378 ms) than were the outside locations (371 ms). The interaction was non-significant, F < 1. The reported location of the prime event was correct 97% of the time, verifying that location discrimination took place. Hence, the absence of in- hibitory after-effects here, and in Task 1 (C[1-R]) of the main experiment, cannot be attributed to the lack of location dis- crimination, since this absence occurs when location discrimi- nation has occurred. Table A1. Pilot data reaction times (ms) using the Task 1 (central locations, 1-response) of the main experiment, along with a requirement to ver- bally report prime event location. Prime Type Distractor Target Trial-Types Ignored- Target- RepetitionControl NP Repeat ControlTR Locations Inside 399 (17)407 (21) −08 373 (15) 383 (19)−10 Outside 411 (20)410 (28) 01 366 (16) 376 (18)−10 SNP = spatial negative priming effect; TR = target-repeat effect; ( ) = standard error.
|