Fatty Acids in Heterocyclic Synthesis. Part XVII: Synthesis of Non Ionic Surfactants Containing Piperidine, Piperazine, Imidazole Based on Thiadiazole and Microbiological Activities Evaluation ()
1. Introduction
1,3,4-Thiadiazole and its derivatives continue to be of great interest owing to their great pharmaceutical and industrial importance. The specific pharmacological activities include antioxidant [1] , anticancer [2] [3] , anti-inflammatory [4] [5] , antimicrobial [6] [7] , anti-HIV [8] [9] , antiviral [10] , anticonvulsant [11] [12] . Addition of the long alkyl chain (hydrophobic part) and propylene oxide (lipophilic part), to the Thiadiazole nucleus increases its solubility in water and enhances the potential of their usage as nonionic surfactants. Surfactants are one of the most important and widely used products in industry. This prompted us to continue our research program about the utilization of fatty acids in heterocyclic synthesis, to synthesize novel nonionic surfactants [13] - [19] . Herein, we report the synthesis and some reactions of 2-chloro-N-(5-heptadecyl-1,3,5 Thiadiazole-2-yl) acetamide (2) with piperidine, piperazine, urea, Thiourea and Potassium thiocyanate then propylene oxide was added in quantitative amounts to produce new types from Nonionic surfactants.
2. Experimental Protocols
2.1. Materials
Stearic acid, Urea, Thiourea, Acetone, Benzaldehyde, Benzene, Ethanol, Anhydrous potassium carbonate, Anhydrous sodium acetate (Adwic 99%), Phosphorus oxy chloride (Alphachemie, 98%), Choloro acetyl chloride (Loba chemie, 98%), Thiosemicarbazide (Oxford lab chem., 98%), Triethyl amine(Loba chemie, 98%), Potassium thiocyanate (Loba chemie, 98%), Pipridine (SdFine-chem. limited, 99%), Piperazine (Loba chemie, 98%), Glacial acetic acid (SdFine-chem. limited, 99%).
2.2. Structures Elucidation
The new compounds structure confirmation is established via elemental analysis detected by using automatic CHNS apparatus as well as different spectroscopic tools (MS, IR, 1H-NMR spectra). All melting points are determined by the open capillary method and are uncorrected. All mass spectra of the synthesized compounds were recorded on a Shimadzu GCMS-QP-1000EXmass spectrophotometer, IR spectra (KBr disk) of the new compounds were checked on JASCO FT/IR-4100 (Japan). 1H-NMR spectra were recorded in Varian Mercury VXR-300 spectrometer using TMS as internal reference. The surface active properties were carried out at the Chemistry Department, Faculty of Science, Benha University, Egypt. Antibacterial and antifungal activities were carried out in the Micro Analytical Center, Faculty of Science, Azhar University, Egypt.
2.3. Synthesis of 5-Heptadecyl-1,3,4-Thiadiazole-2-Amine (1)
A solution of stearic acid (0.01 mol) and Thiosemicarbazide (0.01 mol) in (20 mL) POCl3 was heated for 6 h. The reaction mixture was concentrated, cooled, and then poured onto crushed ice while stirring. The produced solid was filtered off and recrystallized from ethanol. Compound 1 was obtained as a yellow powder in 75% yield, mp 108˚C - 110˚C, IR (KBR): υ3376, 3267 (NH2), 2919 (CHaliphatic), 1686, 1663 (C=N) cm−1. 1H-NMR (DMSO-d6): δ 6.92 (s, 2H, D2o exchangeable, NH2), δ 1.24 (m, 32H, 16CH2), δ 0.88 (t, 3H, CH3). MS m/z (%): 340 (Ṁ++1, 1.81) 339 (Ṁ+, 1.8), 239 (0.67), 115 (100), 100 (0.15), 76 (0.9).
Anal. Calc. (%) for C19H37N3S: C, 67.20, H, 10.89, N, 12.37. Found: C, 67.31, H, 10.95, N, 12.50.
2.4. Synthesis of 2-Chloro-N-(5-Heptadecyl-1,3,5 Thiadiazole-2-yl) Acetamide (2)
Equimolar quantities of compound 1 and chloroacetylcholoride in dry benzene (30 mL) in the presence of Triethyl amine (0.1 mL) was boiled for 7 h. The reaction mixture was concentrated, cooled. The obtained solid product was filtered off, dried and recrystallized from ethanol. Compound 2 was obtained as a pale yellow powder in 80% yield, mp 120˚C - 122˚C.
IR (KBR): υ3374, (NH), 2850 (CHaliphatic), 1681 (C=O), 1658 (C=N) cm−1. 1H-NMR (DMSO-d6): δ 6.9 (s, H, D2o exchangeable, NH), δ 4.27 (s, 2H, CH2Cl), δ 1.24 (m, 32H, 16CH2), δ 0.88 (t, 3H, CH3).
MS m/z (%): 417 (Ṁ++2, 11.8), 415 (Ṁ+, 14.3), 339 (0.67), 324 (0.48), 239 (0.34), 115 (100), 93 (0.74).
Anal. Calc. (%) for C21H38ClN3OS: C, 60.62, H, 9.21, N, 10.10, Cl, 8.52. Found: C, 60.74, H, 9.10, N, 10.20, Cl, 8.40.
2.5. Synthesis of N-(5-Heptadecyl-1,3,4-Thiadiazol-2-yl)-2-(Piperidin-1-yl) Acetamide (3)
A solution of compound 2 (0.01 mol) and Piperidine (0.01 mol) in ethanol (30 ml) in the presence of anhydrous Potassium carbonate (0.5 gm), was heated for 6 h. The reaction mixture was concentrated, cooled. The solid product obtained was filtered off, dried and recrystallized from ethanol. Compound 3 was obtained as a yellow powder in 82% yield, mp 90˚C - 92˚C. IR (KBR):υ3279, (NH), 2920, 2851 (CHaliphatic), 1674 (C=O), 1665 (C=N) cm−1.
1H-NMR (DMSO-d6): δ 6.90 (s, 1H, D2o exchangeable, NH), δ 3.26 (s, 2H, CH2), δ 2.51 (t, 4H, CH2-N-CH2), δ 1.6 (t, 6H, 3CH2), δ 1.24 (m, 32H, 16CH2), δ 0.88 (t, 3H, CH3). MS m/z (%): 464 (Ṁ+, 1.21), 380 (0.18), 338 (2.72), 265 (1.01), 115 (100), 84 (13.71).
Anal. Calc. (%) for C26H48N4SO: C, 67.19, H, 10.41, N, 12.06. Found: C, 67.32, H, 10.55, N, 12.21.
2.6. Synthesis of N-(5-Heptadecyl-1,3,4-Thiadiazol-2-yl)-2-(Piperazin-1-yl) Acetamide (4)
Equimolar amounts of compound 2 and piperazine in the presence of anhydrous Potassium carbonate (0.5 gm), was heated for 6 h. The reaction mixture was concentrated, cooled. The solid product obtained was filtered off, dried and recrystallized from ethanol. Compound 4 was obtained as a yellow powder in 82% yield, mp 86˚C - 88˚C.
IR (KBR): υ3281, 3110 (NH’s), 2919, 2850 (CHaliphatic), 1680 (C=O), 1658 (C=N) cm−1. 1H-NMR (DMSO-d6): δ 10.5, 6.85 (s, 1H, NH), δ 3.26 (s, 2H, CH2), δ 2.6 (t, 4H, CH2-NH-CH2), δ 2.3 (t, 4H, CH2-N-CH2), δ 1.25 (m, 32H, 16CH2), δ 0.88 (t, 3H, CH3).
MS m/z (%): 466 (Ṁ++1, 0.18), 465 (M+, 0.92), 380 (0.95), 339 (2.86), 265 (1.56), 115 (100), 85 (16. 18).
Anal. Calc. (%) for C25H47N5OS: C, 64.47, H, 10.17, N, 15.04. Found: C, 64.60, H, 10.02, N, 15.20.
2.7. Synthesis of 3-(5-Heptadecyl-1,3,4 Thiadiazol-2-yl) Imidazolidine-2,4-Dione (5).
It was obtained from reaction of compound 2 with Urea in ethanol (30 ml) in the presence of anhydrous Potassium carbonate (0.5 gm), and was boiled for 10 h. The reaction mixture was concentrated, cooled. The solid product obtained was filtered off, dried and recrystallized from ethanol. Compound 5 was obtained as a pale yellow powder in 80% yield, mp 96˚C - 98˚C.
IR (KBR): υ3365 (NH), 2920, 2851 (CHaliphatic), 1680, 1672 (C=O), 1658 (C=N) cm−1. 1H-NMR (DMSO-d6): δ 6.96, (s, 1H, D2o exchangeable, NH), δ 5.4 (s, 2H, CH2), δ 1.24 (m, 32H, 16CH2), δ 0.85 (t, 3H, CH3).
MS m/z (%):423 (Ṁ++1, 6.56), 324 (6.08), 239 (1.56), 115 (100), 99 (2.86).
Anal. Calc. (%) for C22H38N4O2S: C, 62.52, H, 9.06, N, 13.26. Found: C, 62.66, H, 8.95, N, 13.02.
2.8. Synthesis of (Z)-5-Benzylidene-3-(5-Heptadecyl-1,3,4 Thiadiazol-2-yl) Imidazolidine-2,4-Dione (6)
A solution of compound 5 (0.01 mol) and benzaldhyde (0.01 mol) in glacial acetic acid (5 ml) in the presence of anhydrous Sodium acetate (0.8 gm) is heated under reflux for 12 h. A precipitate is formed, after cooling, the solid is filtered off, recrystallized from acetic acid to afford Compound 6 as a white powder in 72% yield, mp 82˚C - 84˚C.
IR (KBR): υ3284 (NH), 2850 (CHaliphatic), 1683, 1676 (C=O), 1673 (C=N) cm−1. 1H-NMR (DMSO-d6): δ 6.94, (s, 1H, D2o exchangeable, NH), δ 7.6-7.3 (m, 5H, ArH), δ 5.9 (s, 1H, =CH), δ 1.24 (m, 32H, 16CH2), δ 0.88 (t, 3H, CH3).
MS m/z (%): 510 (Ṁ+, 0.03), 421 (0.03), 324 (0.06), 115 (100), 89 (0.7).
Anal. Calc. (%) for C29H42N4O2S: C, 68.20, H, 8.29, N, 10.97. Found: C, 68.35, H, 8.02, N, 11.04.
2.9. Synthesis of 4-((5-Heptadecyl-1,3,4-Thiadiazol-2-yl) Amino)-H-imidazole-2(5H)-Thione (7)
Equimolar amounts of compound 2 and Thiourea in ethanol (30 ml) in the presence of anhydrous Potassium carbonate (0.5 gm), was heated under refluxed for 10 h. The reaction mixture was concentrated, cooled. The solid product obtained was filtered off, dried and recrystallized from ethanol. Compound 7 was obtained as a yellow powder in 75% yield, mp 100˚C - 102˚C.
IR (KBR): υ3370, 3177, (NH), 2920 (CHaliphatic), 1662, 1658 (C=N), 1205 (C=S) cm−1. 1H-NMR (DMSO-d6): δ 10.25, 6.96 (s, 1H, 2NH), δ 2.78 (s, 2H, CH2), δ 1.24 (m, 32H, 16CH2), δ 0.87 (t, 3H, CH3).
MS m/z (%): 437 (Ṁ+, 1.97), 339 (2.42), 323 (0.47), 115 (100).
Anal. Calc. (%) for C22H39N5S2: C, 60.37, H, 8.98, N; 16.00. Found: C, 60.52, H, 8.82, N, 16.12.
2.10. Synthesis of 3-(5-Heptadecyl-1,3,4-Thiadiazol-2-yl)-2 Imino Thiazolidin-4-One (8)
It was obtained from reaction of compound 2 with Potassium thiocyanate in acetone (30 ml) in the presence of anhydrous Potassium carbonate (0.5 gm), was heated for 2 h. The reaction mixture was concentrated, cooled. The solid product obtained was filtered off, dried and recrystallized from ethanol. Compound 8 was obtained as a yellow powder in 70% yield, mp 80˚C - 82˚C.
IR (KBR): υ3189, (=NH), 2919 (CHaliphatic), 1680 (C=O), 1659 (C=N) cm−1. 1H-NMR (DMSO-d6): δ 6.92, (s, 1H, D2o exchangeable, =NH), δ 4.1 (s, 2H, CH), δ 1.24 (m, 32H, 16CH2), δ 0.87 (t, 3H, CH3).
MS m/z (%):438 (Ṁ+, 75.24), 323 (57.14), 226 (64.76), 115 (100).
Anal. Calc. (%) for C22H38N4OS2: C, 60.23, H, 8.73, N, 12.77. Found: C, 60.40, H, 8.56, N, 12.87.
2.11. Synthesis of (Z)-5-Benzylidene-3-(5-Heptadecyl-1,3,4 Thiadiazol-2-yl) Imino Thiazolidin-4-One (9)
Equimolar quantities of 8 and benzaldhyde in glacial acetic acid (5 ml) in the presence of anhydrous Sodium acetate (0.8 gm) are heated under reflux for 10 h. after which a precipitate is formed, on cooling, the solid is filtered off, recrystallized from acetic acid to afford Compound 9 was obtained as a pale yellow powder in 75% yield, mp 85˚C - 87˚C.
IR (KBR): υ3426 (=NH), 2919 (CHaliphatic), 1682, (C=O), 1666 (C=N) cm−1. 1H-NMR (DMSO-d6): δ 6.95, (s, 1H, D2o exchangeable, =NH), δ 7.6-7.3, (m, 5H, ArH), δ 1.24 (m, 32H, 16CH2), δ 0.85 (t, 3H, CH3).
MS m/z (%): 526 (Ṁ+, 0.18), 323 (0.29), 115 (100), 89 (0.23).
Anal. Calc. (%) for C29H42N4OS2: C, 66.12, H, 8.01, N, 10.64. Found: C, 66.42, H, 7.90, N, 10.76.
2.12. Antimicrobial Activities
Screening of The antimicrobial activity of the synthesized compounds was evaluated using modified Kirby-Bauer disc diffusion technique [20] [21] [22] [23] , using Mueller-Hinton agar. In 10 ml of fresh media, one hundred micro liters of the test bacteria/fungi were grown until to be approximately 108 cells/ml for bacteria and 105 cells/ml for fungi. Ampicillin and Amphotericin B were used as a standard drugs as a positive control for antibacterial and antifungal activity,
![]()
Table 1. Antimicrobial activity of compounds 1 - 9.
respectively. Filter discs immersed with 10 µl of solvent (distilled water, chloroform, DMSO) and used as a negative control, then a blank paper discs with a diameter of 8.0 mm were impregnated with 10 µl of the tested concentration. (100 µl) was spread onto agar plates that are relevant to the broth in which they are maintained. Standard discs of c (Antibacterial agent), Amphotericin B (Antibacterial agent)were used as positive control for antimicrobial activity, while filter discs impregnated with 10 µl of solvent (distilled water, chloroform, DMSO) were used as a negative control. Blank paper discs (Schleicher and Schuell, Spain) with a diameter of 8.0 mm were impregnated with 10 µl of the tested concentration. The obtained data on the antimicrobial activity of the compounds are shown in Table 1.
2.13. Propoxylation
Using Morgos procedure [24] , 0.01 mol of the synthesized compound was stirred with 0.5 wt% KOH solution and heated to 70˚C slow stream of nitrogen. The nitrogen stream was stopped after flushing out oxygen, then propylene oxide in different moles (3, 5 and 7 mole) was added using a syringe drop-wise with continuous stirring under reflux. The reaction was conducted for different intervals of time (1/2 - 1 h). After cooling, the flask was weighed, and the average degree of propoxylation determined from increment in the mass of the reaction mixture [25] .
3. Surface Active Properties
3.1. Surface and Interfacial Tensions
Measurements of Surface and interfacial tension of 2(a-c) - 9(a-c) were measured by Findlay [26] with a Krüss tensiometer [27] , at different concentrations (0.05 - 10−6 mol/L) of the synthesized surfactant, and at constant temperature (25˚C ± 1˚C) for the interfacial measurements, Paraffin oil and The tensiometer was calibrated using ASTM: D1331-01 method [28] .
3.2. Cloud Point
The cloud point was determined by gradual heating of 1.0 wt% solution in a controlled temperature bath and the temperature at which the clear or nearly clear solution becomes turbid was recorded [29] . It is a measure of the inverse solubility of a nonionic surfactants, the reproducibility of this is checked by clearing the solution again by cooling.
3.3. Wetting Time
Draves test used to measure the Wetting time [30] , of the prepared surfactants by immersing a cotton fabric in a 0.1 wt% aqueous solution of the tested surfactant and measuring The sinking time in seconds.
3.4. Foaming Properties
In a volumetric cylinder, 1.0 wt% solution of the tested surfactant was checked and allowed to fall from a set height initially produced and the height of the foam is measured according the Ross Miles method [31] .
3.5. Emulsion Stability
The emulsion was prepared by stirring (10 ml, 20 mml) of aqueous solution of the tested surfactant and 6 ml of light paraffin oil using magnetic stirrer. After the mixture was vigorously shaken, it was allowed to separate the emulsion and the time taken for about (9 ml) of the aqueous layer separation express the emulsion stability of the surfactant [32] .
3.6. (CMC) Measurements
The critical micelle concentration (CMC) of a surfactant is the concentration at which the solution shows an abrupt change, where the surface active ions in the solution aggregates to form larger units called micelles [33] . Generally, the nonionic surfactants have lower CMC values than their alternative ionic surfactants, and the value obtained as a plot of logarithm of the surfactant concentration versus the surface tension.
3.7. Effectiveness (πCMC)
Decreasing in surface tension induced by a surfactant molecule at the critical micelle concentration is the effectiveness (πCMC) of this surfactants and this can be calculated from difference between surface tension of the pure water (γ0) and the surface tension of the surfactant solution at the critical micelle concentration [34] , (γCMC), Equation (1).
(1)
3.8. Efficiency
The value of negative logarithm of the bulk concentration necessary to reduce surface tension by 20 mN/m is known as efficiency of the surfactant (PC20) [35] , and can be calculated from the following equation, Equation (2).
(2)
where T is absolute temperature in Kelvin and R is the universal gas constant 8.31 × 107 ergs mol−1 K−1.
3.9. Maximum Surface Excess Γmax
Using Gibbs equation (Equation (3)) and values of surface and interfacial tension, the maximum surface excess Γmax can be calculated Equation (3):
(3)
where δγ surface pressure in mN/m, C surfactant concentration and (δγ/δlogC)T is the slope of surface tension versus concentration curves below CMC at constant temperature [36] .
3.10. Minimum Surface Area (Amin)
The average area Amin (Å/mol) occupied by each surfactant molecule and adsorbed at the saturated air/water interface have been calculated easily from Γmax values using the following equation [37] [38] (Equation (4)).
(4)
where N Avogadro’s number 6.023 ´ 1023.
4. Hydrolysis Resistance
Resistance of decomposition of surfactant molecule in aqueous solutions even under extreme PH and temperature conditions was established by surface tension measurements of that surfactant (0.1%) solution in 5% sulfuric acid or 1% sodium hydroxide at ambient temperature.
5. Biodegradability of the Synthesized Surfactants
Die-Away method [39] or River test was used to test the biodegradation of the synthesized nonionic surfactants in River water. In this method samples were drawn daily, filtered and the surface tension was measured using Du-Nouy tensiometer through 7 days and the biodegradation percentage D% was calculated from the following Equation (5).
(5)
where γt surface tension at time t, γ0 surface tension at time zero (initial surface tension). γbt surface tension of the blank experiment at time t.
6. Results and Discussion
Treatment of Stearic acid with Thiosemicarbaziede in phosphorous oxy chloride produce 2-amino-5-heptadecyl-1,3,4-Thiadiazole-2-amine (1) [40] .
Chlorination of the free amino group by chloroacetyl chloride yielded 2-chlorothiadiazolyl acetamide derivative (2) in good yield, which showed υc=o of amide at 1681 cm−1 and its IR spectrum, and mass spectrum showed [Ṁ+] And [Ṁ++2] at (415, 14.3%), and (417, 11.8%) respectively. The chlorothiadiazolyl acetamide (2) reacts with Piperidine in absolute ethanol and anhydrous K2CO3 and furnished N-(5-heptadecyl-1,3,4-Thiadiazol-2-yl)-2-(Piperidin-1-yl) acetamide (3). Its mass spectrum elucidated the structure and indicate the presence of [Ṁ+] at (464, 1.2%), and [Ṁ++2] at (466, 0.16%) and the fragmentation pattern (Figure 1) supported these structures and the mass fragmentation of compound 4 is presented in (Figure 2). Also the mass fragmentation compounds 7.9 are presented in (Figure 3) and (Figure 4) respectively. Heterolytic addition of low molecular weight alcohols to the C=N of thiadiazole has been reported for the photochemical reactions [41] [42] .
By the same manner, piperazine reacts with compound (2) and produced N-(5-heptadecyl-1,3,4-Thiadiazol-2-yl)-2-(piperazin-1-yl) acetamide 4 and its IR exhibits the presence of two υNH’s at 3281,3110 cm−1 while the mass spectra showed [Ṁ+] at (465, 0.9%), [Ṁ++1] at (466, 0.18%)., which supported by the fragmentation pattern of the structure, Figure 2.
Reaction of compound (2) with urea and thiourea in boiling ethanol and in the presence of potassium carbonate yielded Thiadiazolidine-dione (5) and thiadiazolyl imidazole thione (7) respectively and when compound (5) allowed to condense with benzaldehyde in refluxing glacial acetic acid produced (Z)-5-benzyli- dene-3-(5-heptadecyl-1,3,4 Thiadiazol-2-yl) imidazolidine-2,4-dione (6).
On the other hand, when compound (2) refluxed with potassium thiocyanate in dry acetone and in the presence of anhydrous potassium carbonate furnished the thiadiazolyl imithiazolidinone, which upon condensation with benzaldehyde, the (Z)-5-benzylidene-3-(5-heptadecyl-1,3,4 Thiadiazol-2-yl) iminothiadazolidine-4-one (9) obtained.
6.1. Antimicrobial Activities
The antimicrobial activity of the synthesized compounds (1-9) were investigated in vitro (using a modified Kirby-Bauer disc diffusion method against two bacterial strains namely, Escherichia coli (Gram-ve), and Staphylococcus aureus Gram +ve) and two fungal species namely, Aspergillus flavus and Candida albicans With Ampicilline and Amphotericin B as a positive references for antibacterial and antifungal agents, respectively and shown in Table 1. As shown in Table 1, the synthesized compounds showed variable inhibition efficiency against the tested microorganisms. Compounds (3 - 5 and 8) exhibit moderate
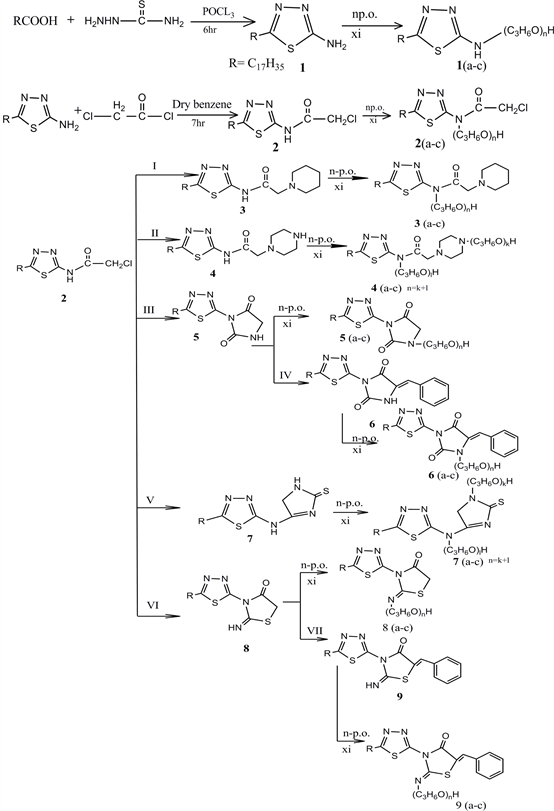
Scheme 1. Synthetic routes of compounds of (1 - 9) and surfactants (1(a-c) - 9(a-c)). (I) piperidine, ethanol, anhydrous K2CO3 reflux 6 hr. (II) piperazine, ethanol, anhydrous K2CO3 reflux 6 hr. (III) Urea, ethanol, anhydrous K2CO3 reflux 10 hr. (IV) benzaldehyde, glacial acetic acid, anhydrous sodium acetate, reflux 10 hr. (V) thiourea, ethanol, anhydrous K2CO3 reflux 10 hr. (VI) Potassium thiocyanate, acetone, anhydrous K2CO3 reflux 2 hr. (VII) benzaldehyde, glacial acetic acid, anhydrous sodium acetate, reflux 10 hr. (XI) n = 3, 5 and 7 mol of propylene oxide (P.o.).
![]()
Figure 1. Mass fragmentation of compound 3.
![]()
Figure 2. Mass fragmentation of compound 4.
![]()
Figure 3. Mass fragmentation of compound 7.
activity towards both tested fungi and bacterial strains. While, compound (7) showed the highest activity towards the tested microorganisms. Which may be possibly due to the presence of the =N-C-S moiety. On the other side, compounds (6 and 9) revealed good activity towards both bacterial and fungal species. These results are in agreement with previously reported results for thiadiazole derivatives [43] [44] [45] [46] .
![]()
Figure 4. Mass fragmentation of compound 9.
6.2. Nonionic Surfactants from the Synthesized Compounds
Addition of propylene oxide in different moles (3, 5 and 7 mol) to the new synthesized compounds (2 - 9) produced the nonionic surfactants 2(a-c) - 9(a-c) which elucidated via their IR and 1H-NMR spectra.
IR spectrum of these compounds showed a broad band in the region (3.500 - 2.500) cm−1 and two other bands in the region of (1100 - 1000) cm−1 and (950 - 900) cm−1, which attributed to (υOH) and (υC-O-C) ether linkage, respectively, in addition to the other bands reported for these compounds. 1H-NMR spectrum showed the propyleneoxy group protons as multiple signals with the chemical shift (3.5 - 3.7) ppm. The physical properties of these compounds are dipped in Table 2.
6.3. Surface Active Properties
The surface active properties of the new propoxylated compounds 2(a-c) to 9(a-c) were evaluated in a neutral medium, and the data obtained are listed in Table 3(a), Table 3(b).
6.4. Surface and Interfacial Tensions
The ability of surfactants to lower the surface and interfacial tension make them available for large number of applications [47] . As their molecules dissociate in water, they weaken the hydrogen bond by orientate themselves in-between the water molecules, which decreases the holding forces and lowers the surface and interfacial tension. The surface and Interfacial Tensions of the new synthesized surfactants increases by increasing the number of propylene oxide units (Table 3(a)), which may be attributed to increase the attractive forces and this is in accordance with the previously reported results [48] .
6.5. Cloud Point
The temperature above which an aqueous solution of surfactants becomes turbid and separates into two phases is the cloud point of surfactant. It is considered effective when used near or below the cloud point, which is helping in determination of the storage stability. The cloud point depends on the chemical structure [49] [50] , and it was reported that it increase with increasing the hydrophilic part [51] [52] , and this is compatible with our results (Table 3(a)).
6.6. Wetting Time
In terms of wetting time, the synthesized nonionic surfactants efficiency was measured according to Draves technique. Shorter the time of surfactant to wet a piece of cotton fibre indicates the more efficiency of the surfactant as wetting agent. As shown in (Table 3), surfactants 2(a-c) to 9(a-c) exhibits various wetting abilities, which in general decreased with increasing the number of moles of propylene oxide, and this may be due to increasing the adhesive forces relatively to the cohesive forces [53] .
![]()
Table 2. Physicochemical properties of the synthesized surfactants.
(a)
(b) ![]()
Table 3. Surface properties of some synthesized surfactants.
a Number of propylene oxide units
6.7. Foaming Power
By lowering the surface tension, the surfactant molecule help in foam simultaneous adsorption of the surfactant molecules onto the interface between gas and liquid interface. Using the Ross Miles method, the foam power of the synthesized nonionic surfactant was measured and the data depicted in Table 3 showed that as the number of moles of propylene oxide increase, the foam height increased, which in agreement with previously reported [53] [54] [55] .
6.8. Emulsion Stability
The ability of the prepared surfactant to form emulsions spread their applications, thus the emulsifying power of these surfactants was measured in term of time needed for 9 ml of the solution is presented in Table 3(a).
The data revealed that, the emulsion stability decreases as the number of propylene oxide units increased, and it is a moderate emulsifying agents.
Generally, the surfactant solubility in the oil phase decreases by increasing the hydrophilic part (head), which weaken the emulsion stability [55] [56] .
6.9. CMC Measurements
CMC is an important feature of a surfactant which measures the efficiency of the prepared surfactants. Before reaching the CMC the surface tension is dramatically changed with the surfactant concentration, and after reaching the CMC, the surface tension remains relatively constant or changes with a lower slope. Surfactants with low CMC values exhibit excellent emulsifying, wetting, solubilizing and detergency properties. As outlined in Table 3(a), Table 3(b), the measured surface tension, CMC values and the surface tension at the critical micelle concentration (γCMC) of compounds 2(a-c) - 9(a-c) increased with the increasing number of moles of propylene oxide incorporated in the structures, which in agreement with the reported results [57] .
6.10. Effectiveness (πCMC)
The ability of a surfactant to induce the maximum reduce in the surface tension is a measure of the effectiveness of a surfactant (πCMC). Since the CMC represents the minimum concentration of the surfactant needed for the maximum reduction in surface tension, thus, the effectiveness (πCMC).
Can be measured from the decrease in the surface tension of the water (γ0), which is induced by this surfactant at CMC. Table 3(b) showed Logically decreasing in the effectiveness of the prepared surfactants s as (γCMC) increased.
6.11. Efficiency (PC20)
In aqueous media, surface active compounds act by lowering the surface tension in between water molecules, so, the surfactant performance can be measured in terms of its adsorption efficiency which is known as surfactant adsorption efficiency and defined as the surfactant concentration required to produce a 20 mN/m reduction in surface tension and denotes as PC20 and can be calculated by Equation (2).
Increasing PC20, increase the adsorption of surfactant at the interface and efficiently the surface tension reduced. Accordingly, the synthesized surfactant PC20 values are found to be decreased by increasing the number of moles of propylene oxide (Table 3(a), Table 3(b)).
6.12. Maximum Surface Excess Γmax
The excess of surfactant per unit area of surface is known as surface excess concentration which express the extent of the surfactant adsorption at the surface of the liquid. Gibbs equation, Equation (3) express the relation between surface tension and Surface excess concentration (Γmax) and to the maximum surface concentration CMC, and efficiency [58] .
(6)
The obtained data from applying Equation (3) are listed in (Table 3(b)). It showed that by increasing the number of propylene oxide units, the maximum surface excess increased and in range of 0.79 and 2.57 mol/cm2.
6.13. Minimum Surface Area (Amin)
The area per surfactant molecule Amin at the interface air/water at the saturated surface gives us information about the packing degree and the adsorbed surfactant orientation. (Table 3(b)) represent the calculated average areas Amin which exhibit a significant decrease in Amin.
Values as the number of propylene oxide units increased in a significant decrease in Amin. This indicates a high packing order upon increasing number of moles of propylene oxide (n).
6.14. Hydrolysis Resistance
Because of tremendous uses of surfactants in manufacture of detergents, the surfactant stability towards acid/base hydrolysis is an important factor in its utilization. The resistance of the synthesized nonionic surfactants towards acidic and alkaline hydrolysis was tested and the data obtained presented in (Table 4).
The data showed that, in acidic medium, compounds 1(a-c) to 9(a-c) show high stability when boiled for 30 min, but they are less stable upon boiling for 60 min. On the other hand, in alkaline medium, they affected slightly even after boiling for 60 min. thus, the synthesized nonionic surfactants would be safe to be used in detergents manufacture.
6.15. Biodegradability
Biodegradation is the destruction a chemical by metabolic activity of microorganisms. Surfactants must be susceptible to biodegradation test in order to examine their safety to environment. The biodegradation of the synthesized surfactants was evaluated by the conventional River Die-Away test [59] and the data listed in (Table 5).
All the synthesized nonionic surfactants seem to degrade easily as the results showed. About 40% - 50% of the surfactants was biodegradable within the first day of the test, and died away through 7 days. Consequently, these surfactants are safe for human beings as well as the environment. In general, the biodegradation of the surfactants decreases by increasing the number of propylene oxide units incorporated in the structure.
![]()
Table 4. Resistance of the synthesized surfactants towards acidic and alkaline hydrolysis.
![]()
Table 5. Biodegradability of the synthesized surfactants.
aNumber of propylene oxide units
7. Conclusions
New thiadiazole derivatives (2 - 9) have been successfully synthesized in good yield. The synthesized compounds (2 - 9) exhibited high activity toward strains of G−, G+ bacteria, while compound (7) showed good antifungal activity. The new nonionic surfactants bearing heterocyclic moieties were synthesized efficiently by incorporation of different moles of propylene oxide.
All the synthesized nonionic surfactants revealed good surface active properties which affected by the hydrophilic part. The lower the number of propylene oxide units, the surface and interfacial synthesized nonionic surfactants tension of the synthesized surfactants is markedly changed by the change of the hydrophilic part. Consequently, the CMC and Γmax, effectiveness (πCMC), emulsion stability, efficiency (PC20) and minimum surface area (Amin) were changed.
Additionally, the new synthesized surfactants exhibit good fastness towards alkaline/acidic media and they are susceptible to degrade within one week. In conclusion, the new synthesized thiadiazole derivatives surfactants are safe for both human beings and the environment. So, it can be recommended as wetting, moderate emulsifiers as well as cosmetics, textiles and dyes manufacture.