Hemodynamic Changes during Off-Pump Coronary Ar-tery Bypass Graft Surgery: Experience Using Pericardial Bands for Target Stabilization ()
1. Introduction
The off-pump coronary artery bypass graft surgery (OPCAB) is being expanded in recent years because of the advantage of avoiding cardiopulmonary bypass (CPB) and its attendant complications, improvements in techniques as well as recent introduction of instruments to optimally stabilize the coronary targets on a beating heart. [1-5]. The introduction of mechanical stabilizers such as CTS pressure mechanical stabilizers, epicardial fixation by suction devices and the Medtronic-Utrecht Octopus system, (Medtronic Inc, Minneapolis, MN), produced adequate cardiac immobilization of the coronary target site for performance of a safe anastomosis [6]. However, the hemodynamic instability that occurs during positioning of the heart and stabilization of the coronary targets with interruption of coronary blood flow are important factors limiting the safe performance of OPCAB. Several studies have been performed noting the changes in hemodynamic parameters and management of hemodynamic instability during OPCAB using mechanical stabilizers for coronary target stabilization and hemodynamic variables measured in most of these studies were either sparse or were measured only once during procedure without noting the progress of hemodynamic change during coronary artery anastomosis [7,8]. This study evaluated the use of pericardial bands for coronary target stabilization, noting serial hemodynamic parameters recorded during coronary artery anastomoses.
2. Material and Methods
During a three year period 250 patients with coronary artery disease participated in this prospective study and all were planned to undergo OPCAB. Patients with significant preoperative hepatic or renal dysfunction, bleeding disorder or uncontrolled arrhythmia were excluded. The characteristics of this patient group are shown in Table 1. This study was approved by the institutional review board and ethics committee of the NWBIMC and informed consent was obtained from all patients.
2.1. Surgical Technique
All preoperative cardiac medications were continued till the morning of surgery, but digoxin was withheld. Morphine sulphate 0.05 mg/kg IM was given an hour before the surgery as premedication. In the operating room, EKG leads II and V5 were monitored and the radial artery was cannulated for continuous monitoring of arterial blood pressure and blood gas analysis. Anesthetic induction was done with 2.0 - 3.0 mg of midazolam, 1.0 - 3.0 µg/kg of sufentanil, and 50 mg of rocuronium. Anaesthesia was maintained with 0.2 - 0.5 vol% of isoflurane and
continuous intravenous infusion of 0.5 - 1.5 µg/kg/min of sufentanil and vecuronium. Ventilation was controlled with oxygen-air mixture (FiO2 0.6) to maintain end-tidal CO2 in 35 - 38 mmHg. After anaesthetic induction isosorbide dinitrate infusion at 0.5 µg/kg/min and diltiazem infusion at 1mcg/kg/min were started and the SwanGanz catheter (Swan-Ganz, Baxter Healthcare Co., Irvine, CA, USA) was inserted through the right internal jugular vein into the main pulmonary artery. The temperature of the operating room was kept above 25˚C and all the fluid (infusions and irrigations) was warmed to prevent hypothermia. Warm humidifier was connected to the breathing circuit. Patients were also warmed with warm mattress and after the venous harvest; the lower limbs were wrapped with ace bandage and covered with a warm blanket.
After median sternotomy, adequate amount of fluid (1.5 - 2.0 litters) was administered to keep the CVP around 10 mm∙Hg. After dissection of left internal mammary artery (LIMA), and harvesting 3 pericardial bands intravenous heparin was injected (1 mg/kg) and activated clotting time was maintained over 250 s during the coronary anastomoses. To expose the left lateral side of the heart and to elevate the apex, four deep pericardial stay sutures were placed at aorto-pericardial reflection, left superior and inferior pulmonary veins and between left inferior pulmonary veins and inferior vena cava [9]. Traction on these stay sutures positioned the heart to expose the coronary artery target, and then appropriate pericardial bands were snared for stabilization of the coronary targets for anastomosis. The technique of harvesting the pericardial bands and coronary target stabilization using pericardial bands was as described earlier [10]. Briefly, this technique involved fashioning 3 pericardial bands of 2.5 cm wide and 8 to 10 cm long from the body of the anterior pericardium after median sternotomy incision was completed. Two pericardial bands are positioned one above and one below the coronary target and the distal ends of the bands were snared to the pericardial well for stabilization of the anastomotic site. Trendelenburg position was often employed during anastomosis. In case of hypotension, nor-epinephrine was given to maintain mean systemic arterial pressure (MAP) above 60 mmHg. In this study (250 patients) an average of 2.8 ± 0.7 coronary arteries were bypassed in a patient. The time for performing three coronary artery anastomoses was 48 ± 12 min (mean). The time required to anastomose each coronary artery are following: 17.6 ± 3.4 min for LAD, 17.6 ± 1.8 min for obtuse marginal branch of LCX and 17.9 ± 1.8 min for RCA.
2.2. Hemodynamic Monitoring
The hemodynamic variables recorded were heart rate (HR), central venous right atrial pressure (CVP), systemic mean arterial pressure (MAP), pulmonary artery pressure (PAP), pulmonary capillary wedge pressure (PCWP), cardiac output (CO), and systemic venous oxygen saturation (SvO2). The cardiac index (CI), stroke volume index (SVI), left ventricular stroke work index (LVSWI), and systemic and pulmonary vascular resistances were calculated according to standard formulas. Hemodynamic measurements were recorded after pericardiotomy for baseline value, then 3, 5, 10, and 15 min after snaring the pericardial bands for coronary artery target stabilization during each coronary artery anastomosis, after the removal of the bands and after sternal closure. The amount of nor-epinephrine used to maintain MAP for each anastomosis was also recorded.
2.3. Statistical Analysis
All hemodynamic variables were expressed as the mean value ± SD. Hemodynamic changes for each coronary graft anastomosis were compared to baseline values using repeated measures of analysis of variance (ANOVA). The comparisons of variables between coronary arteries were analyzed with one-way ANOVA. Percent changes of CI and SvO2 for baseline values in each coronary artery were analyzed with linear regression. A P value of <0.05 was considered as statistically significant. Data were analyzed with SPSS for Windows, release 10.0 (SPSS Inc., Chicago, IL, USA).
3. Results
During RCA anastomosis, the HR was significantly increased throughout the procedure (T3 to T15 and at sterna closure) compared to the baseline value, but MAP decreased only initially at T3. The MPAP and PCWP did not show significant change compared with baseline, except a brief elevation of PCWP at T3. The CVP at T3 to T15 significantly increased and the CI, SvO2, SVI and LVSWI at T3 to T15 significantly decreased compared with baseline. The SVRI did not change compared with baseline except increase at T10.
During obtuse marginal branch of LCX anastomosis, the HR at T3 to T10 and at sternal closure (Ts), signifycantly increased, but without any change in MAP. The CVP at T3 to T15 and PCWP at T10 to T15 significantly increased compared with baseline values. The cardiac index (CI), SvO2, SVI, and LVSWI showed significant decrease at T3 to T15 compared with baseline. The SVRI at T3 to T15, significantly increased compared with baseline values During LAD anastomosis, the HR was significantly increased only at sternal closure without a change in MAP. The CVP, MPAP, PCWP significantly increased from T3 to T15 compared with the baseline values. The CI, SvO2, SVI, and LVSWI were significantly decreased from T3 through T15 and SVRI was significantly increased at T15 compared with baseline values. Hemodynamic data during coronary artery bypass graft anastomoses are shown in Tables 2 and 3.
On comparing variables between the 3 coronary artery anastomoses, no differences were noted in HR, MAP and CVP during entire period of surgery (B to Ts). MPAP was significantly lower in OM at T3, and in RCA at T3

Table 2. Hemodynamic data during coronary artery bypass graft anastomosis. (Mean ± SD).
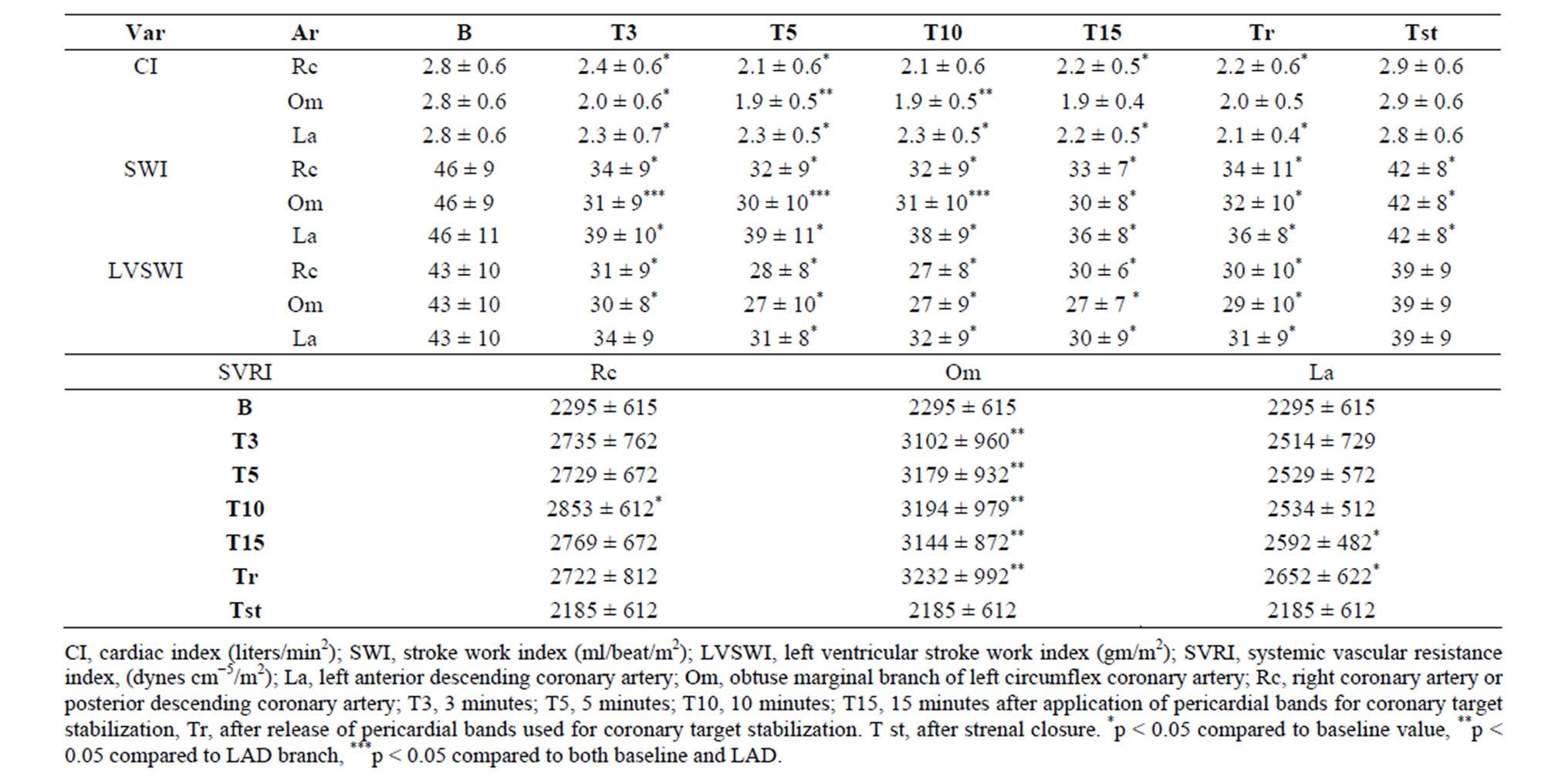
Table 3. Calculated hemodynamic data during coronary artery bypass graft anastomosis. (Mean ± SD).
and T10 as compared to LAD. The CI and SvO2 from T3 to T15 were significantly lower in OM as compared to LAD. The SVI was significantly lower in OM than in LAD from T3 to T10. The LVSWI of LAD at T3 was higher than that of OM and RCA. The SVRI was significantly higher in OM form T3 to Tr compared to LAD. The changes of CI and SvO2 of each coronary artery were compared. The decrease in CI was greater in OM compared to that of LAD (Figure 1). Similarly the decrease in SvO2 was greater in OM compared to that of LAD (Figure 2). Noticeably, the change of CI in LAD showed linear decrease with time though the change of CI in OM and RCA did not show the same pattern. The total doses of nor-epinephrine used to maintain MAP over 60 mmHg during performance of anastomoses were 87.1 ± 106.4, 74.0 ± 60.9 and 216.9 ± 282.6 µg for LAD, RCA, and OM vessels respectively. The amount of norepinephrine used was significantly larger in OM than in other two arteries.
4. Comment
Though during earlier years, the OPCAB surgery was performed in patients with good ventricular function and a single or two-vessel disease, this has been recently performed in patients with calcified and atheromatous ascending aorta, renal failure, cerebrovascular disease, or in patients with poor ventricular function or advanced heart failure for avoiding CPB-related complications [11]. The hemodynamic deterioration occurring during coronary anatomosis is an important concern of OPCAB pro-

Figure 1. The changes in cardiac index during each coronary graft anastomosis. ∆ CI% = (cardiac index for each period/baseline cardiac index) ×100. The vertical bars represent standard deviation. B = baseline after pericardiotomy, T3 = 3minutes after stabilization of a coronary target. T5 = 5 minutes after stabilization of a coronary target, T10 = 10 minutes after stabilization of a coronary target, T15 = 15 minutes after stabilization of a coronary target, Tr = after release of pericardial bands used for stabilization of a coronary target and post grafting period. LAD = left anterior descending coronary artery, RCA = right coronary artery, OM = obtuse marginal branch of left circumflex coronary artery, the white arrows indicate p < 0.05 compared to LAD.
cedure. This study evaluated the hemodynamic variables during coronary artery anastomoses using deep pericardial stay sutures and pericardial bands for coronary artery target stabilization and all hemodynamic variables were

Figure 2. The changes in mixed venous oxygen saturation (SvO2) during each coronary graft anastomosis. ∆ SvO2% = (SvO2 for each period/baseline SvO2) × 100. The vertical bars represent standard deviation. B = baseline after pericardiotomy, T3 = 3minutes after stabilization of a coronary target. T5 = 5 minutes after stabilization of a coronary target, T10 = 10 minutes after stabilization of a coronary target, T15 = 15 minutes after stabilization of a coronary target, Tr = after release of pericardial bands used for stabilization of a coronary target and post grafting period. LAD = left anterior descending coronary artery, RCA = right coronary artery, OM = obtuse marginal branch of left circumflex coronary artery. The white arrows indicate p < 0.05 compared to LAD.
recorded as a function of time during each coronary artery anastomosis. After the initial hemodynamic change induced by the heart positioning and snaring the pericardial bands to the pericardial well, no further significant hemodynamic deterioration or improvement were noticed. There was no case that could not perform planned anastomosis or convert to CPB due to hemodynamic instability. Though incidence of emergency conversion during OPCAB has decreased with increasing surgical experience, outcomes in these patients continued to be worse than those in non-converted patients. Therefore, careful monitoring of hemodynamic changes and safer bail out strategies are still warranted [12].
The CI and SvO2 significantly decreased at the beginning of the anastomosis in all three coronary arteries although the MAP was constantly maintained throughout the procedure. In the OM and RCA, immediately after snaring the pericardial bands for stabilization CI and SvO2 decreased abruptly, but further decrease did not occur. However, the cardiac index was decreased in linear fashion with time during LAD anastomosis. Compression of the left ventricular outflow tract and abnormal diastolic expansion secondary to direct deformation of the left ventricular geometry are proposed mechanisms for hemodynamic derangements with compression type stabilizer. Coronary occlusion during the anastomosis can have additional effects on left ventricular function, depending on the status of collateral flow [8].
During anastomosis of anterior arteries such as LAD coronary artery target stabilization results in direct compression of the right ventricular outflow tract and the right ventricular end systolic and diastolic volumes increase. As a result, the left ventricular diastolic compliance is reduced through the interventricular relationship and the PAP and PCWP increase [13]. During LAD anastomosis, the obstruction of the right ventricular outflow tract may be the possible reason for the gradual decrease in CI, although reduction in blood supply to the large portion of left ventricular myocardium with impaired global ventricular function might have contributed, especially if intracoronary shunt was not used.
During OPCAB the sustained hemodynamic compromise may adversely affect major organ function, especially if the total time required for anastomosis is prolonged. The grafting of the posterior coronary branches (OM and posterior descending artery) through sternotomy requires anterior displacement of the beating heart and this often causes hemodynamic compromise [14,15]. The sustained decrease in CI (<2.0l/min) and SvO2 (<70%) during whole period of OM anastomosis should warn of impending major organ damage though none of patients, in this study, had developed neurologic complications or an evidence of organ damage. If high risk patients are subjected to OPCAB the sustained or repetitive low cardiac output status might have deleterious effect on organ function, therefore, it is increasingly necessary to adequately monitor and manage variables like CI, and SvO2. The SvO2 represents both delivery and consumption of oxygen and changes earlier compared to the continuous cardiac output measurements. Though the changes in cardiac output might be too slow to monitor the cardiac status during the manipulation and tilting of the heart, it is still a useful variable as a trend during the entire surgical period.
The optimal management of patients undergoing OPCAB has been focused on the maintenance of stable hemodynamics such as MAP, HR and cardiac rhythm as well adequate tissue perfusion during coronary anastomosis [16-18]. The intravenous fluid loading and head down position were often used to compensate decreased MAP and CO while the use of inotropic drugs was relatively contraindicated [19]. The mechanical displacement and compression of the heart during anastomosis results in decrease in the CI and use of inotropic drugs may further increase myocardial oxygen consumption and induces myocardial ischemia [20-22]. In the study, it was found that the augmentation of preload with head down position and fluid loading was not adequate enough to compensate the reduced CO during anastomosis. Therefore, management to maintain the CO and SvO2 without subsequent adverse cardiac effects is essential and inotropic drugs that would not adversely increase chronotopism of the heart and myocardial oxygen consumption may be useful [22,23].
In conclusion, this study represents our experience using four deep pericardial stay sutures and pericardial bands for coronary artery target stabilization, recording serial hemodynamic changes occurred during OPCAB surgery. Other techniques using mechanical stabilizers, slings and tapes to expose and stabilize the coronary vessel may result in similar magnitude of hemodynamic changes which are largely dependent on the surgical technique and surgeon’s skill. Though HR and MAP were maintained constantly throughout the procedure, SvO2 and CI decreased significantly at the beginning of OM and RCA anastomosis, and maintained constantly throughout, while the CI and SvO2 decreased further as a function of time during LAD anastomosis. Therefore, close monitoring with adequate interventions are necessary to prevent decreases in CI and SvO2 and avoiding conversion to on-pump technique.