Zero-Order Release Profiles from A Multistimuli Responsive Electro-Conductive Hydrogel ()
1. Introduction
Conductive electroactive polymers (CEPs) [1,2] and hydrogels [3-5] are two of the most promising class of materials for biomedical applications The former ones, because its electrically modulated properties, could be engineered for devices like biosensors [6-9], drug delivery systems [10-12] and substrates for neural prostheses [13- 16]. The second ones, due its transport properties, high hydration levels and biocompatibility are extensively found in controlled delivery devices [17], biosensors, contact lenses, catheters, wound dresses and tourniquets [5]. In 1995, Guiseppi-Elie [18] described the synthesis of electroconductive hydrogels (ECHs), materials recognized as composites or blends made by the union of conducting polymers with hydrogels, resulting in a unique polymeric material that combines the special properties of their constituents: high swell ability in water, in vitro and in vivo biocompatibility and the high diffusivity of small molecules from hydrogels and high electrical conductivity, ON-OFF electrical and optical switching, electrochemical triggered redox properties and volume changes. Later, Wallace et al. reported the synthesis and application of a polyacrylamide/polypyrrole composite for controlled release applications [19]. Since the early works of Guiseppi-Eli and Wallace, ECH have been the subject of growing attention and today there are several works that shows the synthesis and application of ECHs in implantable devices (biosensors, controlled drug delivery, tissue engineering) and most of them involve conducting polymers such as polyaniline-PANI, polypyrrole-PPy and poly(3,4-ethylenedioxythiophene)—PEDOT and hydrogels networks (poly(vinyl alcohol)—PVA, polyacrylamide—PAAm, poly(hydroxyl ethyl methacrylate)—PHEMA, poly(acrylic acid)-PAA, poly(lactic-co-glycolic acid) —PLGA, etc) [20,21]. A number of routes have been proposed for synthesizing these ECHs, and they can be summarized into three categories, based on their fabrication route [20]: 1) Templated conducting polymer—hydrogel; 2) Conducting polymer deposited within a hydrogel matrix; 3) Conducting polymer—hydrogels formed from mixed precursors—either simultaneously or in a two-step process. The most common synthetic method is the polymerization (chemical or electrochemical) of the conducting polymer in a preformed matrix (category 2). As an example, our group has demonstrated the synthesis of a polyaniline-polyacrylamide composite by electropolymerization of conducting polymer inside an insulating hydrogel matrix of different pore sizes. This resulting new material was successfully applied in electrochemical controlled release devices [22-24]. A number of examples of synthesis and application of ECHs can be found in a recent review on this topic [20,21]. Ocusert™ system introduced by Alza Corporation in 1975 can be cited considering its technical and historical importance; it was the first topic formulation of controlled release for ocular use. The system, that is commercialized even today, is a reservoir type and releases pilocarpine trough an ethylene vinyl acetate copolymer membrane with a zero-order kinetics that provides a constant release of the drug over the time [25]. This special regime of release is often desired in order to obtain the needed therapeutic action and to avoid side effects. The problem is that most of controlled delivery devices based on ECH display other kind of release kinetics, typically a first order one, which limits its application. Usually, the structural characteristics of ECH, determined by the synthetic conditions, govern the release pattern of a determinate solute; and, environmental parameters such as temperature, pH and ionic strength only modify the release rate but not the mechanism that is inherent to the ECH. Our work presents the synthesis and characterization of an electroconductive hydrogel based on the electropolymerization of pyrrole in a poly(acrylic acid) hydrogel (named as polypyrrolepoly(acrylic acid) composite). This type of ECH posses a multistimuli responsive behavior: the anionic hydrogel matrix ensures a great swelling change driven by changes in pH and ionic strength of the release media while the conducting polymer provides the electrochemical switch capability based on changes in the polypyrrole charge and volume depending on its oxidation state. An unexpected synergetic effect between the hydrogel and the conducting polymer that causes an increase of the degree of swelling of the entire system when the pH is raised up to 13, is observed. More remarkable, the existence of a combination of pH and electrical potential applied to the polypyrrole-poly(acrylic acid) composite allows the release of safranin in a linear (constant) fashion. For the best of our knowledge, this work describes for the first time, a multistimuli responsive ECH that can display different release patterns (based on Fick’s Law), i.e., zeroorder or other mechanisms only as a function of external parameters.
2. Experimental
2.1. Poly(Acrylic Acid) Hydrogel Synthesis
Hydrogel was obtained by free radical polymerization of acrylic acid. The monomer and the crosslinking agent N, N’-methylene bis-acrylamide were dissolved and the solution was degassed for 10 minutes at room temperature. Then, potassium peroxydissulfate and N,N,N’,N’-tetramethylethylenediamine were added and they acted as oxidizer and catalyst, respectively. The moisture was placed in a cylindric plastic tube to obtain a cylindric hydrogel. After being removed from the tube, the hydrogels were immersed in water that was replaced periodically to remove unreacted substances.
2.2. Pyrrole Polymerization on Poly(Acrylic Acid) Hydrogel
The conducting polymer was electrochemically polymerized into poly(acrylic acid) hydrogel matrices. A constant potential of +0.6 V was applied until de charge achieves a value of 10.5 C. A platinum wire was inserted on hydrogel and was used as the working electrode. The reference and counter electrodes used were, respectively, Ag/AgCl (KCl(sat)) and a platinum sheet. The polymerization solution was pyrrole (0.4 mol·L–1) dissolved in sodium nitrate solution 1.0 mol·L–1. The composite obtained (polypyrrole-poly(acrylic acid) composite) is nemed as PPy-AA in the whole manuscript. Before the monomer polymerization, the swollen AA was sliced in small pieces and immersed in 1.0 mol·L–1 sodium nitrate solution for 24 hours. Pyrrole monomer was distilled using fractional distillation method prior to use. All the experiments were carried out with a potentiostat/galvanostat Autolab PSTAT 30.
2.3. Swelling Degree (Q)
Pieces of known mass of dried AA and PPy-AA hydrogels were placed in water or in sodium nitrate solutions of different concentration at room temperature. Periodically, hydrogels were carefully wiped with a filter paper and weighted. The swelling degree was calculated applying the Equation (1):
(1)
where Q is the swelling degree and W0 and Wt are hydrogel mass at the dried state and at different times t.
2.4. Cyclic Voltametry
The electroactivity of PPy-AA and AA were determined by cyclic voltammetry. In this case, the working electrodes were the hydrogel with and without polypyrrole and the counter and reference electrode were the same used on conducting polymer polymerization. Prior this characterization, the AA and PPy-AA were put in sodium nitrate solution 1.0 mol·L–1 for 24 hours, and the cyclic voltammetry experiemts were performed in this solution.
2.5. Safranin Loading and Delivery
AA and PPy-AA hydrogels were immersed in a safranin solution (0.01 mol·L–1) for 5 hours and after this time, they were used as working in the potentiostatic controlled release experiments. The counter and reference were the same used at pyrrole polymerization in poly(acrylic acid) hydrogel. The hydrogels/composites were washed with distillated water in order to remove the excess of safranin and then placed on sodium nitrate solution on pH 6.4 or 3.8 and the potential was applied. The solution was sampled at regular times and the amount of released safranin was determined by UVVIS spectrophotometry by using a Hewlett Packard 8453 diode array spectrophotometer at a fixed wavelength of 519 nm.
3. Results and Discussion
The chronoamperogram for the pyrrole polymerization is ilustred it Figure 1. It can be observed that the current increases with time indicating that an electroactive material is being formed. This is because the pyrrole polymerization stars at the platinum wire and after be covered totally, the polymerization branches on the hydrogel structure until that the conducting polymer fills all the material. This process can be observed on photos inserted in the graphic that was obtained during the polymerization. The hydrogel’s ability to absorb water and biological fluids is related to the flexibility and the hydrophilic nature of the polymeric network. When the hydrogel is immersed in aqueous medium, water and others molecules diffuse through the pores due to their interaction with the functional groups of the polymer.
The elasticity of the hydrogel is related to the degree of crosslinking and, in this specific case, to the presence of the conducting polymer. Figure 2 shows the swelling degree measurements performed for AA and PPy-AA in

Figure 1. i/t profile recorded during pyrrole polymerization inside the polyacrylic acid matrix. Electrolytic solution: 0.4 mol·L–1 pyrrole +0.1 mol·L–1 NaNO3. Eapp: +0.6 V. Inserts: photos obtained during the conducting polymer polymerization.
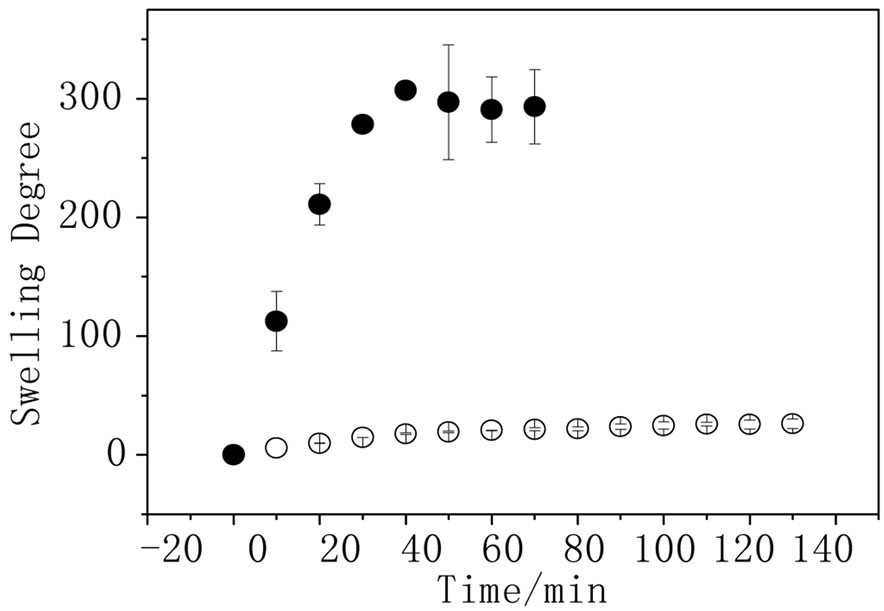
Figure 2. Swelling degree as function of time for ● AA and ○ PPy-AA in water (n = 3).
water. It can be observed that the presence of the polypyrrole decreases the water absorption from 300 to 25; this result is expected due to the fact that the conducting polymer was polymerized inside the hydrogel pores and the entanglement of the polypyrrole chains in AA would reduce the material elasticity and the swelling degree. Figure 3(a) shows the hydrogel swelling degree dependence on time. All the measurements were done at the same pH (6.4) but in sodium nitrate solutions of different concentrations. The hydrogel swelling decreases with the increase of sodium nitrate concentration, as expected in agreement with the thermodynamics basis of the equilibrium swelling theory that predicts that osmotic swelling pressure decreases as the ionic strength in the solution outside the hydrogel increases. As the osmotic pressure decreases, the Gibbs’s free energy of swelling also decreases and, consequently, the degree of swelling goes in the same direction [3]. The same outline was observed for the hydrogel filled with polypyrrole (Figure 3 (b)). For this experiment, the concentration of the sodium nitrate solution was less than those used for the hydrogel without conducting polymer because for concentrations above 0.25 mol·L–1 , the swelling degree was unaltered; so that, no differences of the swelling degree could be analyzed. For the achievement of a dual response system, the PPy-AA must keep the capability of changing the swelling degree due to changes in the pH. In order to verify this behavior, swelling experiments were carried out in pHs 3.8, 6.4 and 13.0 but keeping the concentration of sodium nitrate solution constant at 0.25 mol·L–1.

Figure 3. Swelling degree as function of time for (a) AA in NaNO3 ◄ 0.25, ● 0.5, ▲ 1.0, ▼2.0, ►4.0 mol·L–1; (b) PPyAA in NaNO3 ■ 0.125, ▲ 0.25, ● 0.5 mol·L–1 (n = 3).
Figure 4 depicts the swelling behavior of both the hydrogel and the composite in different pHs. As it can be observed, when AA is immersed in pH higher than 4.2, the pKa of poly(acrylic acid), carboxylate groups are formed and to neutralize the charge, water and protons penetrate the hydrogel. So, the swelling degree was higher for pHs 13 and 6.4 than for 3.8. The swelling behavior of PPy-AA (Figure 4(b)) was also higher for pH 13 than for 3.8 and 6.4, these two last ones being almost equal and lower than the values obtained for the AA hydrogel. Here, a striking feature must be pointed out related to the fact the swelling of the PPy-AA composite at pH 13 is practically twice compared to that observed for the AA hydrogel in the same pH. The pKa of PPy was measured to be 8.6 [26], above this value an electrostatic repulsion between the polyacrylate and polypyrrole chains is expected due to the unpaired electrons on the nitrogens, since the polypyrrole is deprotonated. That is to say, that the interaction between the hydrogel and the conducting polypyrrole chains will be weaker. Moreover, conformational changes of the conducting polymer are expected leading to more linear chains due the presence of the polypyrrole’s unbounded amine electrons and these two factors together, result in a more open pore structure and , consequently, to a higher swelling degree as depicted in the scheme shown in Figure 5. Only at pH below 8.6, an effective interaction among the two components of the polymeric system is present, and because of this interaction, the conducting polymer chains are completely spread into the hydrogel matrix, leading to a state of mini-