1,25-Dihydroxyvitamin D3 effects on the regulation of the insulin receptor gene in the hind limb muscle and heart of streptozotocin-induced diabetic rats ()
1. INTRODUCTION
1,25-dihydroxyvitamin D3 (1,25D3), acting through the vitamin D receptor (VDR), can produce multiple biological effects in different physiological systems [1]. VDR is a member of the nuclear receptor superfamily of transcription factors [2] that exerts its actions preferentially as a heterodimer with the retinoid X receptor (RXR), binding to specific nucleotide sequences termed vitamin D response elements (VDREs) located within the vicinity of 1,25D3 target gene promoters. A VDRE generally consists of two direct imperfect repetitions of six nucleotides separated by a three-nucleotide spacer. The VDR usually occupies the 3’ half-site while the RXR binds to the 5’ half-site [3]. Differences in the sequence of these halfsites, the spacer and the sequences flanking and/or overlapping the VDREs, together with a wide variety of coregulatory factors, appear to modulate the transcriptional regulation by 1,25D3 [4].
Our group reported the first demonstration that 1,25D3 was able to increase the expression of the human insulin receptor (INSR) gene, the INSR number and the insulin response to glucose transport and glucose oxidation in U-937 human promonocytic cells [5]. These in vitro effects of 1,25D3 were accompanied by the transcriptional activation of the human INSR gene [6] and by the enhanced expression of the human VDR at the level of both RNA and protein [7]. In support of a transcriptional regulation of this INSR gene by VDR, our group identified a functional VDRE located between −761/−732 bp of the human INSR gene promoter [8].
1,25D3 also exerts in vivo effects on insulin secretion and insulin sensitivity. Treatment with 1,25D3 enhanced pancreatic insulin secretion while vitamin D deficiency inhibited rat pancreatic secretion and turnover of insulin, leading to impaired glucose tolerance [9]. Replacement therapy with 1,25D3 in rats with vitamin D deficiency reversed these abnormalities [10]. The administration of 1,25D3 in animal models of diabetes, including the diabetes induced by streptozotocin (STZ), improved the diabetes attenuating pancreatic islet damage and increasing insulin sensitivity [11]. We reported that 1,25D3 treatment reversed the over-expression of the rat INSR gene in the liver and adipose tissue of STZ-induced diabetic rats without altering the normal expression of this gene in the kidney [12]. These effects were accompanied by a normalization of the number of INSRs and an improvement of the insulin response to glucose transport in adipocytes of these animals. In support of a transcriptional participation of 1,25D3 in these processes, we localized by computer search two candidate DNA sequences in the rat INSR promoter containing virtual VDREs [12].
With these antecedents, in the present work we examine the possible existence of in vivo 1,25D3 effects in other two important insulin target tissues: the hind limb muscle and heart of non-diabetic and STZ-induced diabetic rats. Both tissues express INSRs [13,14] although with different receptor capacity and sensitivity to developed insulin resistance [15,16]. Both tissues also express VDRs [17-19], providing the support for possible genomic effects of 1,25D3 in these tissues. We hypothesized that STZ-induced diabetic rats could be more susceptible to 1,25D3 that non-diabetic rats and that the diabetes induced by STZ could provoke tissue specific changes that could alter the genomic 1,25D3 response. We also postulated the possible alteration by 1,25D3 of INSR mRNA levels and the possible participation of transcriptional and/or posttranscriptional mechanisms in this regulation.
2. MATERIALS AND METHODS
2.1. Experimental Animals
Male Wistar rats weighing 200 - 220 g at the onset were used. During the 15 days of the experimental period, the rats were kept under standard conditions of light and temperature with free access to tap water and standard laboratory chow (Panlab, A04) containing 8.8 mg/g of calcium, 5.9 mg/g of phosphorus and 1.5 IU/g of vitamin D. Four groups of rats were employed in this study. The first group was comprised of non-diabetic rats receiving sham-treatments during the 15 days of experimental period (non-diabetic rats). The second group included nondiabetic rats i.p. injected with 1,25D3 (Calcijex, Abbot) (150 IU/Kg [3.75 µg/Kg] once a day, for 15 days) (1,25D3-rats).The third group consisted of rats rendered diabetic by a single injection of STZ (Sigma) (65 mg/kg) on the 8th day of the 15-day period (STZ-rats). Finally, the fourth group was comprised of rats rendered diabetic by STZ on the 8th day, and i.p. injected with 1,25D3 (150 IU/Kg [3.75 µg/Kg] once a day, for 15 days) (STZ + 1,25D3-rats). The National Guide for the Care and Use of Laboratory Animals was strictly followed during this study.
On sacrifice, fed rats were decapitated without anaesthesia and trunk blood samples were recovered for plasma measurements. The hind limb muscle and heart were immediately removed, and frozen in liquid nitrogen for nucleic acid and protein determinations.
2.2. Analytical Methods
Plasma insulin levels were determined by radioimmunoassay using rat insulin as standard [20]. Plasma concentrations of glucose, 25-hydroxyvitamin D3, calcium, phosphorus and proteins were estimated using commercially available techniques. The total DNA and RNA contents of the homogenates from individual tissues were estimated by spectrofluorometry. The protein content was determined by the Bradford method.
2.3. Northern Blot Assays
For Northern blot assays, RNA samples (30 µg) of hind limb muscle and heart, were denatured, electrophoresed in 1.1% agarose-formaldehyde gels and blotted onto nylon membranes as previously described [21]. Ethidium bromide staining of the 28 S and 18 S ribosomal RNAs was routinely checked before blotting as a control for sample loading, and after blotting as a control for RNA transfer. RNA blots were prehybridized, hybridized with excess [32P]-labeled probe (the 0.98 Kb rat INSR specific EcoRI fragment of the p16 clone, gift from Prof. Goldstein), washed under stringent conditions and finally autoradiographed. The autoradiographs were scanned with a laser densitometer and the readings normalized with the respective amounts of 28 S rRNA, as revealed by ethidium bromide.
2.4. Computer Analysis of DNA and RNA Sequences
SEQFIND is a program developed in our laboratory and initially utilized by our group in the computer search of AUUUA pentamers and U-rich domains in the 3’-untranslated region (UTR) of the human INSR pre-mRNA [22]. SEQFIND was also employed by our group in the identification of various DNA regulatory sequences in the human INSR gene promoter including two estrogen response elements [23], one VDRE [8], and various signal transducers and activators of transcription response elements [24].
Recently, we employed SEQFIND in the computer search of virtual VDREs in the rat INSR promoter, using the Rattus norvegicus partial INSR gene sequence (GenBank: AJ006071.1) [12]. In the present study, we carried out a detailed analysis of the characteristics of these VDREs in this promoter, using homology with the consensus VDRE (5’PuGGTCANNPuPuGTTCA3’), proposed by Colnot et al. [25] and Haussler et al. [3], and with the consensus VDRE (5’GGGTCANNGGGGGCA3’), selected by us from a series of described functional VDREs, in various 1,25D3-stimulated promoters [8].
In the present study, we also used SEQFIND in the computer inspection of the 5’-UTR and the 3’-UTR of the pre-mRNA encoding rat INSR (GenBank: NM- 017071.2), looking for regulatory cis-elements that could control mRNA translation and stability respectively.
2.5. Statistical Analysis
Unless otherwise indicated, the data are expressed as the mean ± S.E.M. A comparison between the groups was carried out using the two-tailed, unpaired Student t-test and/or ANOVA comparison, as appropriate. Differences were considered statistically significant when p < 0.05.
3. RESULTS
3.1. Body Weight and Plasma Values of Glucose, Insulin, 25-Hydroxyvitamin D3, Calcium, Phosphorus and Proteins
Treatment with 1,25D3 to non-diabetic rats increased body weight but did not affect the values of any parameter measured in the plasma of these animals including glucose, insulin and 25-hydroxyvitamin D3 (Table 1).
The induction of diabetes by STZ reduced body weight and induced hyperglycemia and hypoinsulinemia. Treatment with 1,25D3 to STZ-induced diabetic rats increased body weight without altering the hyperglycemia and the hypoinsulinemia induced by the diabetes, but increasing calcium and phosphorus plasma levels (Table 1).
3.2. Total DNA, RNA and Protein Content of the Hind Limb Muscle and Heart
Treatment with 1,25D3 increased the protein content and the indicator of cell size (protein/DNA) in both the hind limb muscle and heart of non-diabetic rats while the DNA and RNA contents remained unaltered (Table 2). STZ injection decreased RNA and protein content in hind limb muscle but increased both parameters and also the DNA content in the heart of these animals. Treatment with 1,25D3 to STZ-induced diabetic rats decreased even more the low values of RNA provoked by the diabetes in the hind limb muscle, but did not modify the high values of DNA, RNA and proteins induced by the diabetes in the heart (Table 2).
3.3. Insulin Receptor mRNA Levels in the Hind Limb Muscle and Heart
Northern blot assays of hind limb muscle (Figure 1) and heart (Figure 2) from non-diabetic rats revealed two major INSR mRNA species of approximately 9.5 and 7.5 Kb in both tissues. Since there is only one rat INSR gene, it has been suggested that the variation in transcript length may be due to the existence of alternative polyadenylation sites [26]. The relative amounts of these two INSR mRNA species measured as 9.5 Kb/7.5 Kb ratio were: 1.1 ± 0.1 in hind limb muscle and 1.3 ± 0.1 in the heart. These ratios are similar to those measured in adipose tissue [12,21] but lower than those detected in kidney [12]. Treatment with 1,25D3 to non-diabetic rats did not affect any of the two INSR mRNA species in any of
Table 1. Body weight and plasma values of the four groups of rats under study.

Non-diabetic rats (non-diabetic), rats treated with 1,25D3 (150 IU/kg [3.75 µg/kg] once a day, for 15 days) (1,25D3), streptozotocin-induced diabetic rats (STZ) and STZ-induced diabetic rats treated with 1,25D3 (STZ + 1,25D3). Values are the mean ± S.E.M. of 7 - 14 determinations in each group. ap < 0.05 vs non-diabetic rats; bp < 0.05 vs 1,25D3-rats; cp < 0.05 vs STZ-rats.
Table 2. Total DNA, RNA and protein content in the hind limb muscle and heart of the four groups of rats under study.
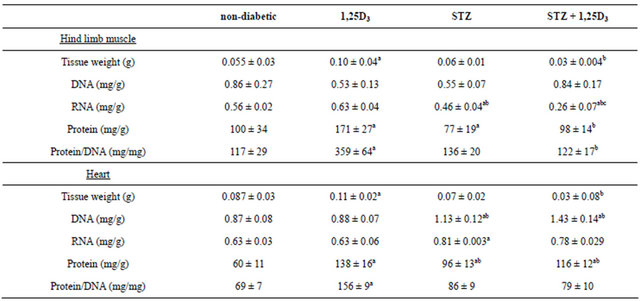
Non-diabetic rats (non-diabetic), rats treated with 1,25D3 (150 IU/kg [3.75 µg/kg] once a day, for 15 days) (1,25D3), streptozotocin-induced diabetic rats (STZ) and STZ-induced diabetic rats treated with 1,25D3 (STZ + 1,25D3). Values are the mean ± S.E.M. of 7 - 14 determinations in each group. ap< 0.05 vs non-diabetic rats; bp< 0.05 vs 1,25D3-rats; cp< 0.05 vs STZ-rats.
the tissues studied (Figure 1) and (Figure 2). INSR mRNA species were expressed per unit of DNA and per unit of RNA in view of the observation (Table 2) that although treatment with 1,25D3 to non-diabetic rats did not alter DNA or RNA content per gram of tissue in any of these tissues, the same treatment in diabetic rats decreased even more the RNA content in the hind limb muscle without altering the high DNA and RNA content in the heart.
The induction of diabetes by STZ produced important increments in the levels of both INSR mRNA species in both tissues. In particular, 174% increase corresponding to the 9.5 Kb and 280% to the 7.5 Kb species in the hind limb muscle (Figure 1) when expressed per unit of DNA and 113% and 195% increases of the respective species, per unit of RNA. In the heart, 57% increase corresponding to the 9.5 Kb and 87% to the 7.5 Kb species only when expressed per unit of RNA (Figure 2).
Treatment with 1,25D3 to STZ-induced diabetic rats corrected the over-expression of the INSR mRNA in the hind limb muscle (Figure 1) and heart (Figure 2) of these animals. Both the 9.5 and 7.5 Kb species were decreased in the hind limb muscle only when expressed per unit of DNA and the 9.5 Kb species in the heart only when expressed per unit of RNA.
3.4. Computer Analysis of VDREs in the Rat INSR Gene Promoter
In a previous computer search of virtual VDREs in the rat INSR gene promoter, we identified two candidates DNA sequences containing VDREs [12]. One DNA sequence, located between −256 and −219 bp of the rat INSR gene promoter with two superposed VDREs (the −247/−233 bp and the −249/−235 bp) overlapped by three AP-2-likes sites, and the other DNA sequence extending from −653 and −620 bp of this promoter with one VDRE (−637/−623 bp) and four overlapped AP-2- like sites. These VDREs separately or together could form a locus that may respond to 1,25D3 via VDR.
In the present work, we analyzed these virtual VDREs in order to find the best candidate for a repression response. We observed that the −247/−233 bp VDRE (5’GTGACCCGGGGTTGA3’) showed a difference of four bases with the consensus VDRE of Colnot et al. [25] and Haussler et al. [3] and of six bases with our consensus VDRE [8] (Figure 3). The −249/−235 bp VDRE (5’GGGTGACCCGGGGTT3’) showed differences of six and four bases respectively with these consensus (Figure 3). Whereas the −637/−623 bp VDRE (5’CGGGCAAAGGGGCGA3’) showed differences of five and four bases with the respective VDRE consensus (Figure 3).
Besides, the presence in the −247/−233 bp VDRE of a (T) at the (+4) position of its 3’ half-site provides the higher affinity of this site for the VDR [25]. The existence in the three VDREs of a (G) in the (−5) position of the 5’ half-site provides the consensus binding for RXR [3,25]. In addition, the presence in these three VDREs of various guanines in their two half-sites may help establish contacts with the VDR [25] (Figure 3).
We focused our attention especially in the presence in
(a)
(b)
Figure 1. Insulin receptor (INSR) mRNA levels in the hind limb muscle of the four groups of rats in study: nondiabetic rats (non-diabetic), rats treated with 1,25D3 (150 IU/Kg [3.75 µg/Kg] once a day, for 15 days) (1,25D3), streptozotocin-induced diabetic rats (STZ) and STZ-induced diabetic rats treated with 1,25D3 (STZ + 1,25D3). (a) Autoradiograph of a representative Northern blot experiment in the hind limb muscle of two rats from each group. The sizes of the two major INSR mRNA species are shown in the margin. The respective amounts of 28 S rRNA revealed by ethidium bromide are also indicated; (b) Densitometric analysis of three independent Northern blot experiments. INSR mRNA species were quantified separately, normalized with the respective 28 S rRNA values and expressed (per unit of DNA and per unit of RNA) as a percentage of the values obtained for non-diabetic rats (mean ± S.E.M., ap < 0.05 vs non-diabetic rats; bp < 0.05 vs 1,25D3-rats; and cp < 0.05 vs STZ-rats).
both the −247/−233 bp VDRE (5’GTGACCCGGGGTTGA3’) and the −637/−623 bp VDRE (5’CGGGCAAAGGGGCGA3’) of a purine (A) at the (+7) position of both 3’ half-sites, which allows positive control by 1,25D3 [3,27]. Contrarily, we observed the presence in the −249/−235 bp VDRE (5’GGGTGACCCGGGGTT3’) of a pyrimidine (T) in the same (+7) position, which allows negative control by 1,25D3 [28] (Figure 3). Therefore, only the −249/−235 VDRE could mediate a response of repression like that observed in the present work in the attenuation of the over-expression of the INSR gene caused by the treatment with 1,25D3 in the hind limb muscle and heart of di-
(a)
(b)
Figure 2. Insulin receptor (INSR) mRNA levels in the heart of the four groups of rats in study: non-diabetic rats (non-diabetic), rats treated with 1,25D3 (150 IU/Kg [3.75 µg/Kg] once a day, for 15 days) (1,25D3), streptozotocin-induced diabetic rats (STZ) and STZ-induced diabetic rats treated with 1,25D3 (STZ + 1,25D3). (a) Autoradiograph of a representative Northern blot experiment in the heart of two rats from each group. The sizes of the two major INSR mRNA species are shown in the margin. The respective amounts of 28 S rRNA revealed by ethidium bromide are also indicated; (b) Densitometric analysis of three independent Northern blot experiments. INSR mRNA species were quantified separately, normalized with the respective 28 S rRNA values and expressed (per unit of DNA and per unit of RNA) as a percentage of the values obtained for non-diabetic rats (mean ± S.E.M., ap < 0.05 vs non-diabetic rats; bp < 0.05 vs 1,25D3-rats; and cp < 0.05 vs STZ-rats).
abetic rats.
3.5. Computer Analysis of the 5’ and 3’-UTR Regions of the Pre-mRNA Encoding Rat INSR
Eukaryotic mRNAs possess diverse regulatory cis-elements that control, among others, mRNA processing, stability and translational processes. Translation is most commonly initiated by a cap-dependent mechanism but some mRNAs from receptors, oncogenes and tumor suppressors that show inconsistence between their mRNA
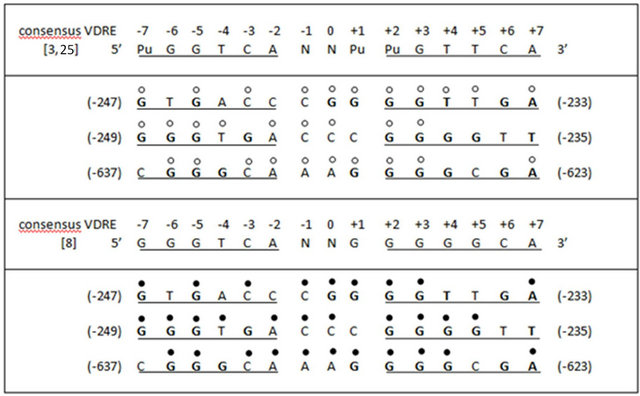
Figure 3. Virtual vitamin D response elements (VDREs) localized in the rat insulin receptor (INSR) gene promoter. Homology of the bases with the consensus VDRE proposed by Colnot et al. [25] and Haussler et al. [3], and with the consensus VDRE selected by our group [8], are indicated labelling the nucleotides with empty and filled circles, respectively.
and protein levels contain IRESs (internal ribosome entry sites) [29]. Here, we observed by computational analysis using SEQFIND that the 5’-UTR region of the precursor mRNA encoding rat INSR contains a virtual IRES with up to seven CCU motifs in a region between −295 to −73 nt upstream of the initiator AUG (Table 3).
We also performed a computational analysis of the 3’-UTR region of this precursor mRNA of the rat INSR looking for destabilizating elements [29] that could be implicated in our observed reduction of IR mRNA species provoked by 1,25D3 in STZ-induced diabetic rats. The results in Table 3 reveal the presence of one (AUUUA) pentamer, three (AUUUUA) hexamers, and the presence of various U-rich domains: six with five U (no identified in the table), one with seven U and one with ten U. This high number of destabilizing elements is higher than that detected in the 3’-UTRs of other mRNAs considered as highly regulated mRNAs [22]. We hypothesize that the 9.5 Kb INSR species would possess all these destabilizing elements and be more affected than the 7.5 Kb INSR species.
4. DISCUSSION
4.1. Effects of Treatment with 1,25D3 to Non-Diabetic Rats
The no alteration of any parameter measured in the plasma of these animals after treatment with 1,25D3 is consistent with similar results previously reported by us [12], and others [11] in equivalent rat models. A counter regulatory mechanism in the rat similar to that demon
Table 3. Computer identification of virtual cis-regulatory sequences in the 5’ and 3’ untranslated regions (UTRs) of the premRNA encoding rat insulin receptor (GenBank: NM017071.2).

Number in 5’-UTR indicates the distance upstream of the initiator AUG. Number in 3’-UTR indicates the distance downstream of the initiator AUG.
strated in humans [30], could maintain 25-hydroxyvita min D3 plasma levels within the observed range of 15 - 19 ng/ml with vitamin D supplies of 150 IU daily administered by injection plus the vitamin D consumed in the diet. In addition, in the same experimental model, we previously demonstrated the no affectation by the treatment with 1,25D3 of any urine parameter such as specific gravity, pH, leukocytes, nitrite, protein, glucose, ketones, urobilinogen, bilirubin and blood [12].
The increments in protein content and the indicator of cell size (protein/DNA) induced by 1,25D3 might indicate hypertrophy of both tissues. This is supported by the fact that 1,25D3 besides increasing body weight also increased the weights of both tissues. This in vivo hypertrophy induced by 1,25D3 is in agreement with results showing increased muscle fibre number and diameter in elderly women treated with 1,25D3 [31], with indirect results indicating smaller fibre sizes in various muscle types of VDR-null mice [32], and with a possible role for this vitamin as a muscular-protective agent [33,34]. In other tissues such as liver, kidney and adipose tissue, the induction of hypertrophy by 1,25D3 is not so clear [12].
The presence of two INSR mRNA species of 9.5 and 7.5 Kb in the hind limb muscle of non-diabetic rats is consistent with earlier studies from our group [12,21] and others [35,36] showing species of comparable size in this same tissue. Regarding the presence of these two INSR mRNA species in the heart, to our knowledge this is the first description. The treatment with 1,25D3 did not alter the expression of these INSR mRNA species in any of these two tissues in study. We had previously reported species of similar size and their no alteration by the treatment with 1,25D3 in the kidney, liver, and adipose tissue of non-diabetic rats. This was accompanied by the no affectation of INSR number and the insulin response to glucose transport in isolated adipocytes of these animals [12]. Other authors observed increased INSR mRNA expression (measured by RT-PCR) in the gastrocnemius muscle of rats fed a diet containing whey protein, high calcium and high vitamin D intake (10,000 IU/kg for 13 weeks), but not when the rats were exposed at a dose (400 IU/Kg for 13 weeks) closer to the one used in our experiments [37].
The lack of effects of 1,25D3 on INSR gene expression in the hind limb muscle and heart of non-diabetic rats could be also related to the absence of VDR in both tissues and/or to the absence of regulation of VDR by its ligand in these tissues. In the first case, recently Wang and DeLuca [38] did not find VDR expression in skeletal, heart and smooth muscle extracts from mouse and human (measured by western blot and immunohistochemistry) in contradiction with previous studies in chick myoblasts [17,18] and in mouse and human extracts [32,39]. The different specificity of the VDR antibodies used in these works could account, at least in part, for these results. In the second case, Bischoff et al. [39] indicated that VDR expression correlated with age but not with 25-hydroxyvitamin D3 or 1,25D3 levels in skeletal muscle, whereas Chem et al. [40] showed that VDR has a ligand-independent activity in myocytes. Moreover, both tissues express the 1a-hydroxylase gene, reflecting the possible importance of the endogenously synthesized 1,25D3 [40,41].
4.2. Effects of Treatment with 1,25D3 to STZ-Induced Diabetic Rats
STZ-induced diabetes is an animal model of diabetes that comprises both toxic and inflammatory mechanisms [42]. Mononuclear infiltration, altered morphology of islets and disappearance of beta cells are the most reported histological changes in the pancreas of these animals [43]. Using this diabetic model, we previously reported glycosuria and the appearance of leukocytes, ketones and blood in the urine of these animals [12]. The presence of hyperglycemia and hypoinsulinemia has also been described [12,44,45]. We also detected normal levels of 25-hydroxyvitamin D3 in accordance with other authors [46]. Treatment with 1,25D3 increased rat body weight but did not revert the hyperglycemia and hypoinsulinemia induced by the diabetes. The 25-hydroxyvitamin D3 levels were maintained unaltered by 1,25D3. However, we observed increased calcium and phosphorus plasma levels after 1,25D3 treatment. It is well recognized the essential role of 1,25D3 in the homeostasis of calcium and phosphorus in muscle tissues [47]. Moreover, calcium per se is important for insulin secretion, as well as for correction of glucose intolerance [48,49]. Both parameters had been described as regulators of VDR in some tissues including the skeletal muscle [50,51].
The diabetes induced by STZ decreased RNA and protein content in the hind limb muscle accordingly with other authors [52]. Moreover, it is know that the hypoinsulinemia of the diabetes accelerates the proteolysis provoking muscle atrophy [53]. Regarding the heart, various authors had reported hypertrophy induced by the diabetes [54,55] and our present results also suggest hypertrophy with increased values of DNA, RNA and proteins but without changes in the weight of tissue and in the protein/DNA ratio. The treatment with 1,25D3 decreased even more the low values of RNA provoked by the diabetes in the hind limb muscle, but at the same time, 1,25D3 maintained the high values of DNA, RNA and proteins induced by the diabetes in the heart. A similar situation of permanence of high levels of proteins and DNA accompanied by slight increases of the protein/DNA ratio was observed by our group in the kidney and liver, but not in the adipose tissue of STZ-diabetic rats treated with 1,25D3 [12].
The diabetes induced by STZ also produced important increments in the levels of the 9.5 and 7.5 Kb INSR mRNA species in the hind limb muscle and heart of these animals. To our knowledge, this is the first description of such increments in these tissues of diabetic rats. Studying other tissues, our group reported increased levels of these INSR mRNA species in the liver and adipose tissue but not in the kidney of STZ-induced diabetic rats [12]. In addition, other investigators described increased INSR mRNA levels in the liver and kidney but not in the brain of STZdiabetic rats [56,57]. These results at the RNA level coexist with controversial results at the protein level in both the hind limb muscle [58,59] and the heart [60,61] of STZ-induced diabetic rats. Despite these differences, STZ diabetic rats characteristically display insulin resistance in both tissues [15,62,63]. Noteworthy, hind limb muscle contributes more to peripheral glucose utilization than the heart [15].
The treatment with 1,25D3 corrected the over-expression of the INSR mRNA induced by the diabetes in both tissues. These results represent the first demonstration of an in vivo regulation by 1,25D3 of INSR mRNA levels in the hind limb muscle and heart of diabetic rats. A similar prevention of the over-expression of INSR mRNA had been also observed by our group in the liver and adipose tissue but not in the kidney of STZ-induced diabetic rats treated with 1,25D3 [12]. In the case of adipose tissue, it was accompanied by the almost normalization of the number of INSRs and the improvement of both basal and insulin-stimulated glucose transport [12]. Although we did not analyze the insulin response of both tissues after the treatment with 1,25D3, the in vitro data of Zou et al. [64] indicating that 1,25D3 improved the free fattyacid-induced inhibition of glucose uptake in cultured C2C12 muscle cells, would support the possibility that 1,25D3 could prevent insulin resistance in muscle cells. These results were accompanied by the stimulation of various parameters of the insulin signalling, such as the IRS-1 and AKT [64]. Moreover, in the STZ-induced diabetic model, the in vivo administration of 1,25D3 for 8 weeks was reported to improve diabetes, attenuating pancreatic islet damage and decreasing the insulin requirements [42].
4.3. Possible Participation of Transcriptional and/or Posttranscriptional Mechanisms of Regulation in These in Vivo 1,25D3-Mediated Processes
In support of a possible transcriptional regulation by 1,25D3 correcting the over-expression of the INSR at the RNA level, in the hind limb muscle and heart of STZ-induced diabetic rats, we postulated the −249/−235 bp VDRE (5’GGGTGACCCGGGGTT3’) with a pyrimidine (T) in the (+7) position which allows negative control by 1,25D3 [28], as the best candidate for such a response of repression.
Posttranscriptional mechanisms of regulation at the level of mRNA translation could be acting in these in vivo 1,25D3-mediated processes. Only recently, the regulation of IR expression at the level of mRNA translation has been reported with the finding of a functional IRES in the human INSR mRNA [65]. In the present work, by computational analysis of the 5’-UTR region of the precursor mRNA encoding rat INSR, we detected a virtual IRES with up to seven CCU motifs in a region between −295 to −73 nt upstream of the initiator AUG. These data represent the first identification of an IRES in the rat INSR pre-mRNA. The possible function of this virtual IRES in maintaining INSR protein expression and its regulation by 1,25D3 needs more investigation.
Finally, variation in INSR mRNA stability is a posttranscriptional mechanism considered as an important regulator of INSR expression [66]. Our group previously demonstrated a high presence of destabilizing elements in the 3’-UTR region of the human INSR mRNA favouring its consideration as a highly regulated mRNA [22]. In the present work, using computational analysis of the 3’-UTR region of the precursor mRNA, we detected for the first time the presence of a high number of AU-rich elements and U-domains. Stability of the INSR mRNA could be highly regulated through these destabilizing elements. The possible implication of these facts in the observed correction by 1,25D3 of the over-expression of INSR mRNA species in the hind limb muscle and heart of STZ-induced diabetic rats remains the object of further research.
In conclusion, the present data suggest certain beneficial effects of 1,25D3 in the amelioration of the diabetes, which could be mediated by the control of INSR expression via transcriptional and/or posttranscriptional mechanisms. In agreement with Girgis et al. [67] the potential benefits of 1,25D3 are more pronounced in situations of vitamin D deficiency or poor glycemic control, as is the case of our STZ-induced diabetic rats.
5. ACKNOWLEDGEMENTS
This work was supported by research Funds from the Ministerio de Ciencia e Innovación (SAF2009-12671).