Subsurface Geotechnical-Characterization near Some Buildings at the Centro Universitario de la Costa UDG (Puerto Vallarta, Jalisco, Mexico) Using Electrical Resistivity Tomography ()
1. Introduction
In civil engineering, geotechnical studies are crucial for assessing subsurface composition, strata continuity, and resistance to loads, ensuring safe substructure and superstructure design. Typically, in-situ, studies involve costly boreholes, especially when multiple are required. Non-invasive geophysical methods have become popular alternatives, providing insights into subsurface characteristics, groundwater presence, cavities, fractures, material changes, and geological risks. These methods help prevent structural issues and facilitate effective risk mitigation, contributing to the success of civil engineering projects.
One of the most developed methods in shallow geophysical exploration is Electrical Resistivity Tomography (ERT). This technique has advanced significantly due to improvements in technology, enhancing how measurements are taken, processed, and interpreted. ERT is versatile, providing detailed and accurate subsurface images from relatively simple and quick field implementations. Some examples of ERT applied to geotechnical subsurface characterization [1] [2], archaeological prospecting [3], mineral exploration [4], flow path in geothermal areas [5], pollution and vulnerability of aquifers [6].
In geotechnics, ERT’s primary application is studying subsurface strata continuity for civil engineering projects. For instance, some central Mexican states, such as Aguascalientes, mandate geological-geophysical studies to detect potential geological discontinuities for construction permits [7]. ERT also assesses the current subsurface conditions under existing buildings that have sustained structural damage, possibly linked to changes in subsurface conditions. For critical structures without evident damage, ERT studies help determine current subsurface conditions, allowing for the identification of anomalies and the development of mitigation strategies to ensure the integrity of these civil edifications.
In the particular case of the state of Jalisco, several significant cities are situated in regions of sedimentary fill and currently exhibit a high demand for groundwater resources. This demand fosters conditions for land subsidence, potentially causing surface fissures that represent subsurface geological discontinuities. Furthermore, changes in the groundwater level could alter the degree of subsurface saturation, consequently modifying its mechanical properties. Recently, in Puerto Vallarta, geophysical methods have begun to be implemented in subsurface geotechnical characterization.
Reference [8] identified significant risks of saline intrusion into freshwater aquifers due to high groundwater demand and proximity to the sea. Utilizing ERT, the research reveals low-resistivity (7 to 54 Ω‧m) areas indicative of saline water, particularly near the Pitillal River basin. Chloride presence in certain wells signals saline water infiltration, threatening water quality and posing health risks. The study recommends sustainable groundwater management, including monitoring extraction rates and ensuring aquifer recharge, to mitigate these risks and protect freshwater resources.
Reference [9] estimated the liquefaction potential in the metropolitan area by analyzing 91 shear wave velocity profiles (V_S30) and SPT tests, considering three earthquake scenarios with magnitudes of 7.5, 8, and 8.1. Two MASW surveys were conducted near Centro Universitario de la Costa (CUCosta): the first to the East in the Pacífico Azul (~0.3 km) residential development and the second to the South on Avenida México (~0.6 km). The first survey showed a velocity of ~290 m/s from 0 to 2 meters in depth, with a groundwater level causing a decrease to ~225 m/s, and further decreasing to ~140 m/s at greater depths. The second survey indicated a velocity of ~320 m/s from 0 to 2 meters in depth, with groundwater reducing it to ~235 m/s, increasing to ~390 m/s at 8 meters, and then decreasing between 10 to 25 meters in depth.
Reference [10] classified into three soil types: C (very dense soil and soft rock), D (stiff soil), and E (soil with more than 3 meters of soft clay) the subsurface at Puerto Vallarta based on V_S30 values and the National Earthquake Hazard Reduction Program. In addition, a geotechnical microzonation was proposed based on soil period: Zone 1 (Ts < 0.4 s), Zone 2 (0.4 < Ts < 0.8 s), and Zone 3 (Ts > 0.8 s). The central area predominantly has type E (Zone 3) and D (Zone 2 and 3) soils, while the southern region near the mountains has type C (Zone 1) soil. Particularly, the CUCosta is located within the soil type D (Zone 3). The study considers four main building typologies and emphasizes the high vulnerability of confined masonry and moment-resisting frame structures to seismic resonance. Recommendations include avoiding tall buildings in very soft soil areas and implementing comprehensive building instrumentation for better seismic assessments.
Within the CUCosta facilities (vehicle parking lot in front of the graduate building), a pavement design was carried out based on the results of invasive soil mechanics tests, specifically standard penetration tests [11]. The soil stratigraphy was generally characterized by the presence of silts and sands from the surface down to 1.5 m depth, a saturated silt horizon was identified, with little clay content, and no groundwater level was found. Based on the previous results and to achieve a more comprehensive geotechnical characterization of the subsurface, ERT profiles were meticulously designed and executed around key buildings within CUCosta (including the Workshops, Auditorium, and Rectory). These profiles were aimed to delineate subsurface conditions at greater depths, providing critical insights into the soil stratigraphy and potential geotechnical challenges. This advanced characterization is essential for ensuring the stability and safety of these structures.
2. Materials and Methods
2.1. Study Area
Puerto Vallarta is a city and port located on the Mexican Pacific coast, in the state of Jalisco, approximately 300 km west of Guadalajara, the capital of Jalisco. Geographically, Puerto Vallarta is located at latitude 20˚37'19" North and longitude 105˚13'52" West. The population of Puerto Vallarta is approximately 291,839 inhabitants [12]. The city has experienced steady growth over the past decades due to its popularity as a tourist destination, which in turn has increased the demand for services and infrastructure. The main economic activities include tourism, tourism-related services, commerce, fishing, and agriculture [13].
The Puerto Vallarta region is characterized by its geological diversity and numerous surface water currents, which have resulted in the formation of diverse soils. In addition, the active tectonic environment emphasizes the necessity of a detailed characterization of the subsoil to identify soil layers, their continuity, and the presence of water. These factors are essential to ensure the stability of both newly designed and constructed buildings and existing structures.
2.2. Tectonic and Geological Setting
The Puerto Vallarta region is in western Mexico and represents an area with a complex tectonic configuration due to the convergence of the North American, Cocos, and Rivera tectonic plates (Figure 1). This convergence causes deformation and fragmentation of the continental plate, forming a tectonic unit known as the Jalisco Block. This unit is bordered by the Tepic-Zacoalco, Colima, and Chapala continental rift zones, which are regions of crustal weakness that have led to the area’s volcanism [14]. Seismic activity in the region is not as intense as in the southern part of the country. However, during the 20th century, there were 12 earthquakes with magnitudes greater than 6.5, including events with magnitudes of 8.2 in 1932 and the most recent one in 1995 with a magnitude of 8 [15].
Near the urban area of Puerto Vallarta, the following lithostratigraphic units are distinguished: Granites, volcano-sedimentary groups, fluvial-deltaic conglomerates, alluvial deposits, and beach sediments (Figure 2). According to [15], the intrusive igneous rocks of granitic composition are identified to the south and southeast of the region and are associated with the Puerto Vallarta batholith. To the northeast and east, a volcano-sedimentary sequence composed of andesites, tuffs, and volcanic conglomerates outcrops, deformed by the influence of faults. In the coastal plain, extending to the foothills of the mountains, there is an outcrop of poorly consolidated sediment sequences consisting of conglomeratic sands, sands of various grain sizes, and silts, presumably deposited by a high-energy fluvial-deltaic system.
The main watercourses in the region are responsible for eroding and depositing sediments in riverbeds and floodplains (Figure 2). The Ameca River is the largest in the area and has created a wide floodplain that has expanded due to the confluence with the Mascota River, whose channel has occupied sectors of Ixtapa, including CUCosta. The fluvial deposits have a very varied composition, including sands composed of white quartz, crystalline quartz, and rock fragments with grain sizes ranging from silts and fine sands to cobbles over 1 meter in diameter [15].
![]()
Figure 1. Location map of Puerto Vallarta showing its spatial relation to the surrounding tectonic domains (North America, Cocos, Rivera, and Pacific Plates) and plate boundaries. Filled black triangles showed the principal volcanoes of the Trans-Mexican Volcanic Belt. Rift zones: Tepic-Zacoalco (TZR), Colima (ColR), and Chapala (ChaR) also are indicated. Gray solid-lines show the tectonic-fault systems. Star symbols show the largest regional cities: Guadalajara (GDL), Tepic (TEP), and Colima (COL). Inset: Location map of the study area within the American continent.
Figure 2. Location map of Puerto Vallarta showing its spatial relation to the surrounding geology units, topography, watercourses, and bathymetry. The star symbol indicates the location of CUCosta.
The described geological units create characteristic relief with similar topoforms and land features (Figure 2). Specifically, in the vicinity of CUCosta, the influence of geoforms associated with marine terraces, coastal plains, floodplains, and basins is distinguished. The marine terrace forms a west-northwest inclined plateau, bordered to the north and west by the floodplain and coastal plain via a topographic drop of between 12 and 15 meters. The floodplains host numerous shifts in the positions of the main watercourses, such as the Mascota River floodplain adjacent to CUCosta, which is over 4 km wide [15].
2.3. ERT Design, Implementation, and Data Processing
Within the CUCosta, ERT-2D profiles were conducted on the Rectory, Auditorium, and Workshop Buildings due to their significance and high occupancy levels. These structures likely did not undergo ERT studies during their construction. To identify vertical subsurface variations, the Dipole-Dipole array was used, known for its sensitivity to horizontal changes in electrical resistivity. This method ensures a detailed assessment of the subsurface characteristics, enhancing the understanding of potential geotechnical issues and contributing to the safety and stability of these critical facilities.
For this study, two Dipole-Dipole arrays were designed and implemented on the target buildings. The first array had a 1-meter electrode spacing (a = 1), while the second array used a 3-meter electrode spacing (a = 3). For both arrays, we conducted seven investigation levels (n = 7). The theoretical exploration depth (ted) were calculated as 2.8 meters and 8.4 meters, respectively, using Equation (1). The equipment used is a subsurface electrical resistivity meter, model Gito-1100 V, manufactured by Hematec.
(1)
On February 21, 2024, near the Workshops Building, an ERT profile using a Dipole-Dipole array with a 1-meter electrode separation was conducted, covering 48 meters with an NW-SE orientation (Figure 3). On March 13, 2024, near the Institutional Auditorium, a 58-meter Dipole-Dipole ERT profile with a 3-meter separation and the same orientation was carried out (Figure 3). Around the Rectory building, two Dipole-Dipole ERT profiles were performed on March 14, 2024. The first, with a 3-meter separation, spanned 41 meters in a NE-SW direction, while the second covered 28 meters in an NW-SE direction (Figure 3).
The apparent electrical resistivity values measured along each ERT profile were manually recorded. These values were then digitized into Excel files. Basic descriptive statistics (mean, median, mode, standard deviation) were calculated for each exploration level to identify and remove anomalous values before the modeling and inversion process. The inversion of geophysical data was performed using the Res2DInv software (x64 ver.4.810) from Geotomo Software. The software employs the least-squares method based on a quasi-Newton optimization technique [16] [17]. To ensure data quality, field data was assessed using RES2DINV’s edit tool to identify and remove outliers, such as high and low resistivity values, which can skew analysis and affect the generated apparent resistivity pseudosections. The outliers removed were selected based on our statistical analysis conducted. During inversion, RES2DINV generates appropriate 2D apparent resistivity pseudosections for each survey, using finite-difference modeling and a nonlinear smoothness-constrained least-squares optimization technique to calculate the model block resistivities. Specifically, for this study, we considered the model refinement option with blocks of the size of half the electrode spacing and extended the model’s boundaries to reduce edge effects in the inversion.
![]()
Figure 3. Location of the ERT profiles within CUCosta. (a) Workshops Building. (b) Auditorium and Rectory. The starting point, endpoint, and acquisition direction are indicated by the arrow symbol. Additionally, the length of each profile is shown.
3. Results and Discussions
The ERT profile at the Workshops Building (Figure 3(a) comprised 280 data points with apparent resistivity values ranging from 2.10 Ω‧m to 264.90 Ω‧m, with an average of 33.96 Ω‧m. Basic descriptive statistics were calculated for each exploration level (Table 1), and the graphical representation of resistivity values across levels is shown in Figure 4.
Table 1. Descriptive statistics for each exploration level of the ERT profile corresponding to the Workshops Building. Values are in units of Ohm-meters.
Level |
Minimum |
Maximum |
Range |
Mean |
Standard Deviation |
N = 1 |
2.10 |
183.30 |
181.20 |
54.89 |
45.13 |
N = 2 |
7.70 |
134.30 |
126.60 |
54.32 |
34.62 |
N = 3 |
10.70 |
143.00 |
132.30 |
46.02 |
25.03 |
N = 4 |
17.30 |
194.60 |
177.30 |
44.39 |
29.93 |
N = 5 |
7.90 |
153.80 |
145.90 |
37.07 |
23.78 |
N = 6 |
5.30 |
264.90 |
259.60 |
35.34 |
40.25 |
N = 7 |
6.30 |
88.00 |
81.80 |
27.20 |
18.65 |
Based on the results indicated in Figure 4, 19 data points were removed. The corrected ERT profile consists of 261 resistivity values. The maximum, minimum, and average values are 194.60 Ω‧m, 6.30 Ω‧m, and 35.24 Ω‧m, respectively. Figure 5 presents the inversion results of the ERT profile data collected near the Workshops Building. After 5 iterations, the RMS error was reduced to 16.20%.
Figure 4. Apparent electrical resistivity behavior measured for each exploration level in the ERT profile of the Workshops Building (black line). The average value is indicated by a dashed gray line, plus or minus one standard deviation is illustrated by a dotted gray line.
The ERT conducted near the institutional Auditorium, consisted of 112 data points with apparent resistivity values, ranging from a minimum of 5.50 Ω‧m to a maximum of 952.10 Ω‧m, with an average value of 86.88 Ω‧m. Descriptive statistics identified 29 outlier resistivity values. After removing these outliers, the remaining 83 data points had a recalculated average resistivity of 70.48 Ω‧m, with the maximum and minimum values adjusted to 949.50 Ω‧m and 5.50 Ω‧m, respectively. The inversion results of the corrected ERT profile data showed a reduction in RMS error to 34.20% (6 iterations) in comparison with the inversion of the original data (RMS = 61.20%).
ERT profile-1 in the Rectory building (Figure 3(b)) consisted of 70 points with apparent resistivity values, the maximum value was 137.60 Ω‧m and the minimum was 5.90 Ω‧m; while the average value was 22.16 Ω‧m. After removing 7 outlier values based on descriptive statistics, the inversion results were achieved after 7 iterations, resulting in a final RMS error of 16.20%. Rectory ERT corrected profile-2 (Figure 3(b)) consisted of 38 apparent resistivity values, with an average of 12.70 Ω‧m and a range between 5.00 Ω‧m and 35.10 Ω‧m. The resistivity inversion model was obtained after 7 iterations (RMS = 15.60%).
Figure 5. 2D Electrical Resistivity Tomography Model of Workshops Building. (a) Observed apparent resistivity pseudosection; (b) Calculated apparent resistivity pseudosection; (c) Real resistivity model. The horizontal axis is the length of the ERT profile in meters. The vertical axis is the pseudo-depth for (a, and b) and depth for (c), in meters.
To interpret the ERT results within the same geological and geotechnical context, a unified color scale with 16 divisions (ranging from 1 to 1000 Ω‧m) was employed. This standardization ensures that geoelectric units present in the ERT profiles can be consistently established and identified. In this context, five geoelectric units (Table 2) were defined based on the results of the soil mechanics study [11], the shear wave velocity profiles [9] [10], and the theoretical values and corresponding range of variation of electrical resistivity for different geological materials.
Table 2. Geoelectrical units, resistivity variation range, and geological materials associated. Values are in units of Ohm-meters.
Geoelectrical Unit |
Resistivity Variation Range |
Color Scale |
Geological Material |
UG-1 |
1 - 20 |
|
Silt, sands, and clays with a high
degree of saturation |
UG-2 |
20 - 40 |
|
Wet silts, sands, and clays |
UG-3 |
40 - 100 |
|
Dry silts, sands, and clays |
UG-4 |
100 - 500 |
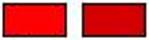
|
Sands and gravels in a silty to clayey matrix in a dry condition |
UG-5 |
750 - 1000 |
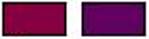
|
Sands and gravels in a lightly
cemented matrix or consolidated borrow fill material |
In general terms, these geoelectric units correspond to the same subsurface sequence composed mainly of silts and sands, with minimal clay and gravel content. The variation in electrical resistivity within these materials is primarily influenced by water content (humidity or saturation). Additionally, there may be slightly cemented materials or consolidated fill material constituting the final geoelectric unit.
The ERT profile near the Workshops Building (Figure 6(a)) reveals that the subsurface, up to 2 meters deep, consists of moist silts and sands (UG 2) and dry sands (UG-3). Moist areas are likely due to irrigation water percolating through tree root zones. A resistive anomaly (UG-4) detected between 32 and 44 meters from the surface to a depth of ~1.50 meters may suggest the building’s foundation, though detailed substructure information is unavailable, and no other similar anomalies were found.
A possible explanation for the high-resistivity zone near the Workshops Building and CUCosta’s boundary is that it relates to fill material used for ground stabilization. This area contrasts with a conductive anomaly (UG-1) at the profile’s end, which could indicate water-saturated fill or loose, saturated material. Notably, heavy rainfall from a recent cold front caused persistent waterlogging near CUCosta’s boundaries. This area’s frequent moisture and infiltration issues are evidenced by persistent signs of dampness and seepage through the boundary wall, with the residential development slightly elevated compared to CUCosta.
Shear wave velocity profiles interpreted by [9] in the Pacífico Azul residential development, about 300 meters east of CUCosta, identified the water table at a 2-meter depth as the cause of decreased V_S30 wave velocity. This is consistent with the geoelectric unit UG-1, characterized by high saturation and conductive properties, found along the entire ERT profile at the Workshops Building, with depths from 1.25 to 2.25 meters. The northwest section is linked to a nearby canal, while the southeast may be due to infiltration from the neighboring residential development. UG-1 might also contribute to increased moisture (UG-2) through capillary action.
Figure 6. Interpreted ERT profiles conducted: (a) Workshops Buildings, (b) Auditorium, and Rectory, profile-1 (c) and profile-2 (d).
The ERT profile near the Auditorium Building (Figure 6(b)) reveals that up to a depth of approximately 3 meters, the subsurface comprises lateral successions of saturated silts and sands (UG-1), moist (UG-2), and dry (UG-3). From 3 to 6 meters, the subsurface features relatively consolidated units (UG-4 and UG-5) with an almost horizontal arrangement.
The ERT profile shows surface-level UG-1 consistency with tree locations, which retain water and moisture when irrigated. Between 5 and 15 meters, UG-1 is associated with water accumulation around a pedestrian walkway, transitioning into a natural channel. Moist zones (UG-2) also align with tree locations. Dry material (UG-3) predominates on the surface, while slightly conductive anomalies (UG-2 and UG-1) represent the same material with varying moisture levels. Additionally, superficial resistive anomalies (~100 Ω‧m) correlate well with recorded electrical and sanitary infrastructure during resistivity data acquisition.
It is feasible to associate the resistive units (UG-4 and UG-5) with consolidated and possibly cemented material, which represents the soil on which civil buildings can be constructed. Specifically, UG-4 extends almost throughout the entire ERT profile, with a depth varying between 3 and 5 meters, and is exposed at the profile boundaries. However, its presence in these regions may correspond to a mathematical artifact generated by the inversion model, indicating the need for similar studies specifically in these areas. The most resistive unit (UG-5) is associated with a cemented subsurface starting from a depth of 5 meters. These units are consistent with the depth at which an increase in shear wave velocity was observed as in [9], which can be physically associated with denser or cemented material. Additionally, due to the building characteristics, the presence of platforms with borrowed fill material that provides the required load-bearing capacity for the institutional Auditorium cannot be ruled out. Finally, it is noteworthy that the electrical resistivity measurements do not show values that could be associated with a shallow water table in the vicinity of the studied building.
Figure 6(c) illustrates ERT profile-1 from the eastern side of the Rectory Building. The shallow sector (up to 1 meter deep) displayed high resistivity values (UG-4) between 10 and 20 meters, likely due to concrete structures near a fountain. Despite the profile being laid 30 centimeters from the structure, the method detected this poorly conductive material due to its 3D sensitivity. The middle to the end of the profile identified moist to dry materials (UG-2 and UG-3) in the shallow zone, clearly defining a surface channel (~38 meters in the profile, UG-1) that drains towards a nearby artificial lake.
In this context, the dominant geoelectric unit in this ERT profile, UG-1, is strongly influenced by the nearby artificial lake, located just 10 meters to the east. This lake receives treated water rich in nutrients and chlorides, which results in high electrical conductivity. UG-1, found between 1.50 and 4.50 meters deep, consists of poorly consolidated silts and sands highly saturated with this electrolyte-rich water, indicating a shallow local water table. This is consistent with findings near the Workshops Building but not with the Auditorium. The discrepancy is due to a natural channel near the Workshops that directs treated municipal water toward the artificial lake near the Rectory, while the Auditorium remains unaffected by this flow.
Below the low-resistivity (high conductivity) unit, UG-4 was identified. This unit is consistent with consolidated and possibly cemented material suitable for civil building foundations. It is also responsible for the increase in shear wave velocity identified by [9] starting at a depth of 5 meters.
Figure 6(d) shows the interpretation of ERT profile-2 conducted south of the Rectory building. The shallow sector up to 1.50 meters deep consists of alternating silts and sands with varying saturation (UG-1 and UG-3) and moisture levels (UG-2). From 1.50 to 6 meters, UG-1 is consistent with findings from profile-1 at the Rectory, indicating highly saturated material due to lateral infiltration from the nutrient and chloride-rich artificial lake, reducing electrical resistivity. Below this layer lies UG-4, a practically impermeable, resistive unit of consolidated, likely cemented material, suitable for civil construction.
4. Conclusion
The ERT profiles conducted near the buildings of interest allowed for detailed subsurface characterization from a geotechnical perspective. The method effectively detected changes in moisture and saturation levels in the subsurface. The measured and modeled electrical resistivity values matched the subsurface composition (sands and silts) identified in previous invasive studies. However, the primary source of uncertainty was at the profile boundaries, which showed significant resistivity contrasts not reflected in the apparent resistivity pseudosections. These anomalies might be mathematical artifacts from the inversion process aimed at reducing RMS error. If these discontinuities are real, they indicate regions with different geotechnical properties, potentially posing structural risks. Therefore, additional complementary studies are needed to better characterize these profile boundaries and other areas of the CUCosta campus. In this context, the results obtained are crucial for the planning and construction of future buildings within the CUCosta campus. These findings serve as a reference framework for the decision-making authorities responsible for projecting infrastructure within CUCosta. It is intended that, in time, this technique will be commonly implemented in the region as a non-invasive alternative to successfully characterize the subsurface from a geotechnical and civil engineering perspective.
Acknowledgements
We would like to express our gratitude to the University of Guadalajara for providing the technical and infrastructural support that greatly facilitated this research. In addition, the APC was funded by the Guadalajara University, Project 275173 (Programa de Difusión de los Resultados de Investigación del Centro Universitario de la Costa de la Universidad de Guadalajara, Proyecto 275173). The authors gratefully acknowledge to the geophysical data acquisition brigades made up by students performing their social service: Diana Arrizón, Daniela Ayala, Karen Ramírez, Erik Tapia, Vanessa González, Luis García, Daniela Anaya and Itzel Rivas. Thanks are extended to the reviewers for useful comments that improved the manuscript.