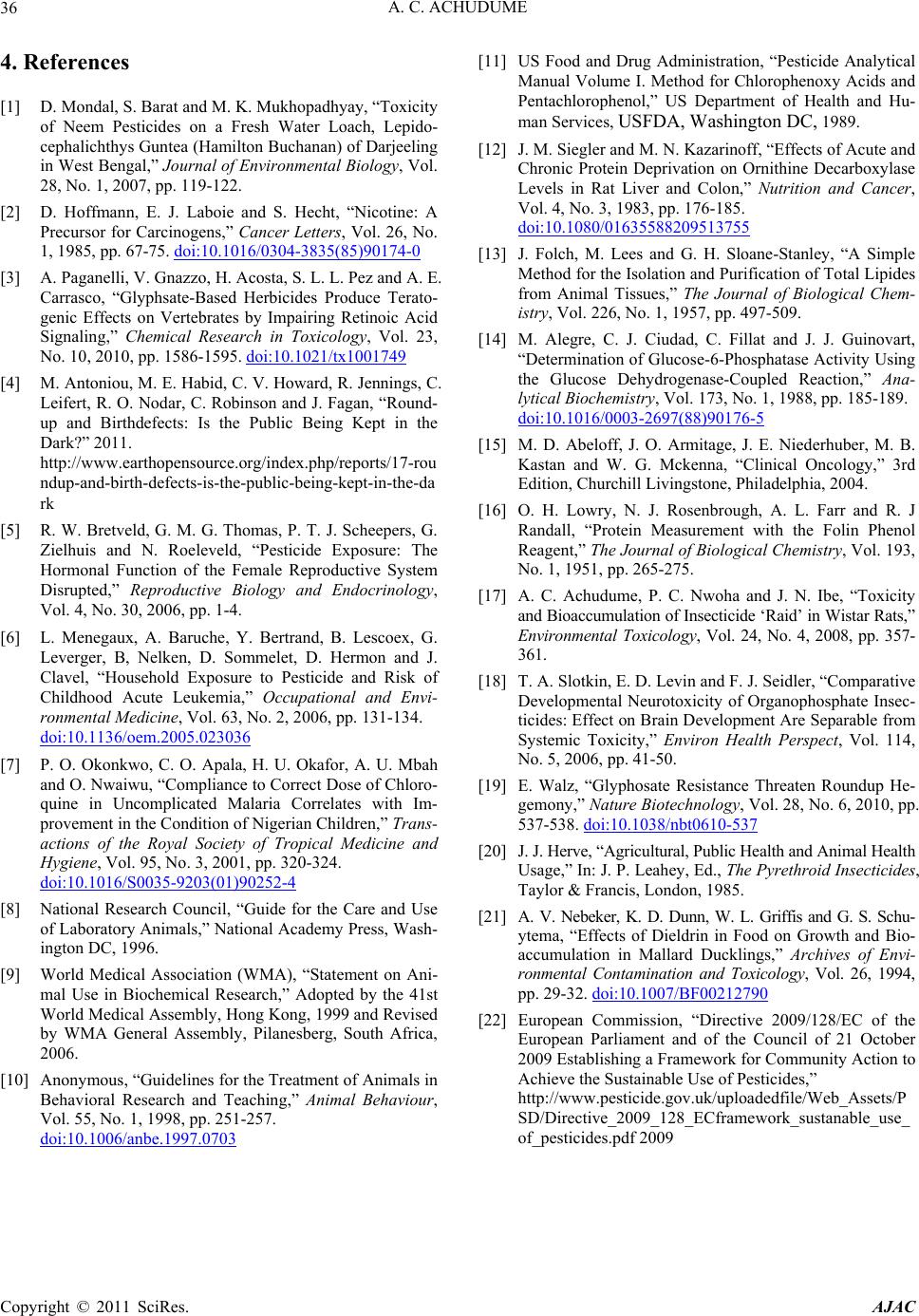
A. C. ACHUDUME
36
4. References
[1] D. Mondal, S. Barat and M. K. Mukhopadhyay, “Toxicity
of Neem Pesticides on a Fresh Water Loach, Lepido-
cephalichthys Guntea (Hamilton Buchanan) of Darjeeling
in West Bengal,” Journal of Environmental Biology, Vol.
28, No. 1, 2007, pp. 119-122.
[2] D. Hoffmann, E. J. Laboie and S. Hecht, “Nicotine: A
Precursor for Carcinogens,” Cancer Letters, Vol. 26, No.
1, 1985, pp. 67-75. doi:10.1016/0304-3835(85)90174-0
[3] A. Paganelli, V. Gnazzo, H. Acosta, S. L. L. Pez and A. E.
Carrasco, “Glyphsate-Based Herbicides Produce Terato-
genic Effects on Vertebrates by Impairing Retinoic Acid
Signaling,” Chemical Research in Toxicology, Vol. 23,
No. 10, 2010, pp. 1586-1595. doi:10.1021/tx1001749
[4] M. Antoniou, M. E. Habid, C. V. Howard, R. Jennings, C.
Leifert, R. O. Nodar, C. Robinson and J. Fagan, “Round-
up and Birthdefects: Is the Public Being Kept in the
Dark?” 2011.
http://www.earthopensource.org/index.php/reports/17-rou
ndup-and-birth-defects-is-the-public-being-kept-in-the-da
rk
[5] R. W. Bretveld, G. M. G. Thomas, P. T. J. Scheepers, G.
Zielhuis and N. Roeleveld, “Pesticide Exposure: The
Hormonal Function of the Female Reproductive System
Disrupted,” Reproductive Biology and Endocrinology,
Vol. 4, No. 30, 2006, pp. 1-4.
[6] L. Menegaux, A. Baruche, Y. Bertrand, B. Lescoex, G.
Leverger, B, Nelken, D. Sommelet, D. Hermon and J.
Clavel, “Household Exposure to Pesticide and Risk of
Childhood Acute Leukemia,” Occupational and Envi-
ronmental Medicine, Vol. 63, No. 2, 2006, pp. 131-134.
doi:10.1136/oem.2005.023036
[7] P. O. Okonkwo, C. O. Apala, H. U. Okafor, A. U. Mbah
and O. Nwaiwu, “Compliance to Correct Dose of Chloro-
quine in Uncomplicated Malaria Correlates with Im-
provement in the Condition of Nigerian Children,” Trans-
actions of the Royal Society of Tropical Medicine and
Hygiene, Vol. 95, No. 3, 2001, pp. 320-324.
doi:10.1016/S0035-9203(01)90252-4
[8] National Research Council, “Guide for the Care and Use
of Laboratory Animals,” National Academy Press, Wash-
ington DC, 1996.
[9] World Medical Association (WMA), “Statement on Ani-
mal Use in Biochemical Research,” Adopted by the 41st
World Medical Assembly, Hong Kong, 1999 and Revised
by WMA General Assembly, Pilanesberg, South Africa,
2006.
[10] Anonymous, “Guidelines for the Treatment of Animals in
Behavioral Research and Teaching,” Animal Behaviour,
Vol. 55, No. 1, 1998, pp. 251-257.
doi:10.1006/anbe.1997.0703
[11] US Food and Drug Administration, “Pesticide Analytical
Manual Volume I. Method for Chlorophenoxy Acids and
Pentachlorophenol,” US Department of Health and Hu-
man Services, USFDA, Washington DC, 1989.
[12] J. M. Siegler and M. N. Kazarinoff, “Effects of Acute and
Chronic Protein Deprivation on Ornithine Decarboxylase
Levels in Rat Liver and Colon,” Nutrition and Cancer,
Vol. 4, No. 3, 1983, pp. 176-185.
doi:10.1080/01635588209513755
[13] J. Folch, M. Lees and G. H. Sloane-Stanley, “A Simple
Method for the Isolation and Purification of Total Lipides
from Animal Tissues,” The Journal of Biological Chem-
istry, Vol. 226, No. 1, 1957, pp. 497-509.
[14] M. Alegre, C. J. Ciudad, C. Fillat and J. J. Guinovart,
“Determination of Glucose-6-Phosphatase Activity Using
the Glucose Dehydrogenase-Coupled Reaction,” Ana-
lytical Biochemistry, Vol. 173, No. 1, 1988, pp. 185-189.
doi:10.1016/0003-2697(88)90176-5
[15] M. D. Abeloff, J. O. Armitage, J. E. Niederhuber, M. B.
Kastan and W. G. Mckenna, “Clinical Oncology,” 3rd
Edition, Churchill Livingstone, Philadelphia, 2004.
[16] O. H. Lowry, N. J. Rosenbrough, A. L. Farr and R. J
Randall, “Protein Measurement with the Folin Phenol
Reagent,” The Journal of Biological Chemistry, Vol. 193,
No. 1, 1951, pp. 265-275.
[17] A. C. Achudume, P. C. Nwoha and J. N. Ibe, “Toxicity
and Bioaccumulation of Insecticide ‘Raid’ in Wistar Rats,”
Environmental Toxicology, Vol. 24, No. 4, 2008, pp. 357-
361.
[18] T. A. Slotkin, E. D. Levin and F. J. Seidler, “Comparative
Developmental Neurotoxicity of Organophosphate Insec-
ticides: Effect on Brain Development Are Separable from
Systemic Toxicity,” Environ Health Perspect, Vol. 114,
No. 5, 2006, pp. 41-50.
[19] E. Walz, “Glyphosate Resistance Threaten Roundup He-
gemony,” Nature Biotechnology, Vol. 28, No. 6, 2010, pp.
537-538. doi:10.1038/nbt0610-537
[20] J. J. Herve, “Agricultural, Public Health and Animal Health
Usage,” In: J. P. Leahey, Ed., The Pyrethroid Insecticides,
Taylor & Francis, London, 1985.
[21] A. V. Nebeker, K. D. Dunn, W. L. Griffis and G. S. Schu-
ytema, “Effects of Dieldrin in Food on Growth and Bio-
accumulation in Mallard Ducklings,” Archives of Envi-
ronmental Contamination and Toxicology, Vol. 26, 1994,
pp. 29-32. doi:10.1007/BF00212790
[22] European Commission, “Directive 2009/128/EC of the
European Parliament and of the Council of 21 October
2009 Establishing a Framework for Community Action to
Achieve the Sustainable Use of Pesticides,”
http://www.pesticide.gov.uk/uploadedfile/Web_Assets/P
SD/Directive_2009_128_ECframework_sustanable_use_
of_pesticides.pdf 2009
Copyright © 2011 SciRes. AJAC