The Sources of Extracellular Adenosine in Hippocampus and Neostriatum of the Rat Brain ()
1. Introduction
Adenosine is released metabolically from active brain cells and is generated extracellular by degradation of adenosine triphosphate (ATP) [1] , and to provide a scientific data for present research. It needs to explain how adenosine was released from sources in the striatum. Adenosine has long been recognised as an important neuromodulator with profound effects on activity in the central nervous system (CNS). The inhibitory effect on synaptic transmission in hippocampus and neostriatum is mediated by A1 adenosine receptors [2] , which are located both preand postsynaptically. Adenosine acts postsynaptically to induce or to increase potassium conductance’s [3] [4] and presynaptically to decrease the release of neurotransmitters such as glutamate [5] [6] . Adenosine is a normal constituent of all body fluids, including the extracellular fluid in the CNS, where it can regulate nervous activity. It has been proposed to be a sleep propensity factor [7] Adenosine levels are drastically elevated during hypoxia or ischemia [8] for further reference see [9] metabolic stress and seizures [10] , with the consequence of profound inhibition of neuronal excitability and synaptic transmission. Intraand extracellular adenosine concentrations are kept in equilibrium by means of bidirectional nucleoside transporters. Some of these carrier mechanisms can be inhibited by drugs such as dipyridamole, nitrobenzylthioinosine and dilazep [11] [12] . The direction of adenosine transport depends on its concentration gradient between the cytosol and the extracellular space.
The intracellular level of adenosine is regulated by several enzymes. Adenosine kinase phosphorylates intracellular adenosine and keeps the intracellular concentration low. The basal extracellular levels of adenine nucleotides are low. Adenine nucleotide is released in the extracellular space and rapidly hydrolysed by ecto-nucleotidases to form adenosine. The nucleotide catabolizing enzyme ecto-5'-nucleotidase is abundant in the central nervous system [13] -[15] . The relevance of adenosine kinase, adenosine deaminase and SAHH (S-Adenosylhomocysteine hydrolase) activity for adenosine levels has been investigated previously [16] -[18] .
2. Methods
We have now examined the relative importance of the two sources of extracellular adenosine in neural tissue: extracellular adenine nucleotides and adenosine released from the intracellular space. We have determined the effect of ectonucleotidase and adenosine kinase on field potentials from hippocampal and neostriatal slices, in order to estimate the relative contributions of adenosine direct release and nucleotides as sources of extracellular adenosine.
We have used a, b-methylene adenosine 5'-diphosphate (AOPCP), a potent inhibitor of ecto-5'-nucleotidase, 1, 3-dipropyl-8-cyclopentylxanthine (DPCPX), a selective A1-receptor antagonist, and iodotubercidin (Itu), a potent and selective inhibitor of adenosine kinase to study this question electrophysiologically in hippocampal and striatal slices.
Young adult Wistar rats were decapitated, and the brains were rapidly removed and placed in ice-cold artificial cerebrospinal fluid (ACSF) of the following composition (mM): NaCl 124, KCl 3, MgSO4 1.25, CaCl2 2.5, KH2PO4 1.25, NaHCO3 22, Glucose 10. Transverse slices of 400 mm thickness were prepared from the hippocampus using a McIlwain tissue chopper. Oblique slices of 400 mm thickness, about 40 degrees rostral-up to the horizontal plane, were cut from the neostriatum using a vibratome. The slices were kept in ACSF at room temperature and gassed continuously with a mixture of 95% O2 and 5% CO2 to attain a steady-state oxygenation level and maintain a pH of 7.4. Slices were allowed to recover at least 1h before recording began at 33˚C.
Field potentials were recorded in the stratum pyramidal of area CA1 following electrical stimulation of the Schaffer collateral pathway in the hippocampus. In the striatum preparation, stimulation took place on the corpus callosum or the external capsule to activate the cortical inputs (0.1 Hz). Recording electrodes were glass micropipettes filled with 2M NaCl, resistance ranged between 6 and 10 MW. The stimulus strength was adjusted to evoke half-maximal field potentials which were averaged over 8 to 10 sweeps.
Data are presented as mean ± standard error of the mean (S.E.M.). For statistical analyses of the responses before, during and after drug application were compared using students’ test. All drugs were added to the perfusion fluid. Adenosine, AOPCP was obtained from Sigma (Deisenhofen, Germany) and DPCPX, iodotubercidin from Research Biochemicals Inc. (Natick, MA, USA).
3. Results
AOPCP (50 mM) significantly (p < 0.01) enhanced the amplitude of population spikes by 34% ± 17.5% (n = 11) in the hippocampus and by 26.5% ± 14% (n = 7) in the neostriatum. The effect of AOPCP was reversible within 10 to 20 minutes (Figure 1). This indicates that a portion of extracellular adenosine is derived from adenine nucleotides. The population spike enhancement by AOPCP was maximal at these concentrations.
Exogenously applied adenosine, at 40 µM, caused a larger decrease in amplitude of field potentials in both preparations: to 47.5% ± 12.5% of control (n = 5) in the hippocampus and 60% ± 15% of control (n = 5) in the neostriatum (Figure 2).
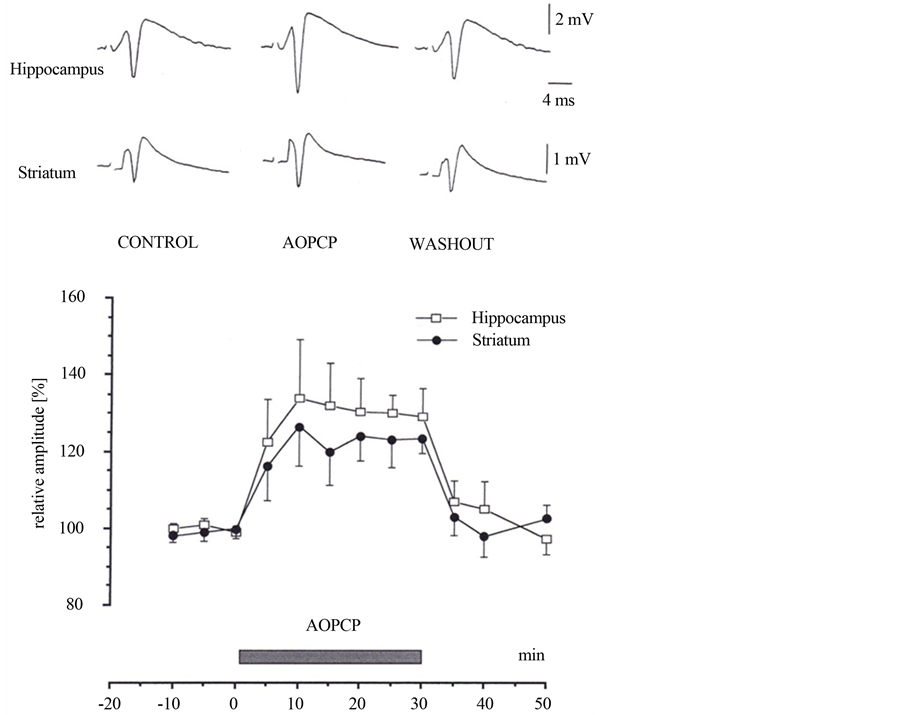
Figure 1. The Effects of a, b methylene adenosine 5'-diphosphate (AOPCP) on field potentials. Averaged field potentials recorded in the absence (control and washout) and during the application of AOPCP (40 mM). AOPCP enhanced the amplitude of the population spikes (mean ± S.E.M., n = 11 hippocampus and n = 7 striatum) in field potentials of hippocampus and neostriatum. The effect of AOPCP was reversible; the amplitude of population spikes was almost at the control level after a washout period of 10 to 20 minutes. Hippocampus: open circles, n = 11 and striatum: filled circles, n = 7.
DPCPX (5 mM), a selective adenosine A1-receptor antagonist [2] , increased the amplitude of population spikes by 68% ± 10.5% (n = 7) in hippocampus and 53.5% ± 7.5% (n = 5) in striatum (Figure 3). Comparison of the effect of the adenosine receptor antagonist DPCPX with the inhibitor of ectonucleotidase AOPCP shows in both structures statistically significant difference (paired t test, t = 4.61, p = 0.0003 for hippocampus, t = 3.89, p = 0.003 for striatum).
4. Discussion
In a previous study [17] we have demonstrated an adenosine mediated inhibitory effect of iodotubercidin, a potent inhibitor of adenosine kinase in the hippocampus. We report now a similar reversible effect by iodotubercidin (5 mM) in the neostriatum, by 72.5% ± 16.5% (n = 5) (Figure 4).
Inhibition of ecto-5'-nucleotidase with AOPCP enhances field potentials in superfused hippocampal slices by 35% and by 25% in neostriatal slices. Inhibition of the catabolizing enzyme prevents the degradation of the nucleotides to adenine nucleoside, thus leading to enhanced excitability of cells in the CA1 region of hippocampus and in neostriatum. AOPCP increased the amplitude of the population spikes in both preparations lesser that observed with an A1-receptor antagonist (Figure 1, Figure 3).

Figure 2. The effect of exogenous adenosine on field potentials recorded from the CA1 pyramidal cell layer in the hippocampus following stimulation of the stratum radiatum and from the striatum following stimulation of the cortico-striatal pathway. Normalised amplitude of averaged field potentials recorded in the absence (control and washout) and during the application of adenosine (40 mM) are displayed. The amplitudes are means of 5 population spikes in each case. Hippocampus: open circles, n = 5 and striatum: filled circles, n = 5.

Figure 3. Effects of 1, 3-dipropyl-8-cyclopentylxanthine (DPCPX) on field potentials recorded from the CA1 pyramidal cell layer in the hippocampus following stimulation of the striatum radiatum and from the striatum following stimulation of the cortico-striatal pathway. Normalised amplitude of averaged field potentials recorded in the absence (control and washout) and during the application of DPCPX (5 mM) are displayed. Hippocampus: open circles, n = 7 and striatum: filled circles, n = 5.

Figure 4. Effects of iodotubercidin (ITu) on field potentials in neostriatum. Averaged field potentials recorded in neostriatum in the absence (control and washout) and during the application of ITu (5 mM) following stimulation of the cortico-striatal pathway. The effect of ITu was reversible; the amplitude of population spikes was almost at the control level after a washout period of 10 to 20 minutes. The amplitudes are means of 5 population spikes.
The ecto-5'-nucleotidase is abundant on the surface of neuronal membranes [14] [15] [19] [20] There are at least two types of ectonucleotidases which are specific for different substrates: ecto-ATPase is specific for ATP, ecto-5'-nucleotidase hydrolyses both 5'-nucleoside and AMP. They are bound to the cell membrane and directed towards the extracellular space [21] [22] have shown that AOPCP blocks responses to the nucleotides but has no significant effects on responses to adenosine itself.
Intracellular adenosine is produced either by hydrolysis of AMP (5'-AMP) via cytosolic 5'-nucleotidase or by hydrolysis of SAH via SAH-hydrolase. Adenosine kinase, which phosphorylates adenosine to AMP, and adenosine deaminase, which deaminates adenosine to inosine, are both thought to be predominantly cytoplasmic enzymes [23] . Adenosine can cross cell membranes either by active transport or by facilitated diffusion, a carrier mediated process in which adenosine follows its concentration gradient [24] . The transporters operate bi-directionally, depending on the adenosine concentrations in the cytosol and the extracellular space.
Adenosine has a role as a modulator of synaptic activity in the CNS [25] . The modulation of the excitatory synaptic transmission is occurred by adenosine released from hippocampal pyramidal neurons [26] . Data from this and other studies [17] [18] [27] suggest that adenosine kinase plays a key role in the regulation of extracellular concentration of adenosine. Intracellular formation of adenosine is the most important source of adenosine in the extracellular space, but a significant amount comes from dephosphorylation of extracellular nucleotides.
NOTES
*Corresponding author.