Study of Physical, Chemical and Morphological Alterations of Smectite Clay upon Activation and Functionalization via the Acid Treatment ()
1. Introduction
Montmorillonite is one of the clay materials widely used for a large number of applications. This abundant (plentiful) raw material is highly valued for its reactive and sorptive properties, which depend strongly on its large surface area and its tendency to adsorb molecules at interlayer sites. One type of the clay from the smectite family is the montmorillonite (MMT) which was found for the first time at montmorillon in France and formed of very soft phillosilicates with a monoclinic crystal system [1]. MMT is derived from weathering of volcanic ash like other types of smectites. It is a 2:1 clay meaning that has two silica tetrahedral sheets and a central alumina octahedral sheet between them. Mineral acids like HCl and H2SO4 can solve and separate the alumina layer of the clay. Eisele (1982) introduced the bleaching of the clay by mineral acids as a new method for producing aluminum metal [2,3].
Several methods have been suggested in literature to improve the properties of clay materials which include modifying or changing the clay mineral composition in site organic material, the type and amount of exchangeable ions and soluble salts, laser enhanced ions, and texture of the clays’ nanostructural. We can improve specific surface area, pore volume, acidity, activated sites and adsorption capacity, exchangeable ion capacity, and other applicable characteristic of the clay materials [4-9]. The individual clay particles aggregate because clays are originated from aluminum, silicon and iron oxides. The theoretical surface area of the clay material reduces ten times. It is due to the nature and number of interlayer cations compensating to neutralize the tetrahedral silicon and octahedral aluminum substituted atoms in the 2:1 lattice of MMT. The acid treatment of the clay is a very simple and efficient method for modifying the clay surface area, reactivity, homogeneity and adsorption. The method can cause the elimination of mineral impurities and exchangeable metal cations. Changes in the chemical and physical properties of the clay using the acid treatment could impart some of its interesting and desirable characteristics.
Madejova and Tyagi used FT-IR spectroscopy for determining the structural modifications and Bronsted acid sites generated during the acid treatment of different clays with varied concentration of sulphuric acid [10,11]. Moreover, changes in Layer Charge (LC), Cation Exchange Capacity (CEC), and distribution functions of the adsorption energy sites on the solid surface were studied by Pentrak and Boudriche [12,13]. Kaolinite and montmorillonite activated by mineral acids are employed as adsorbents for removing metal ions like Cd(II), Co(II), Cu(II), Pb(II), and Ni(II) and compounds such as SO2 [14-18]. Furthermore, some theoretical studies have been undertaken on the Brønsted acid sites, generated during the acid treatment of the montmorillonite, and their bleaching capacity variation [19,20].
The objective of this work is to study the behavior of montmorillonite clay treated with sulphuric acid of different concentrations. Their composition, morphology, and crystallinity of these treated MMT samples were then studied by different instrumental techniques such as SEM, XRF, XRD, FTIR and TEM. The present research work was intended to investigate the physical and chemical characteristics of modified clay samples as an activated nanomaterial.
2. Experimental
2.1. Materials
The clay, Cloisite® Na+, was supplied by the southern Clay Products, Co. (USA). This natural montmorillonite clay was found to have a bulk density of 336 g/l and cation exchange capacity of 92.6 meq/100g with a medium particle size of 12 μm. Regarding the acid treatment of the clay, sulfuric acid (H2SO4 > 96% purity) was used, obtained from Merck Co. (Darmstadt, Germany).
2.2. Methods
Fifty grams of the clay was refluxed in 1000 ml of the prepared acid solution for 120 minutes at 80˚C - 85˚C in a flask equipped with a reflux condenser. To modify and activate the MMT, sulfuric acid solutions with varied concentrations, 1, 2, 3, 4, 8, and 10N (equiv/L) were used. The ratio of solid to liquid was kept constant at 1:20. Cold water was used for rapid quenching of the resulting MMT suspension. Because of poising effect of acid on the catalyst, a vacuum filtration system was employed for the separation of contents at first, and after that, three times filter cakes were washed using hot distilled water after every filtrations in order to remove any remaining acid. It was ground in an electrical mill to a powder form, after drying the modified MMT. After some thermal analyses, all of the powder samples were dried at 150˚C for two hours in the oven. The modified MMT samples were designated as MN1, MN2, …, and MN10, the suffixes indicate acid concentrations and MN0 refers to the untreated clay sample.
2.3. Apparatus and Methodology
Powder X-ray Philips-X’Pert model diffractometer (Netherlands) was employed to analyze the crystalline phase at low angle X-ray Diffraction (XRD). The XRD patterns were recorded in the range of 2˚ - 70˚ with a scanning rate of 0.02˚/s. Changes in the chemical compositions were studied by X-ray fluorescence (XRF) analyses of the samples using a Phillips PW 2400 Model (Netherlands), and Cu X-ray tube and scintillation detector working at the current of 30 mA and voltage of 40 KV. Fourier transform infrared spectroscopy was performed out using a Bruker Tensor 27 (Germany), in the wave range of 400 - 4000 cm−1 via the KBr disc technique. The samples were heated at the constant rate of 10˚C/min from 35˚C to 800˚C in a nitrogen atmosphere. Scanning electron micrographs were obtained using a Hitachi S-4160 Field Emission Scanning Electron Microscope (FESEM) (Japan). In addition, the morphologies of the specimens were also examined by Transmission Electron Microscopy (TEM) taken on a Philips CM120-120KV (Netherlands).
3. Results and Discussion
3.1. Chemical Structure Characterization
Infrared spectroscopy is one of the oldest and most common methods in mineral studies. For an Infrared (IR) analysis the KBr pressed disc technique is frequently used. Figure 1 shows the infrared spectrum of KBr thin discs prepared from the montmorillonite powders. The transmittance FTIR spectrum of the unmodified and various modified montmorillonite samples are shown together.
The clay transmittance bands area of vital importance in distinguishing the structural variation of the smectite clay activated using sulfuric acid. In Table 1, important IR bands of the montmorillonite are illustrated [10,11]. Absorption bands at 3616 cm−1 and 1630 cm−1 are assigned to the stretching and bending vibrations of OH groups in the crystal and adsorbed water molecules, respectively. The Infrared spectral data (peaks at 915, 880 and 840 cm−1) shows that the sulfuric acid first affected the octahedral sheet followed by the tetrahedral sheet. The peak seen at 1425−1 is due to some calcite impurity of the raw montmorillonite. The sign of hydroxyl groups, Al2OH, AlFeOH and AlMgOH, can be observed at 915, 880 and 840 cm−1, respectively. Because of the clay

Figure 1. IR spectra of unmodified (MN-0) and modified montmorillonite (MN-1, MN-2, MN-3, MN-4, and MN-8) at range of 300 - 4000 cm−1.
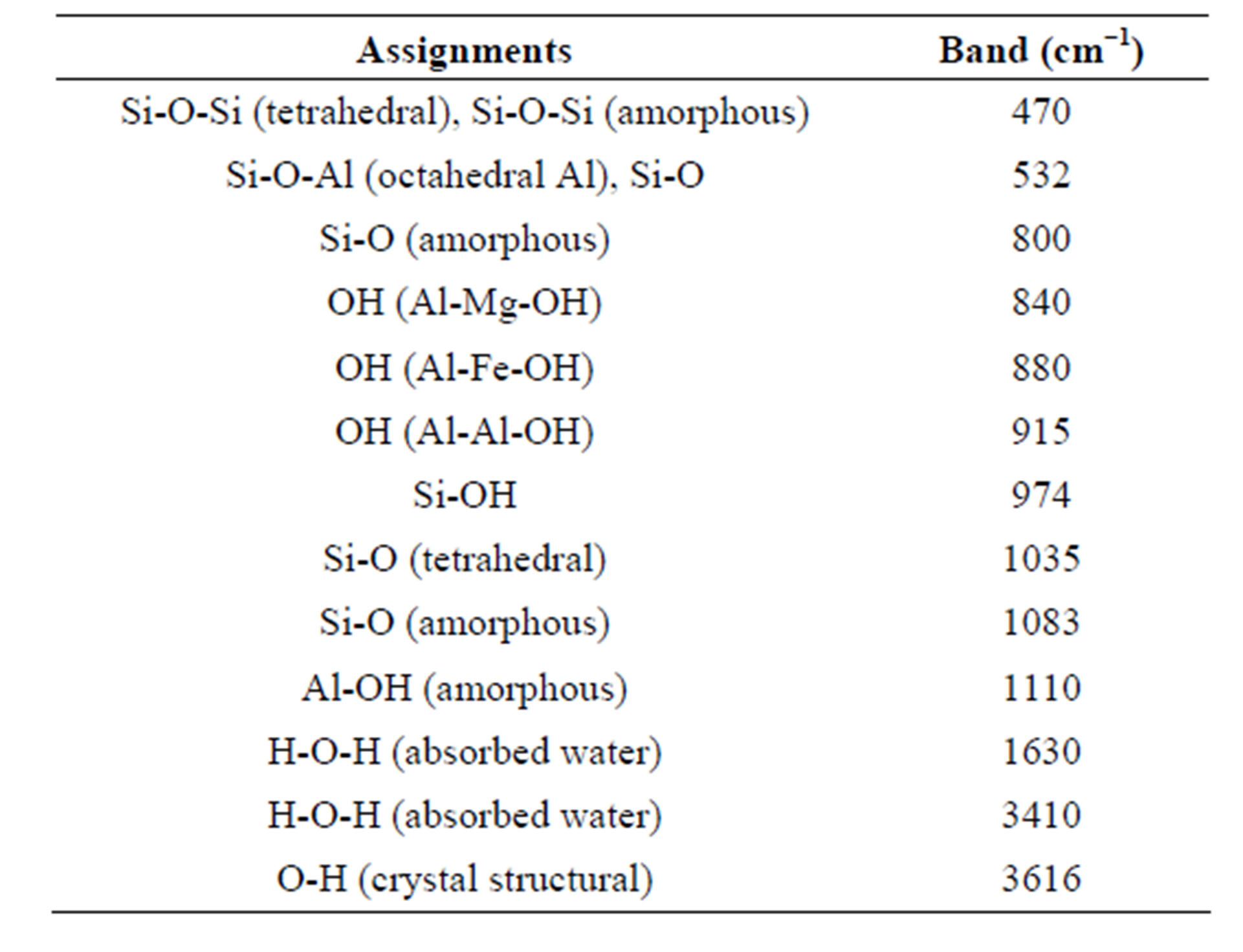
Table 1. Important IR bands of the smectite clay with their possible assignments.
chemical and acidic variation during the acid activation, Al, Mg and Fe are partially washed out from the clay structure and consequently their bands were weakened and to some extent disappeared. The original structure of the montmorillonite was not fully destroyed and it can be distinguished from the presence of a band at 532 cm−1, which is the most sensitive to the presence of residual Al in the octahedral layer.
The IR bands at 1035 and 532 cm−1, corresponding to the Si-0-Si stretchinbg and Si-0 deformation, show that the tetrahedral sheet of the clay was relatively stable. Although the tetrahedral layer of the clay is not affected by the acid treatment, the sign of structural deformation can be seen at a higher acid concentration. A broad band near 974 cm−1 corresponds to the vibrations of SiOH groups, which are present in the three dimensional product formed as the octahedral sheet in the smectite layer. However, in the MN-8 clay samples, the band at 532 cm−1 (Si-0 deformation) became a very weak shoulder. The free silica bands at 470 and 800 cm−1 showed increased intensity with an increasing acid concentration for samples higher than 3N.
In the interaction between clay components and a mineral acid, three different behaviors can be seen. Some substances and compounds would be physically dissolved in an acid solution, some of them react with the acid, and others did not interact with the acid. To distinguish the interactions between the clay and acid through the acid treatment, the X-ray fluorescence (XRF) was employed. Table 2 shows the results of the chemical analysis of the pristine and 120 min acid-treated montmorillonite clay obtained from the XRF (all values are represented as wt% on a dry weight basis). Alumina and silica was the major components of the montmorillonite whereas other oxides, such as magnesium oxide, sodium oxide, potassium oxide, ferric oxide and titanium oxide, was present in trace amounts.
Through the acid modification of the clay, the percentage of the chemical composition of the considered compounds varied. The SiO2 content increased by enhancing the acid strength, which is due to an increase in the Si/Al ratio resulting from the depletion of the Al cations from interlayers and octahedral sheets of the montmorillonite. Simultaneously, the content of Al2O3, MgO, and Na2O decreased progressively. A lower decrease in aluminum content at a lower acid concentration can be related to the shielding of aluminum cations by the silicon oxygen network and the presence of Al-O-Si bonds which results in low solubility at the low acid strength [21]. If a sufficiently high acid concentration is used, a pure silica phase will be created and the montmorillonite structure will be completely decomposed [22]. Hydrolysis of alumina in the octahedral layers of the montmorillonite caused Al3+ ions to be leached and the alumina content decreased in the acid-treated sample.
The exchange of the interlayer Na, Ca, Mg, Fe, and Li cations begins at first, and is followed by leaching of the octahedral cations (Al, Mg, Fe, and Li) through the montmorillonite dissolution. It should be, however, noted that the acidity of the montmorillonite clay arises from H+ in the surface exchange sites; the exchange of interlamellar cations with protons generates the Brønsted acid sites, and Lewis acid sites associated with Mg2+ and Al3+ are present at the edges of the octahedral sheets [23]. Although there is a progressive decrease in the aluminum, magnesium, and sodium content of the clay, the ferric oxide and titanium oxide increase from 4.67 to 7.22 and 0.13 to 0.21 mass%, respectively. Free silica generated at a higher acid concentration acted as a barrier and protected the sodium, ferric oxide, and titanium oxide from acid attack [24]. Because of substitution of Al3+ ions by lower valent ions, like Mg2+, in the octahedral sheet and Si4+ by Al3+ in the tetrahedral layer, a progressive decrease in cation exchange capacity (CEC) values would

Table 2. Chemical composition of pristine and modified montmorillonite obtained using XRF.
be expected for acid treated clays.
3.2. Physical Structure Analysis
To study the structural changes that occurred in the clay material due to the acid treatment, the X-ray diffraction technique was employed. Figure 2 illustrates the XRD profiles of the untreated and acid-treated smectite. This figure can help distinguish the transformation and change of the montmorillonite crystallites and structure. The intensities of almost all reflections decreased as the concentration of acid solution increases. The height and intensity of the peak in the XRD patterns (001) (2θ = 8/1˚, d = 6.35˚A) were evaluated using X-ray diffraction of the powdered samples.
As illustrated in Figure 3, the 001 peak intensity of the clay decreased progressively with the acid treatment. Structural disordering, which occurred due to the acid treatment, affected the montmorillonite crystalline character and content. The crystalline phase content and the basal spacing of the clay are indicated by the height and position of the 001 peak. As shown in Figures 2 and 3, the crystalline phase content of MMT decreased continuously by utilizing acid solution but there was no evidence of an interlayer distance change. The decrease of intensity can be distinguished for the MN-3 clay and higher clay treatments, the peak started broadening onward. The Increase of crystallite size and simultaneously decrease of the mean lattice strain with added acid cause narrowing of the peak. At an acid concentration higher than 2N, delaminating of the clay structure is suggested to begin.
At over a smaller range of 2θ, the clay sample prepared using a very high acid concentration (10N) has a very broad 001 peak, as it is illustrated in Figure 4. Broadened peaks of the samples reveal that the layer nature of these materials was disordered and, to some extent, amorphous like. This may be related to a large degree of structural disorderliness in these samples.
At same time, the peaks appeared at 16.4˚ (2θ = 16/4˚, d = 3.17˚A), 32.7˚ (2θ = 32/7˚, d = 1.57˚A), and 73.5˚ (2θ = 73/5˚, d = 0.75˚A), due to X-ray diffraction from the 002, 003, 004 planes respectively, were gradually broa-

Figure 2. XRD patterns of unmodified (MN-0) and modified montmorillonite (MN-1, MN-2, MN-3, MN-4, and MN-8).
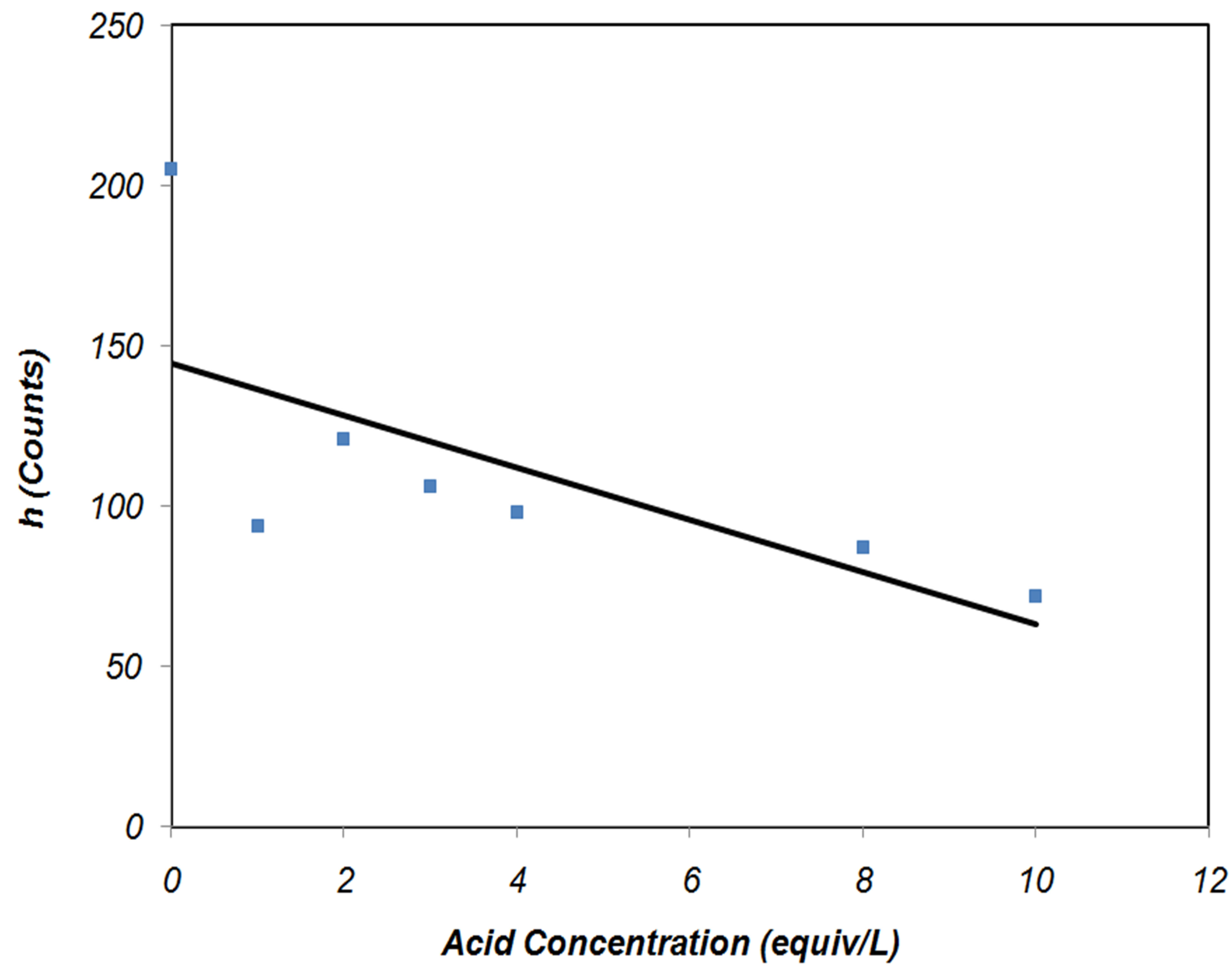
Figure 3. Intensity of the peak (001) in the XRD pattern of unmodified (MN-0) and modified montmorillonite (MN-1, MN-2, MN-3, MN-4, MN-8, and MN-10).
dened with acid treatment [25]. Presence of kaolinite was distinguished from its peaks at 23.9˚ and 41.2˚, yielding a good sequence of 001 and 002 reflections [26]. Peaks, attributed to Kaolinite, didn’t achieve any alteration by acid treatment, because of well-ordered crystal of the kaolinite and its 1:1 layers structure.

Figure 4. Higher resolution XRD pattern of modified montmorillonite MN-10 at initial position.
3.3. Morphological Study
Although there is too much publication about the acid treatment of clays but rarely there are found something about the morphological variation of clays through the acidic modification [27,21]. Changing of clay crystalline structural and its regularity was observed at figure 5 transmission electron micrographs. Figures 6(a) and (b) are TEM pictures of pristine and MMT sample modified by 8N Sulfuric acid solution (MN-8) consequently. Treatment of sample leaded to slightly amorphization of the clay particles. This amorphization is predominated at the end of layer silicates and edge of aggregates. Since in comparison with centeral octahedral cations, the silicate layer structure of MMT is not soluble in the acid solution, this peripheral region probably consists of amorphous silica phases formed predominately from the tetrahedral sheet structure.
Figure 5 shows the TEM picture with different magnifications of montmorillonite clay sample modified by 4N Sulfuric acid solution (MN-4). Aggregate of modified clay are illustrated at figure 5(a). It was observed that thickness of the clay plans and plan neighbor layers is broaden the end of the layered silicates (elliptical circle). It is resulted by disorientation of the montmorillonite layers. Treatment of MMT by acid removes aluminum octahedral layers between the two insoluble silicate layers. Moveable silica acids like dendrimers are formed [28]. Dissolution of border and last part of middle aluminum layer and simultaneously formation of amorphous silicate acids cause widening of the 2:1 silicate layers and montmorillonite crystal planes which is illustrated at figure 5(b). In addition, this widening was distinguished from SEM image of the montmorillonite modified by 4N (equiv/L) sulfuric acid solution. As illustrated in Figure 7, the edge of the 2:1 silicate layers plane is thicker than middle of it.
In addition, SEM images confirmed variation of MMT
(a)
(b)
Figure 5. TEM micrograph of montmorillonite sample modified by 4N Sulfuric acid solution (MN-4), (a) clay aggregates and (b) clay sheet layers.
morphology with increasing of solution acid concentration. The morphology of the non-treated clay was relatively uniform, containing primarily flat layers of several microns length, as shown in Figure 8(a). As the clay became modified by the acid solution, the morphology of the MMT changed from the large flat layers to smaller layers and fragment pieces and the conformity of the layers decreased.
With even small acid concentrations, the morphology of the clay changed quickly, it became very crusty and flaky, as shown in Figure 8(b), segmented clusters instead of the former flat and uniform layers existed [21]. As shown in Figure 8(c), the uniform and flat morphology was increasingly destroyed and nanoclay layered pieces were separated from their tactoids, with further increase in acid. The dissolution of octahedral aluminum layers and ions in addition to tetrahedral silicate were the reasons for the reduction and disruption of crystallinity characteristics (Figure 1) and related properties of the clay. It can destroy the crystalline structure of MMT [28,29]. By destroying the MMT crystalline structure, the aggregation of individual clay particles decreases.
(a)
(b)
Figure 6. TEM micrograph of (a) pure montmorillonite sample (MN-0) and (b) montmorillonite sample modified by 4N Sulfuric acid solution (MN-8).
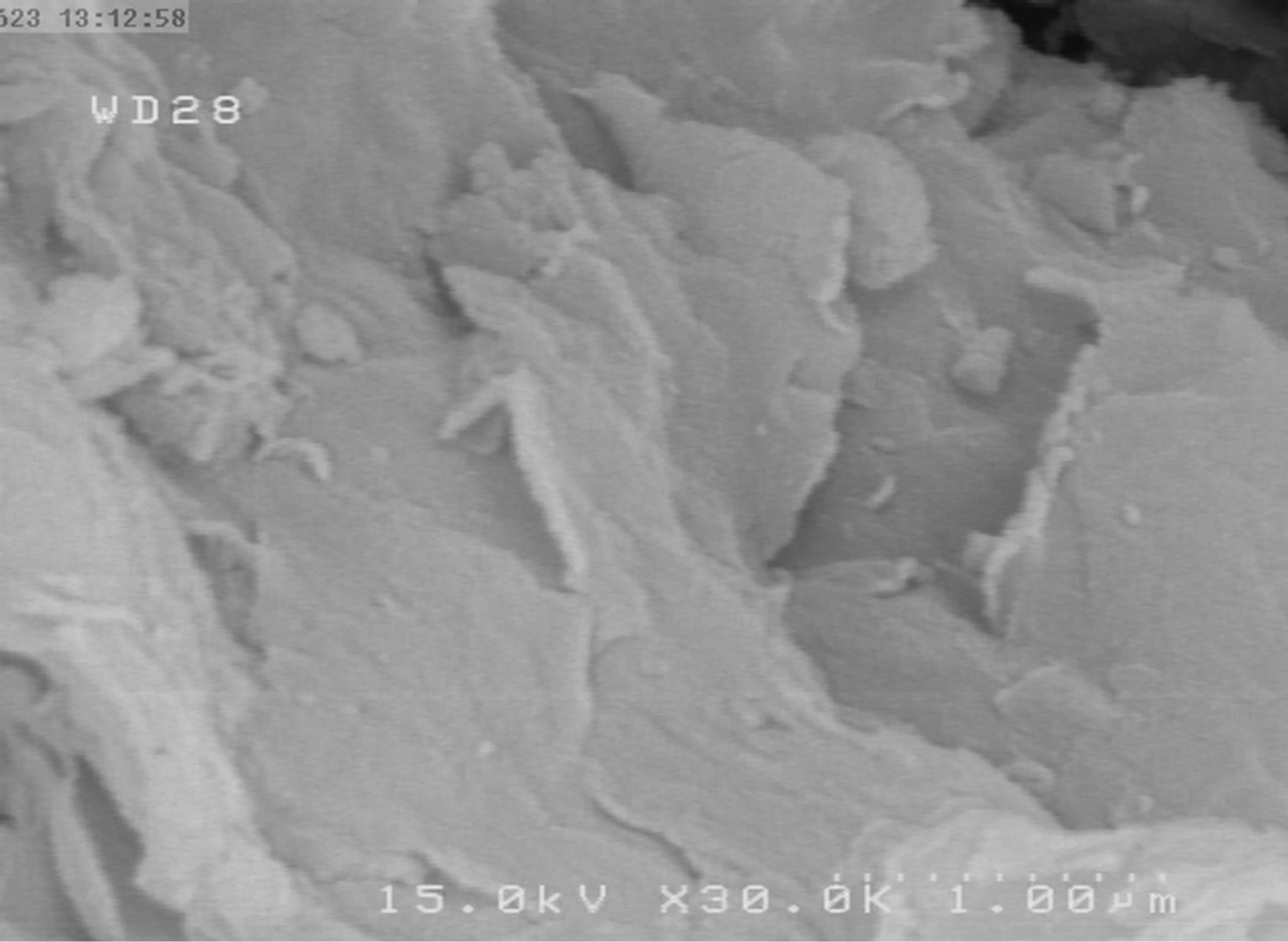
Figure 7. SEM image from montmorillonite sample modified by 4N sulfuric acid solution (MN-4).
At a very high acid concentration, the dissolution of intermediated aluminum layers becomes so intense that the cluster loses its flat and uniform morphology. Destruction, crack, and discontinuity are predominantly visible in Figure 8(d) on the clay cluster. Since leaching
is quite severe for this acidic strength, the most part of layered structure of the clay material disintegrated to give an amorphous phase.
4. Conclusions
The effect of the acid treatment on the chemical and physical structure and morphology of the montmorillonite clay were investigated. The FTIR spectrum confirmed that the original structure of the montmorillonite was not fully destroyed, and the Si tetrahedral layers of the clay were only affected at a high acid concentration. XRF and FTIR analyses illustrated that the SiO2 content and Si/Al ratio increased by enhancing the acid strength; simultaneously the content of Al2O3, MgO, and Na2O decreased progressively. Silicon oxygen network shielding and the barrier effect of the generated free silica were observed through dissolution of the clay component (Al, Mg, and Na). The transformation and change of the montmorillonite crystallinity percentage and structure with the acid activation could be distinguished by the XRD pattern. The crystallinity amount and structure of the clay material were found to be altered with the acid treatment and the clay became, to some extent, semicrystalline at high acid strength.
TEM and SEM pictures showed that the morphology of the MMT changed from the large clay layers of flat layers in pristine to smaller layers and fragment pieces in treated clays as modified by the acid solution. The disorientation and disruption of the MMT layers could be observed.
5. Acknowledgements
We are grateful to Absar Kavir Co. manager, Mr. Mirjalili for permission to use their laboratory instruments and we wish to express our sincere thanks to Mrs. Safaie for her kindly help during this work.
NOTES