1. Introduction
Jatropha curcas L. is a drought resistant perennial plant belonging to family euphorbiaceae. Plants can easily be grown on marginal soils to help reclaim land [1]. Jatropha curcas L. is being cultivated as biodiesel crop in many tropical and sub-tropical areas [2] and can also be seen growing as fence around crop plants in many regions of India. Non-edible oil produced from these seeds is used as feed stock for production of bio-diesel. Press cake is used to improve soil and for the production of biogas. Apart from the oil Jatropha curcas is a significant source of many phytochemicals with varying biological activities [3].
Besides competition weeds have been found responsible for the reduction in quality and quantity of agricultural products through release of some secondary metabolites commonly known as allelochemicals which escape into environment through various processes such as volatilization, root exudation, leaching and decomposition of residue [4] Chemicals thus released exert adverse effects on plants growing in its vicinity. Inhibitory or stimulatory allelopathic interactions among plants significantly influence metabolic processes and productivity of crop plants. Phenolic acids have been identified as most potent compounds which are able to induce inhibitory effects on germination [5] seedling growth and biomass [6]. Inhibition of nutrient uptake and subsequent reduction in accumulated nutrients is one of the mechanisms of action of phenolic compounds [7,8] Supplementation of nutrients not only improves crop productivity but also helps plants to overcome adverse environmental effects such as water stress [9]. Nutrient status of plants has a regulatory role in plant resistance against environmental stresses [10]. Potassium is an important macronutrient and most abundant cation in plant tissues [11].
Application of Jatropha curcas leaf leachate to soil resulted in reduced shoot and root length of marigold (Tagetes erecta L). An increase in relative membrane permeability and proline contents was observed in roots of marigold seedlings. Residue incorporated into soil showed similar effects on growth of marigold plants. This indicates that Jatropha curcas plants release some phytotoxic compounds that are responsible for allelopathic effect [12]. Lower concentrations of extract show stimulation however, inhibitory effects of higher concentrations were more noticeable than stimulatory effects of lower concentrations [13]. Higher concentrations of Jatropha curcas leaf and root extracts have been reported to have strong inhibitory effects on germination, radical and plumule length of some test species such as Phaseolus vulgaris, Zea mays, Lycopersicon lycopersicum and Hibiscus esculentum. These inhibitory effects suggest presence of allelochemicals in leaf and roots of Jatropha curcas that can inhibit crop growth [14].
However, not many reports are available on the allelopathic potential of Jatropha species on crops particularly wheat. Therefore, present investigation focuses on evaluation of its allelopathic potentialon growth of wheat and possible modulation bypotassiun treatments.
2. Material and Methods
2.1. Plant Materials
Leaves and ovary walls of Jatropha curcas were collected from Government Nursery, Agriculture College, Gwalior (affiliated to Rajmata Vijayraje Scindhia Krishi Vishwa Vidhyalaya Gwalior, M P). Seeds of wheat (Triticum aestivum L. cultivar MP-4010) were obtained from Krishi Vigyan Kendra, Rajmata Vijayraje Scindhia Krishi Vishwa Vidhyalaya, Gwalior MP.
2.2. Experimental Procedures
2.2.1. Preparation and Application of Leachates
Leachates were prepared from sun dried leaves and ovary walls of Jatropha curcas. 500 gm of dry powder (prepared using mechanical grinder and subsequently pestle and mortar followed by sieving through 2 mm sieve) of these parts was soaked in water in separate flasks for 48 hours. After 48 hours leachates were filtered through three layers of cheese cloth and final volume is made up to 5.0 L using water. Leaf (LL 1/10, w/v) and ovary wall leachates (OWL 1/10, w/v) so prepared were also used for preparing 1/100 (w/v) concentration by appropriately diluting these solutions.
Pot experiments were conducted during 2009-2010 and 2010-11. Pots of 24” × 12” × 10” in dimensions were used for experiments. Each time pots were filled with well ploughed fresh soil from garden. Leachate (10 ml/ Kg soil) was added to each pot after 15 days of sowing. Irrigation was avoided for 5 days before and after leachate treatment. Wheat plants raised in pots fall in the following four categories:
1—Plants treated with leachates of leaf and ovary walls and grown under normal water supply.
2—Plants treated with leachates of leaf and ovary walls and grown under restricted water supply.
3—Plants treated with leachates of leaf and ovary walls along with potassium treatments (40 kg·h−1) and grown under normal water supply.
4—Plants treated with leachates of leaf and ovary walls along with potassium treatments (40 kg·h−1) and grown under restricted water supply.
2.2.2. Emperical Parameters
After 45 days of leachate treatment leaf area, plant height and length of spike were measured. For determination of leaf area, leaf shape was drawn on a paper and the paper was cut. Subsequently, the paper leaves were weighed and area of the leaves was calculated comparing it with the standard pre-weighed paper of known area following method as outlined by Shahne and Mungikar [15].
Flag leaf and stem were collected from mature plants dried in oven at 60˚C for two days then powdered using pestle and mortar followed by sieving through 2 mm sieve. Fine powder thus obtained was used for analysis.
2.2.3. Total Phenols
Total phenols were estimated following the method of Malik and Singh [16]. 0.5 g powdered plant sample was homogenized in 80% ethanol (ten times volume). Homogenate is centrifuged at 10,000 g for twenty minutes. Supernatant was saved and residue was re-extracted in 80% ethanol (five times volume). Supernatant was evaporated to dryness and dissolved in 5.0 ml distilled water. 0.1 ml of aliquot was taken out and volume is made up to 2.0 ml with distilled water. Folin-Ciocalteau’s reagent (0.5 ml, 1 N) was then added and after three minutes 20% Na2CO3 (2.0 ml) was added followed by keeping in boiling waterbath for one minute. Phenols react with phosphomolybdic acid in Folin-Ciocalteau’s reagent in alkaline medium and produce a blue coloured complex. Absorbance was recorded at 650 nm against blank using spectrophotometer. Concentration of phenols was expressed in mg·g−1 dry weight, equivalent to catechol.
2.2.4. Tannins
Tannins were estimated according to method of Swain and Hills [17]. Powdered plant sample (0.05 g) was taken in test tubes and 7.5 ml distilled water added to it and kept in a boiling water-bath. After 30 minutes it was cooled to room temperature and centrifuged at 2000 g for 20 minutes. Supernatant was collected and final volume made up to 10 ml adding distilled water. 0.1 ml of supernatant was mixed with 1.9 ml of distilled water to make a total volume of 2.0 ml. Folin-Denis reagent (1.0 ml) and 35% Na2CO3 (2.0 ml) was added to it and kept in dark for 45 minutes at room temperature. Absorbance was measured at 700 nm with spectrophotometer. Tannin content of samples was expressed as mg·g−1 dry weight tannic acid equivalents and computation was done using standard curve.
2.2.5. Free Amino Acids
Free amino acids were estimated following the method outlined in Sadasivam and Manickam [18]. Powdered sample (0.5 g) was homogenized in ten times volume of 80% ethanol. Homogenate was centrifuged at 2000 g for 20 minutes and supernatant was collected. Supernatant (0.1 ml)was mixed with distilled water (1.9 ml) to make up 2.0 ml. 1.0 ml of ninhydrin (4% ninhydrin in methyl cellosolve and stannous chloride dissolved in 0.2 M citrate buffer mixed in equal volume) was added to it and kept in boiling water-bath (ninhydrin being a powerful oxidizing agent decarboxylates alpha aminoacids and produces bluish-purple complex ). After 20 minutes 5.0 ml of diluent (Equal volume of n-propanol and distilled water) was added to it and absorbance was recorded at 570 nm with spectrophotometer. Concentration of total free amino acid is expressed in mg·g−1 dry weight as glycine equivalent.
2.2.6. Phytic Acid
Phytic acid was estimated following method of Wilcox et al. [19]. Phytic acid is extracted in 0.4 mM HCl. 100 mg powdered sample was mixed thoroughly with 0.4 mM HCl (1.0 ml) followed by centrifugation at 10,000 g for 20 minutes. 0.1 ml supernatant was taken out and final volume was made 1.0 ml using distilled water. 1.0 ml of colorimetric reagent (3M H2SO4, 2.5% ammonium molybdate and 10% ascorbic acid mixed in equal volume with two volume of distilled water) was added to it and kept at room temperature for one hour. Optical density was measured against blank at 650 nm with spectrophotometer. Concentration of phytic acid was calculated using K2HPO4 as standard and expressed in mg·g−1 dry weight.
2.2.7. Total Free Sugars
Total free sugars were determined using anthrone method [20,21]. Plant sample was boiled in 80% ethyl alcohol followed by centrifugation at 3000 g for five minutes. The residue was again crushed in 80% ethyl alcohol and then centrifuged. The supernatant was pooled together. The alcoholic extract (1 ml) so prepared was evaporated to 0.2 ml in a water bath and the volume was made up to 1 ml with distilled water. 1 ml HCl (1 N) was added and the tubes were kept in boiling water bath for 40 minutes for hydrolysis. Anthrone reagent (4 ml; prepared by dissolving 500 mg anthrone in 720 ml chilled concentrated H2SO4) was then put to 0.5 ml of hydrolyzed extract and the tubes were subsequently kept in boiling water bath for 10 minutes. After taking out from water bath and bringing it to room temperature, optical density was recorded at 620 nm. Computation of total free sugars was done using glucose standards.
2.2.8. Starch
Starch was determined by anthrone method [18]. Residue (pellet) of the sample from which free sugars were extracted was dried in a boiling water bath at boiling temperature, subsequently 2 ml distilled water was added and then kept on a water bath for 15 minutes. Thereafter, cooled and 2 ml 9.2 N perchloric acid was added and stirred for 15 minutes, followed by centrifugation at 3000 g for five minutes. Supernatant was collected in 50 ml volumetric flask. 2 ml of 4.6 N perchloric acid was added again to the residue and stirred for 15 minutes then centrifuged. Finally supernatants were combined and volume made up to 7.5 ml with distilled water. In an aliquot (0.1 ml) 4 ml of anthrone reagent was added. The tubes were then kept in a boiling water bath for 10 minutes and subsequently cooled on ice and brought to room temperature and optical density recorded at 620 nm. Computation was done using standard curve.
2.2.9. Sodium, Potassium and Calcium
The analysis was done using flame photometer as adopted by Tiwari [22]. One gram dried powdered plant material was taken in a conical flask and digested in tri-acid mixture (H2SO4 + HNO3 + HClO4 in 9:3:1 ratio). The colorless digested material was filtered through What man filter paper number 1 into 100 ml volumetric flask making up the total volume to 100 ml. 10 ml aliquot was then taken and made up to 25 ml with distilled water and was read directly on digital flame photometer, employing Na, K and Ca filters separately and percentage of Na, K and Ca was determined using standard curves.
2.2.10. Chloride
Chloride was estimated following Eaton et al. [23]. One gram dry sample was boiled in distilled water (100 ml) on a water bath for 30 minutes. After cooling the extract was filtered and was titrated against 0.1 N AgNO3 solution till a permanent brick red precipitate persists.
2.2.11. Total Nitrogen
Nitrogen estimation was done following micro-Kzeldahl’s method as suggested by Jackson [24] and modified by Iswaran and Marwaha [25]. 1 g soil was taken in a 100 ml Kzeldahl digestion flask and was moistened with 5 ml distilled water followed by the addition of concentrated H2SO4(15 ml) and the mixture was thoroughly shaken. 1N KMnO4 was then added in a small amount till pink colour appeared. Thereafter, catalyst mixture (3 g K2SO4 + 0.3 g FeSO4.5H2O+ 0.15 g CuSO4.5H2O) was added to it. The sample was then digested at low flame for half an hour till the colour of mixture turns from brown to yellowish green. Subsequently, flasks were cooled and contents were transferred to 100 ml volumetric flasks and the volume made up to 100 ml with distilled water and ammonia in the digested solution was then estimated using micro-Kzeldahl’s method. pH and Electrical conductivity of soil were also measured.
2.3. Statistical Analysis
For each parameter, at least four replicates were worked out and standard error determined.
3. Results and Discussion
Treatment of leachates i.e. leaf and ovary wall (1/10, w/v) in pots resulted in slight decrease in plant height and biomass per pot in wheat whereas plants maintained with lower concentrations of leachates exhibited more or less similar growth as in untreated ones. Application of potassium improved growth in all treatments however, maximum improvement in height and biomass was registered in wheat plants which received potassium treatments only and no leachates. Nevertheless, negative impact of higher concentrations of leachates was up to some extent mitigated by potassium application (Table 1).
Decrease in leaf area per plant was registered in plants receiving higher concentrations of leachates of leaf and ovary wall (1/10, w/v). Application of potassium improved leaf area in all treatments but maximum area of leaves has been recorded in plants receiving no leachates but only potassium (Table 2). Nutrient deficiencies have been reported to have additive effects on allelopathy and lower level of soil fertility generally results in more severe allelopathic effects caused by phenolic compounds [8]. Decline in spike length and 100 seed weight of wheat was also observed in leachate treatments i.e., LL (1/10, w/v) and OWL (1/10, w/v). However, treatments of lower concentrations of leachates LL (1/100, w/v) and OWL (1/100, w/v) exhibited more or less similar growth as that of untreated plants (Table 2). Decline in germination, radical elongation, leaf area expansion and dry matter accumulation in cucumber was observed in response to ferulic acid which is many a time implicated in allelopathic interactions [26].
Potassium treatments led to improvement in growth nevertheless, plants receiving higher concentration of leachates along with potassium did not show much enhancement in their biomass. Maximum improvement in all growth parameters was observed in plants which are treated with potassium only and not with leachates (Tables 1 and 2). Potassium induced increase in plant height, leaf area and biomass has been reported by a number of workers [27-29]. Leachate treatment (1/10, w/v) hastened maturity and senescence in wheat plants. Maximum impact on length of plant was noticed after fifteen days of leachate treatment which was probably not so obvious with the passage of time and plants appear to have overcome the adverse effects of allelochemicals present in these leachates, successive irrigation may also play some role in that.
Total phenols in flag leaf and stem of wheat plants treated with leaf and ovary wall leachates (1/10, w/v) slightly increased. Interestingly, substantial increase in total phenols was registered in potassium treatments and increase was more pronounced under water stress conditions (Figures 1 and 2). Potassium has been reported to impart resistance against drought and diseases probably because phenols act as precursor of lignin biosynthesis therefore accumulation of phenols plays a protective role by inhibiting pathogen growth. Application of potassium increases level of phenolic compounds and also improves plant resistance to pathogen growth [30-32]. Phenolic compounds are considered important in plant ecology as they improve plant survival. Environmental stress leads to accumulation of phenols in plants. Phenolic compounds influence competition among plants through al-

Table 1. Plant height (cm) and Biomass (g/pot) of wheat (Triticum aestivum L) grown under normal irrigation (45 days after leachate treatments of Jatropha curcas L).
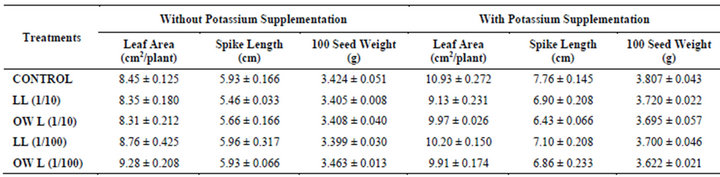
Table 2. Leaf area (cm2/plant), Spike length (cm) and 100 seed weight (g) of wheat (Triticum aestivum L.) grown under normal irrigation (45 days after leachate treatments of Jatropha curcas L.).
Figure 1. Total phenols (mg g−1drwt) in flag leaf of wheat (Triticum aestivum L) grown under normal (N) irrigation and restricted water supply (S) (45 days after leachate treatment of Jatropha curcas L).
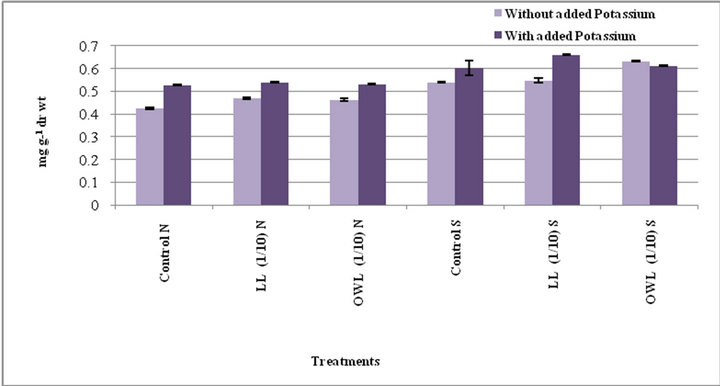
Figure 2. Total phenols (mg g−1drwt) in stem of wheat (Triticum aestivum L) grown under normal (N) irrigation and restricted water supply (S) (45 days after leachate treatment of Jatropha curcas L).
lelopathy [33]. Application of leachates (leaf and ovary wall 1/10, w/v) resulted in increased tannins in wheat plants (flag leaf and stem). An increase in tannin contents as a result of leachate application with added potassium is also obvious. Leaves contain greater tannin contents than stem and the increase was greater under water stress conditions (Figures 3 and 4).
Increase in free amino acids was evident in flag leaf and stem of wheat plants treated with leaf and ovary wall leachates (1/10, w/v). Increase in free amino acid contents was also registered in potassium treated plants (Figures 5 and 6). Potassium induced increase in free amino acids has been reported earlier as well [34]. Amino acids act as osmolytes and are useful in regulating internal water status by bringing about osmotic adjustment. In both the species of Jatropha free amino acids were highest in leaves, possibly indicating it as stress induced consequence contributing to osmotic adjustment. Role of amino acids in increasing relative hydration of protoplasm through osmoregulation has been reported earlier [35]. Increase in level of free amino acids under water stress conditions has also been reported [36]. Treatment of leachates leads to increased free amino acids in wheat plants possibly on account of some kind of stress conditions.
Increased phytic acid contents were evident in flag leaf and stem of wheat plants treated with leachates of leaves and ovary walls (1/10, w/v). Potassium treatments were also observed to enhance phytic acid in the above referred plant parts. Phytate contents do not differ much under normal and water stress conditions in wheat plants treated with leachates. Untreated plants however, showed significant difference in their phytate contents under normal and stress conditions (Figures 7 and 8). Such an increase in the level of these phytochemicals in target plant may be due to their uptake from the medium or synthesis as a result of chemical stress imposed by allelochemicals present in the external medium [37].
Decrease in starch contents was noticed in flag leaf and stem of wheat plants grown in pots treated with leaves and ovary wall leachates (1/10, w/v). However, considerable increase was registered in treatment of these parts with potassium supplementation. Water stress also decreased the starch contents in untreated as well as the plants treated with leachates (1/10, w/v) though positive impact of potassium was evident in plants treated with leachates even under water stress conditions (Figures 9 and 10). Leachate treatments also led to increase in level of free sugars in flag leaf and stem of wheat plants grown in pots. However, increase was more conspicuous when leachate treatments were applied under water stress conditions (Figures 11 and 12). Higher levels of free sugars probably contribute towards maintenance of turgor and osmotic adjustment in seedlings. Accumulation of free sugars under stress conditions is well documented [38- 41]. Potassium induced increase in level of free sugars has also been noticed by others as well [29].
Sodium and potassium contents in leaves of wheat slightly increased with leachate treatments particularly those of leaf (1/10, w/v) and ovary wall (1/10, w/v). Potassium contents were especially greater in potassium treatments because probably potassium supplementation reduced the absorption of sodium. Greater sodium and

Figure 3. Tannins (mg g−1drwt) in flag leaf of wheat (Triticum aestivum L.) grown under normal (N) irrigation and restricted water supply (S) (45 days after leachate treatment of Jatropha curcas L.).

Figure 4. Tannins (mg g−1drwt) in stem of wheat (Triticum aestivum L.) grown under normal (N) irrigation and restricted water supply (S) (45 days after leachate treatment of Jatropha curcas L.).

Figure 5. Free amino acids (mg g−1drwt) in flag leaf of wheat (Triticumaestivum L) grown under normal (N) irrigation and restricted water supply (S) (45 days after leachate treatment of Jatrophacurcas L).

Figure 6. Free amino acids (mg g−1drwt) in stem of wheat (Triticum aestivum L.) grown under normal (N) irrigation and restricted water supply (S) (45 days after leachate treatment of Jatropha curcas L.).

Figure 7. Phytic acid (mg g−1drwt) in flag leaf of wheat (Triticum aestivum L.) grown under normal (N) irrigation and restricted water supply (S) (45 days after leachate treatment of Jatropha curcas L.).

Figure 8. Phytic acid (mg g−1drwt) in stem of wheat (Triticum aestivum L.) grown under normal (N) irrigation and restricted water supply (S) (45 days after leachate treatment of Jatropha curcas L.).
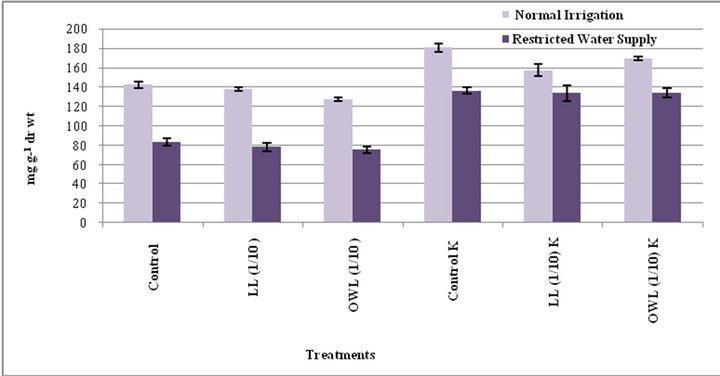
Figure 9. Starch (mg g−1drwt) in flag leaf of wheat(Triticuma estivum L.)45 days after leachate treatment ofJatropha curcas L.
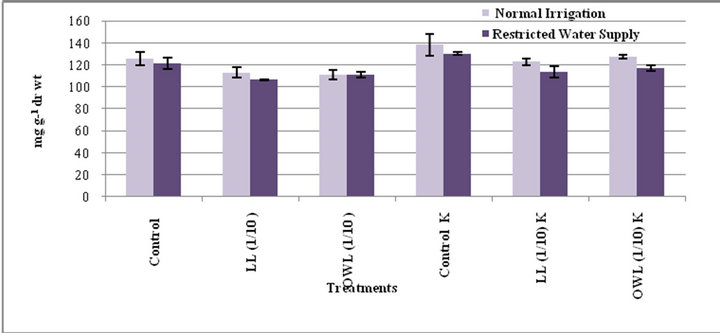
Figure 10. Starch (mg g−1drwt) in stem of wheat(Triticum aestivum L) 45 days after leachate treatment of Jatropha curcas L.

Figure 11. Total free sugars (mg g−1drwt) in flag leaf of wheat (Triticum aestivum L.) 45 days after leachate treatment of Jatropha curcas L.
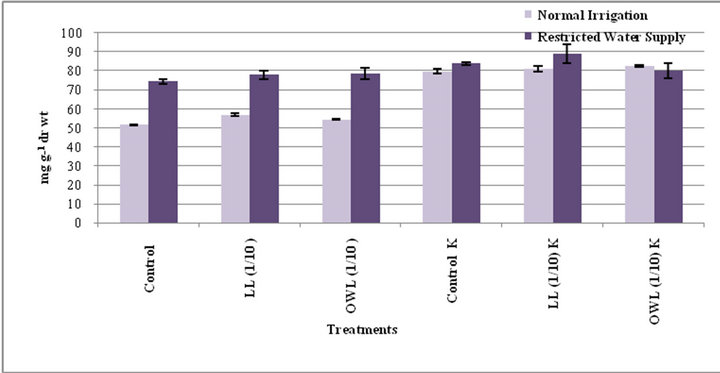
Figure 12. Total free sugars (mg g−1drwt) in stem of wheat (Triticum aestivum L)45 days after leachate treatment of Jatropha curcas L.).
potassium contents were found in soils receiving leachate treatment. In general, it corresponded with the sodium and potassium contents recorded in flag leaves of the plants grown under these treatments (Tables 3-6 and 10). Leachate treatments of leaf and ovary wall of Jatropha curcas resulted in lower Na/K ratio in flag leaf, stem and roots of wheat plants (Tables 3-6). As leaves and ovary walls of both Jatropha spp are rich in sodium (Na) and potassium (K) contents and soil receiving these leachates showed higher Na+/K+ ratio than untreated soil indicating plants selectively preferred absorption of K+ over Na+ (Table 10).
Nitrogen content in flag leaves and stem of wheat are slightly less particularly in treatments of leaf and ovary wall leachates (1/10, w/v) indicating reduced uptake of nitrogen in these treatments whereas, leachate treatments with added potassium showed improvement in nitrogen contents of flag leaf and stem (Tables 7 and 8). Roots receiving leaf and ovary wall leachates (1/10, w/v) show greater contents of nitrogen indicating restricted transport of nitrogen to stem and leaves of wheat plants (Table 9). Soils of leaf and ovary walls of leachate treatment of Jatropha curcas (particularly LL and OWL 1/10, w/v) exhibited less nitrogen contents than soil without leachate treatment indicating its absorption by roots (Table 11).
Calcium contents in flag leaf also registered an increase in leachate treatments particularly in leaf and ovary wall (1/10, w/v) leachates. However, potassium supplementation does not exhibit considerable influence on calcium contents of wheat plants (Tables 7-9). Soil receiving leachates were also found to contain a little more calcium (Table 11). Chloride contents in flag leaf, stem and roots of wheat were greater in plants treated with Jatropha curcas leachates (1/10, w/v) indicating that additional chloride contents may be made available by leachates themselves. Added potassium slightly improved chloride contents in flag leaf, stem and roots of wheat plants. However, increase was more under normal irrigation. Leaves contain highest contents of chloride indicating their efficient absorption and transport. Increase in chloride contents with potassium treatments also indicates its possible entry as counter ion for potassium (Tables 7-9). Soils treated with leachates of Jatropha curcas (particularly 1/10) were also found to contain greater chloride contents as compared with untreated soil (Table 11).
Treatments of leaf and ovary wall leachates (particularly1/10, w/v) resulted in increased contents of sodium in flag leaf, roots and corresponding soils of wheat plants. Increase in absorption and transport of sodium in wheat plants was also observed as a result of leachate treatments both under normal irrigation and restricted water supply. Interestingly, potassium contents also increased in flag leaf, stem, roots and corresponding soils of wheat plants as a result of leaf and ovary wall leachate treatment of Jatropha curcas (Tables 3-6). Greater contents of potassium in flag leaf and roots of wheat plants indicate that potassium is effectively absorbed and transported in plants receiving leachate treatments
Leaves and ovary walls of Jatropha plants are rich in potassium (K) contents which are added to soil as leachates. However, increase in potassium contents in flag leaf and roots of wheat were much more in plants supplemented with potassium. Greater potassium contents are also obvious in corresponding soil of pots. However, leachate treatments also led to increase in potassium contents possibly because of higher contents of potassium in these leachates which led to greater absorption and consequent increase of potassium in plants. Decrease in Na/K ratio as a result of leachate treatment also indicated that absorption and transport of potassium are not affected much by the allelochemicals present in leaf and ovary wall leachates of Jatropha curcas. Na/K ratio further decreases with potassium supplementation reflecting that the absorption of potassium is preferred by wheat plants (Tables 3-6).
Slight increase in pH and electrical conductivity was noticed in soil treated with leachates of leaf and ovary wall of Jatropha curcas. Soil supplied with potassium also showed increased pH and electrical conductivity. However, increase was more conspicuous in soil receiving higher concentrations (1/10, w/v) of leachates (Table 2). Electrical conductivity of soil has been reported to increase as a result of leaf residue of Jatropha curcas incorporated into soil [12]. Chambers and Holm [42] observed reduction in nutrient contents of plants under the influence of allelochemicals. Tannins have been reported to inhibit uptake of potassium and calcium in pigweed seedlings [43]. Interference with nutrient uptake and subsequent reduction in nutrient accumulation is one of the most effective mechanisms of the action of phenolic compounds [8]. Major effects of allelochemicals on nutrient uptake have also been reported [44,45].
Ferulic acid and hydroxybenzoic acid when added to soil resulted into inhibition of phosphorus uptake by plants [46]. Chlorogenic acid when added to soil inhibits the uptake of phosphorus in Amaranthus retroflexus L. However, addition of nutrients NPK to soil nullifies the effect of chrorogenic acid [47]. Nitrogen and phosphorus influence the phytoxicity caused by vanillic and p-oumaric acid [48]. Results of Helianthus annus L debris and chlorogenic acid bioassay suggests that nutrients in debris act differentially in modifying effects of phenolic compounds on seedling growth of Amaranthus retroflexus L than do nutrient added to soil as solution. It suggests
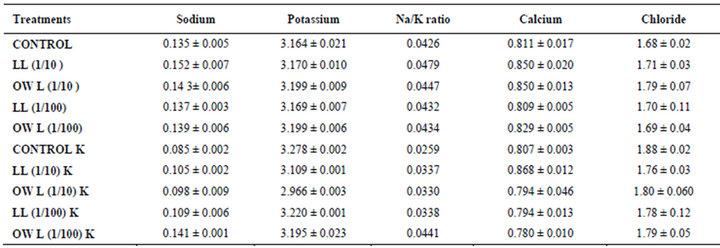
Table 3. Sodium, potassium, calcium and chloride (%) in leaves of (25 days old) wheat (Triticum aestivum L.), 15 days after leachate treatment of Jatropha curcas L.
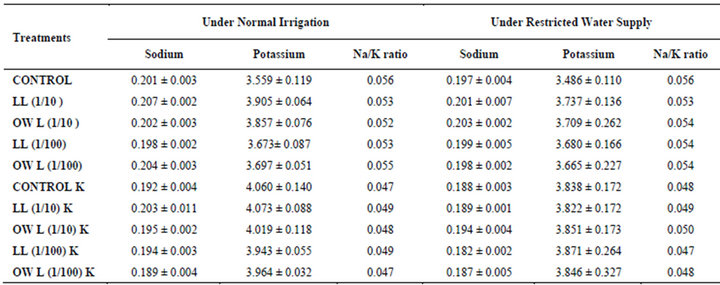
Table 4. Sodium and potassium (%) and Na/K ratio in flag leaf of wheat (Triticum aestivum L.), 45 days after leachate treatment of Jatropha curcas L.
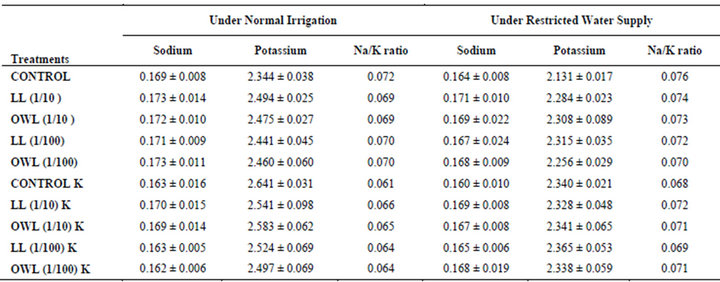
Table 5. Sodium and potassium (%) and Na/K ratio in stem of wheat (Triticum aestivum L.), 45 days after leachate treatment of Jatropha curcas L.
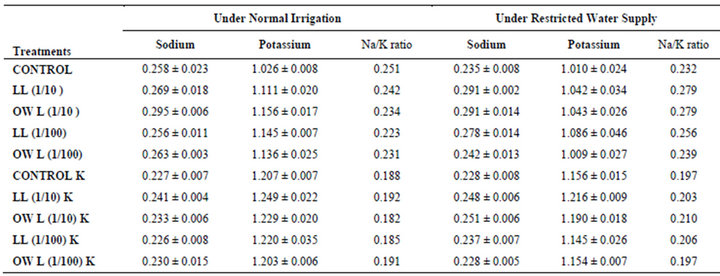
Table 6. Sodium (%) and potassium (%) and Na/K ratio in roots of wheat (Triticum aestivum L.), 45 days after leachate treatment of Jatropha curcas L.
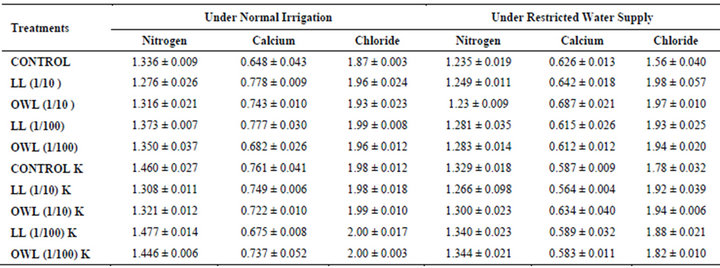
Table 7. Nitrogen, calcium and chloride (%) in flag leaf of wheat (Triticum aestivum L), 45 days after leachate treatment of Jatropha curcas L.
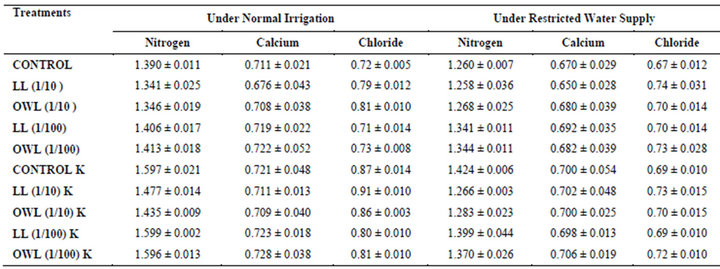
Table 8. Nitrogen, calcium and chloride (%) in stem of wheat (Triticum aestivum L.), 45 days after leachate treatment of Jatropha curcas L.

Table 9. Nitrogen, calcium and chloride (%) in roots of wheat (Triticum aestivum L.), 45 days after leachate treatment of Jatropha curcas L.

Table 10. Sodium and potassium (%) and Na/K ratio in soil in which wheat (Triticum aestivum L.) crop is grown (45 days after leachate treatment of Jatropha curcas L.).
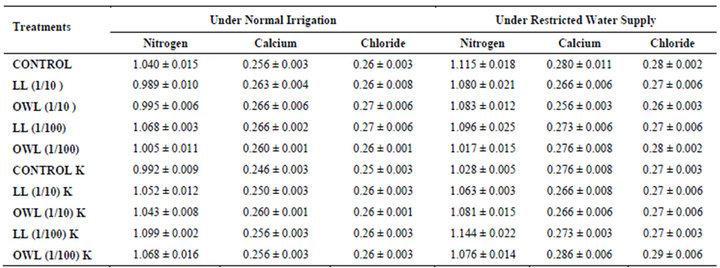
Table 11. Nitrogen, calcium and chloride (%) in soil in which wheat (Triticum aestivum L) crop is grown (45 days after leachate treatment of Jatropha curcas L.).

Table 12. pH and electrical conductivity of soil in which wheat (Triticum aestivum L.) crop is grown (45 days after leachate treatment of Jatropha curcas L.).
that nutrient status of soil may have an impact on allelopathic effects [47]. Salicylic acid treated soybean seedlings showed higher leaf diffusive resistance, lower transpiration and water potential suggesting the interference of allelochemicals with plant water relations as one of the mechanisms that inhibit plant growth. Growth inhibition threshold is influenced by other environmental conditions as well [49].
Jatropha curcas is rich in phenolic compounds [50,51] and flavonoids [52]. Jatropha curcas contains polyphenolic compounds such as flavanols, cinnamic acids, coumarins and caffeic acid. These polyphenolic compounds scavenge free radicals and inhibit peroxidation [53]. HPLC analysis revealed presence of gallic acid, benzoic acid, ellagic acid, quercetin, coumaric acid, benzoic acid and salicylic acid, out of which gallic acid and benzoic acid were predominant [54].
It is evident from the analysis of Jatropha spp that leaves and ovary walls are rich in phenolic compounds and phytic acid etc. and treatments of higher concentrations (1/10, w/v) of leaf and ovary wall leachates lead to stressing imposed by allelochemicals present in the leachates of these parts. Enhancement in total phenols and free amino acids was also conspicuous under water stress and potassium application. Hence, the analysis reveals that treatments of leachates of leaves and ovary wall mimic many consequences which are evident as a result of water stress imposition and potassium application.
Plants differ in their sensitivity to allelochemicals as pearl millet, sesame and cluster bean differ in their response to leaf leachates of Tephrosia purpurea and seasame being more sensitive than pearl millet and cluster bean [55]. Aqueous extracts of Jatropha curcas inhibited the seedling growth of Capsicum annum and degree of inhibition increases with increasing concentrations of extract. Contrary to it the same extract improves the growth of Sesamum indicum and stimulatory effects also increases with the increasing concentrations of extracts up to 20% [56].
Leaf and root extracts of Jatropha curcas inhibited the growth of corn (Zea mays) and tobacco (Nicotiana tabacum) and degree of inhibition increased with increasing concentration of extracts. GC-MS analysis indicated presence of azelaic acid in Jatropha curcas, possibly providing a competitive advantage to Jatropha curcas by inhibiting growth of neighbouring plants [57].
Jatropha curcas biomass when applied as green manure in rice fields improved the crop yield [58]. Press cake of Jatropha curcas being rich in nitrogen serves as source of nutrients to plants and is used as fertilizer [59]. Jatropha curcas planted on boundries of wheat fields do not show any ill effects on crop yield. Moreover, it supports healthy growth of natural vegetation [60]. Our results and observations suggest that effects of Jatropha curcas on wheat are slightly inhibitory however, these effects do not last for a long.
We also observed during the two seasons of our experimentation growth of other plants such as Eclipta alba, Phylanthus niruri, Calotropis procera, Peristrophe bicalyculata, Tephrosia perpuria, Commalina bengalensis, Martynia annua, Corchorus species, Boerhavvia diffusa, Belanites aegyptica, Croton bonplandianum, Digera muricata and Parthenium hysterophorus growing in the vicinity of Jatropha plantation do not appear to be significantly affected. Though influence of Jatropha plants is found to be limited nevertheless, care must be taken to select the location for Jatropha curcas plantation, as continued plantation may lead to accumulation of such constituents and such a situation indicates need for further analysis and experimentation.
4. Acknowledgements
Thanks are due to Prof Rekha Bhadauria, Head, School of Studies in Botany for facilities and Prof G B K S Prasad, Head, School of Studies in Biochemistry, Jiwaji University, Gwalior, for useful interactions. Thanks are also due to MPCST, Bhopal for funding a project.