Local Drug Delivery Strategy for Cancer Treatment: Use of Biocompatible Sol-Gel-Derived Porous Materials ()
1. Introduction
The most common combinational strategies for effective cancer treatment generally involve the combination of multiple chemotherapeutic agents, chemotherapy with radiotherapy, etc. [1,2]. However, one of the biggest problems in cancer therapy is that of dose-dependent toxicity toward the non-cancerous cells, since poor drug accessibility to the tumor site necessitates high doses of drug treatment [1]. Hence, in order to improve overall survival and quality of life, it is now desired to increase the bioavailability of drugs to the disease sites and to contain the delivery to the cancerous tissues.
Existing systems for this approach rely either on 1- systemic delivery (polymer nanoparticle systems, for example, which are administered intravenously but which have difficulty being well localized because of sequestration by the reticulendothelial system [2,3]) or 2- controlled release drug delivery depot systems for implantation intra-tumorally or adjacent to cancerous tissue [3]. Though such tested materials are tolerated well in vivo, they generally have poor solubility in organic solvents thus restricting the inclusion of water-insoluble chemotherapy agents.
Previous reports using porous silica substrates have all been sol-doped systems—thus requiring water soluble additives [4-9]. In order to circumvent these problems, we propose an approach previously unreported, that of post-doping biocompatible silica gels [10] with the adequate chemotherapy agent. With this in mind, we have begun tests first to determine the capacity of the gels to absorb the molecular agents and then, in a second step, to test the ability of the gels to release their absorbed molecular agents to the environment.
2. Sample Preparation
Mesoporous silica matrices were obtained using the ammonium hydroxide catalyzed sol-gel process of hydrolysis and condensation of tetramethyl orthosilicate (TMOS, ≤99%) at 30˚C, under atmospheric pressure [11]. The samples obtained were heat-treated first at 120˚C and then at 850˚C in air to eliminate all residual organic species and the major part of Si-OH, to exclude water from the pores and also to stabilize the resulting xerogels. The resulting monoliths were glassy, colorless, and crack-free. These xerogels were soaked for 12 h in aqueous solutions of test molecules (clotrimazole and the photoluminescent primaquine diphosphate) and of the anticancer agent vinblastine sulphate. The doping solutions consisted of 100 mg of the test drug molecule in 5 mL of distilled water. Accordingly, each sample contained about 12.3 mg of drug per gram of SiO2 gel. In the case of vinblastine sulphate, 10 mg were dissolved in 5 mL of water. The gels were then dried at 50˚C for 24 h. All precursors and solvents were purchased from Aldrich and all compounds were used without further purification.
Silica, clotrimazole, primaquine diphosphate, vinblastine sulphate, clotrimazole/silica, primaquine diphosphate/silica and vinblastine sulphate/silica will be hereafter denoted as SiO2, Cl, Pr, Vi, Cl-Si, Pr-Si and Vi-Si, respectively.
3. Experimental Techniques
In order to determine the porosities of the silica gels before and after dopage, adsorption-desorption isotherms were obtained using a BET Quantachrome Autosorb-1 system.
Raman spectra of undoped and doped gels were recorded with a Horiba-Jobin Yvon Labram HR spectrometer excited with the 632.8, 532 and 473 nm lines of a He-Ne laser and with a Horiba-Jobin Yvon visible UV micro Raman spectrometer excited with the 266 and 325 nm lines of a HeCd laser. In order to follow the amount of dopant molecules released into solution, UV-visible spectra were measured with a double-beam Cary 5000 instrument.
4. Results and Discussion
4.1. Characterization of Drug Molecules and Carrier Matrices
The chemical structures of the tested drugs (Cl, Pr and Vi) are presented in Figure 1. Photographs of an undoped porous silica xerogel and a Pr-doped silica matrix are shown in Figure 2(a). The cylindrical xerogels obtained after doping with drug molecules remained unbroken and without visual inhomogeneities.
Two important aspects in the design and testing of a delivery system is whether or not the drug molecule can be detected in the carrier matrix and whether or not there is interaction between the two. In the present work, the gels were first examined to determine the best method of detecting the absorbed species. The assignments of the Raman bands observed for SiO2 xerogel, in Figure 2(b), are presented in Table 1. Of these bands, those around 430, 490 and 600 cm–1 assigned to the network Si-O-Si bending vibration, and to the symmetric stretching modes of Si-O bonds in 4- and 3-membered silica rings, respectively, are the most important signals of the silica matrix. In the micro-Raman spectra of Figure 2(c), test results obtained using primaquine diphosphate before (A) and
(a)
(b)
(c)
Figure 1. Structural formulas of (a) clotrimazole, (b) primaquine diphosphate and (c) vinblastine sulphate.
after (B) doping are presented. These spectra, obtained with an excitation at 632.8 nm, show the fluorescence interference often encountered with colored samples. However when excited with UV excitation at 266 nm, the spectrum (C) shows a fluorescence-free signal, which unfortunately can be assigned uniquely to carbon thus demonstrating that the high laser energy resulted in a calcination of the samples. Using the characteristic vibrational frequencies for Pr which are presented in Table 2, it can be seen that in spite of the fluorescence, all the bands characteristic of Pr are detected in the doped gel (spectrum B of Figure 2(b)). In the region of 463 cm–1, one can see the contribution of the Si-O-Si matrix. The fact that no frequency changes are observed between A and B, indicates that there is no interaction between Pr and SiO2. As the molecule is not affected by the matrix, when it is released, it will still be in its normal active form.
In a similar way, the spectrum of Cl-Si, in Figure 2(d), shows bands characteristic of Cl (See Table 3: 1008, 1161 and 1460 cm–1). In this case, as the drug is colorless, there is no problem with fluorescence and the spectrum of the gel is clearly obvious (430, 492 and 815 cm–1). Similarly, Vi could be identified in the spectra of Vi-Si samples.
These data show that micro-Raman spectroscopic investigation of doped silica matrices can detect the presence of doping species and can also indicate whether or not there is an interaction between the carrier matrix and the drug molecule. Obviously, an interaction would imply a structural modification of the drug molecule and hence a possible change in its mechanism of interaction.
The porosity of the drug-incorporated matrix also plays an important role in release kinetics. For determination of the texture (porous volume, pore size distribu-
Table 1. Assignments of Raman bands of silica xerogel.

Table 2. Assignments of Raman bands of primaquine diphosphate powder.
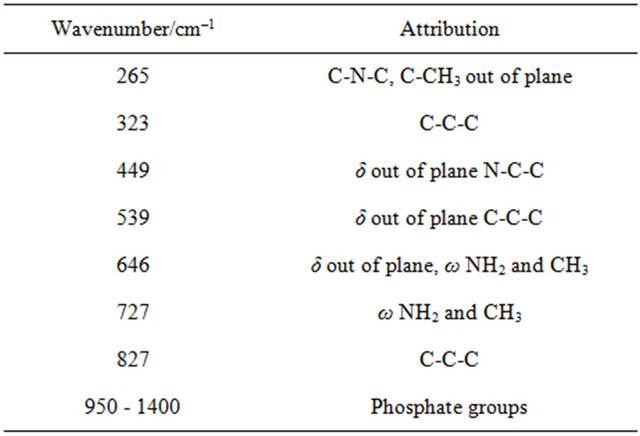
Table 3. Assignments of Raman bands of clotrimazole powder.
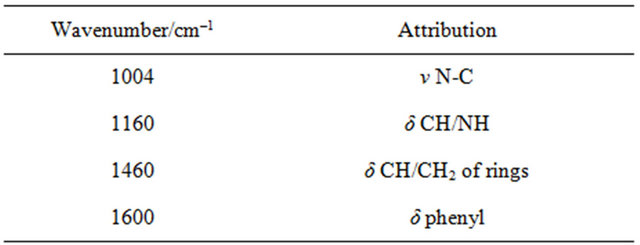
tion and pore interconnection), the nitrogen adsorptiondesorption technique was used. The shape of the adsorption-desorption isotherms and the type of hysteresis loops were described using the Brunauer et al. model and the de Boer correlations, respectively [12,13]. The specific surface areas were determined using the Brunauer-Emmett-Teller (BET) method [14]. The pore-size distributions were thus given by the Barett-Joyner-Hal-enda (BJH) formula which determines the derivative of the desorbed volume as a function of the pore diameter [15]. For the following sections, only results concerning primaquine diphosphate will be presented.
As can be seen in Figure 3(a), the isotherms for undoped and Pr-doped xerogels show reversible sections at low pressures and hysteresis loops at higher pressures. These loops of the type IV isotherms are characteristic of mesoporous samples with interconnected pores. Com-
(a)
(b)
Figure 3. (a) Nitrogen adsorption-desorption isotherms of SiO2 and Pr-Si; (b) BJH desorption pore-size distributions of SiO2 and Pr-Si.
parison of the two curves shows an increase in total pore volume after doping with Pr (from 0.62 (SiO2) to 0.94 cm3×g–1 (Pr-Si)). The corresponding specific surface area increases from 375 to 671 m2×g–1. Determination of the pore diameters shows that the mean pore diameter (Figure 3(b)) decreases with doping, from 6 nm for SiO2 to 4.7 nm for Pr-Si.
These results demonstrate that the doping has a marked effect on the porous structure of this carrier matrix. Remembering that these materials are porous gels and not glasses, the data suggest that during post-doping, the gel behaves like a sponge, with inflation of pore volume and specific surface. The apparent decrease in pore size after doping could be due to the occupation of space within the pores by the primaquine diphosphate molecules, which would limit access of the infiltrating nitrogen gas.
Another important aspect of these results is that these porous gels can be adapted to the use with a multitude of drug molecules. For, as the carrier pores apparently expand during soaking procedures, it can be deduced that the size of the matrix pores does not pose rigid limitations on the size of the molecules to be inserted.
4.2. Release Properties
Release properties of the SiO2 matrix were studied by soaking the Pr-doped gels in distilled water in order to test the discharge of the drug molecules into the surrounding liquid. In order to quantify the amount of drug eluted, UV-visible absorption spectra were first measured for standard solutions of primaquine diphosphate in order to establish a Beer-Lambert curve (See Figure 4(a)).
UV-visible absorption spectra were then measured of the liquid used for soaking the samples at various times up to 10 days of elution (Figure 4(b)). The drug release pattern for Pr-Si, presented in Figure 4(c), shows a biphasic behavior. There is an initial “burst” of 3.5 mg per gram of SiO2 gel, released on the first day. The amount of primaquine diphosphate molecules diffused from the gel decreases to 0.40 mg per gram of SiO2 gel after 4 days. A sustained diffusion of this amount of primaquine continues up to 10 days.
As the samples were consistently washed before elution measurements, all molecules released were issue of the inner pores of the xerogel, not from its surface. The decreased rate of diffusion of the drug from the matrix with time might be due to the lesser driving force for diffusion which is proportional to the availability of entrapped drug molecules.
In general, in the case of an insoluble porous carrier, in which the release is governed by diffusion of the drug from the matrix, release kinetics follows a conventional Higuchi relationship (variation of drug vs. square root of time). Accordingly, the plot of Figure 4(d) shows a two-step release profile. Since our Raman studies have shown that there are no chemical interactions between the drug and the matrix, this behavior must be attributed to physical entrapping and the elution is, in fact, therefore diffusion controlled.
These release properties show that a porous silica matrix can be effectively post-doped by immersion in a drug solution. Moreover, the resulting systems act as effective carriers for drug delivery making it possible to maintain a sustained release of the drug molecules.
5. Conclusions
The present work shows that not only is sol-gel processing a practical technique for the fabrication of carrier substrates, but that post-doping procedures are easily adaptable for the conception of loaded drug delivery materials. These silica xerogels post-doped with aqueous solutions of several drug molecules have been studied by Raman and UV-visible absorption spectroscopies and nitrogen adsorption-desorption techniques.
Nitrogen adsorption-desorption isotherm studies have shown that the present biocompatible silica materials have high surface areas and sufficiently large pore-sizes, making them good for post-doping. BET measurements show that soaking the gels in drug solutions causes an increase of the total pore volume and the specific surface
area. Raman spectra show that after post-doping the drug molecules are indeed entrapped within the silica matrix, but with no apparent chemical interactions between the two.
Use of the Beer-Lambert curve for Primaquine diphosphate solutions and the UV-visible spectra of the solutions used for soaking the Pr-Si samples made it possible to quantify the amount of Pr released from the loaded gels. Results show that drug release begins with an initial value of 3.5 mg per gram of SiO2 gel. The elution decreases to a sustained value of 0.40 mg per gram of SiO2 gel for at least 10 days.
These studies point to the possibility of developing these systems for implantable drug-release agents.