Production and Characterization of Green Biosorbent Based on Modified Corn Cob Decorated Magnetite Nanoparticles ()
1. Introduction
Adsorbent cost is one of the crucial and important factors to know the economic feasibility of the process, designing and installing water and wastewater treatment plants [1] [2] . It is for this reason that, these last decades, waste biomasses were approved as renewable and inexpensive sources of high value biomaterials widely used for the synthesis of adsorbents. However, the effective use of these waste biomasses provides value-added products and functional materials, thus minimizing environmental pollution.
Among waste biomasses, corn cobs were widely investigated as precursors for the preparation of activated carbon [3] [4] [5] [6] . Activated carbon was recognized as one of the oldest and widely used adsorbents for the water and wastewater treatment for removing organic and inorganic pollutants [7] [8] , but the pyrolysis or physical activation involves high energy. The present study aimed to investigate the possibility to prepare an effective and low-cost sorbent from waste biomasses avoiding high energy consumption. Moreover, magnetic adsorbent technology has received considerable attention recent years because of the fact that it has the capability to treat large amounts of wastewater within a short time and can be conveniently separated from wastewater [9] [10] . From this viewpoint, activated raw corn cobs without carbonization recovered with magnetite nanoparticles have attracted our attention.
Among the heavy metals having high detrimental effect on the environment and human health, hexavalent chromium Cr(VI) is most often cited because of its high mobility and carcinogen effect [11] [12] [13] . Cr(VI) concentration in industrial wastewater was found to be between 0.5 and 270 mg/L while the permissible level indicated by the World Health Organization (WHO) is 0.05 mg/L [14] [15] . Therefore, processes have to be developed by scientific community to bring Cr(VI) concentration down to the permissible effluent standards.
In this study, green biosorbent was prepared from corn cobs which constitute a thorny environmental pollution problem. The magnetized activated corn cob was characterized by various spectral and analytical technics such as FT-IR, XRD, FE-SEM associated with EDX, HR-TEM, TG analysis, BET surface area analysis and XPS, to evaluate its use for the removal of Cr(VI).
2. Materials and Methods
2.1. Preparation and Modification of Corn Cob Wastes
The corn cobs were collected from local farmers. The used corn cob samples were taken from the middle part of corn cob, washed several times with distilled water to remove surface impurities and dried under the sunlight. And then the sufficiently dried cobs, would dried in oven at 80˚C for 24 h. After removal of the pith part, the dried corn cobs were crushed, washed several times with distilled water, and dried in the oven at 80˚C for 72 h, respectively. Finally the dried corn cob was grounded in a planetary micro pulveriser at 500 rpm for 10 minutes, sieved and called in this study as RCC.
The modification of the biosorbent was performed using NaOH solution. In the typical process, 5 g biosorbent was poured in 100 ml NaOH solution (2.5 mol/L) and vigorously stirred for 24 h at room temperature. Thereafter, the obtained mixture was filtered, and washed several times using distilled water until the washing solution pH becomes neutral and then the activated biosorbent (ACC) was dried in oven at 80˚C for 24 h. The process was finalized sieving the activated biosorbent in order to obtain uniform particle size.
2.2. Magnetization of the Modified Corn Cob
0.5 g of the modified corn cob was dispersed in 100 ml of 10 mmol of ferrous sulfate and magnetically stirred. Then, 10 ml of 10% sodium hydroxide solution was added drop wise to precipitate the hydrated iron oxides. The mixture was then shaken on a magnetic stirrer and heated to 100˚C for 1 h [16] . After cooling, the resultant magnetic biosorbent was several times washed with distilled water until its pH became neutral. The magnetized biosorbent derived from chemically activated corn cob (MACC) was separated by simple magnetic procedure, dried and stored in an airtight container.
2.3. Characterizations
All the biosorbents prepared in the present study, namely RCC, ACC and MACC, were submitted to important characterization at CSIR-North East Institute of Science and Technology Jorhat, Assam, India. The crystallinity characteristics of the biosorbents were determined using X-Ray diffraction (XRD) analysis. The XRD patterns were obtained on a Rigaku Ultima IV model Advance diffractometer using Cukα radiation (λ = 1.54 Å) at 40 kV, 40 mA, at a scan rate of 0.2˚ 2θ/min with a Bragg angle ranging from 2˚ to 80˚ 2θ. The functional groups present on the surface of the prepared biosorbents and after their interaction with Cr (VI) solution was analyzed by Fourier transform infrared (FT-IR) spectrometry. The FT-IR spectra were recorded in the range of 4000 - 400 cm−1 using the KBr technique with a Shimadzu MS-11-IR affinity-1 spectrophotometer at a spectral resolution of 4 cm−1. The Brunauer-Emmett-Teller (BET) specific surface areas and pore size of the sample were measured by adsorption and desorption isotherms of nitrogen gas at 77.35 K collected from an Autosorb-1 (Quantachrome, USA) surface area and pore size analyzer. The field emission scanning electron microscope (FE-SEM) was performed to observe the surface morphology using a Carl Zeiss (Sigma VP) scanning electron microscope. The high-resolution transmission electron microscopy (HR-TEM) analysis was carried out using a JEOL JEM 2100, Japan operated at an accelerating voltage of 200 kV. The X-ray photoelectron spectroscopy (XPS) measurements were carried out using a Thermo-Scientific ESCALAB Xi + spectrometer having a monochromatic Al Kα X-ray source and a spherical energy analyzer that operates in the CAE (constant analyzer energy) mode using the electromagnetic lens mode. The thermal behaviors of the samples were determined in an SDT Q600 thermal analysis system under argon atmosphere with a flow rate of about 100 ml/min and a heating rate of 10˚C/min from room temperature to 750˚C.
3. Results and Discussion
3.1. X-Ray Diffraction Analysis and Infrared Spectroscopy
Figure 1 shows the XRD patterns and FT-IR spectra of the prepared biosorbents.
(a)
(b)
Figure 1. (a) XRD patterns of RCC, ACC and MACC; (b) FT-IR spectra of RCC, ACC and MACC and MACC after Cr(VI) adsorption.
In the XRD profile, the broad peak appearing at ~22˚ corresponding to the (002) peak indicates the lesser cristallinity of ACC. However, this peak might be shielded by strong peaks of magnetite in MACC. A series of peaks (220), (311), (400), (422), (511), and (440), characteristic of the inverse cubic spinel phase of Fe3O4 were observed [17] , suggesting the formation of crystalline magnetite.
In the FT-IR pattern of ACC (Figure 1(b)), the absorption peak centered at 3290 cm−1 corresponds to the −OH stretching vibration of the associated water molecules. The peak observed at 2913 cm−1 could be due to the presence of C–H asymmetric stretching of –CH3 groups [18] . In addition, the spectra of the prepared samples show several other bands, which could be assigned to C=C at 1435 cm−1, C−O at 1058 cm−1, and C−H or O−H at 894 cm−1. This indicates that mainly amorphous carbons with different oxygen groups are in the prepared biosorbents. Therefore, these functional groups could play an important effect on the surface properties of the biosorbents as well as on their adsorption characteristics. The very strong absorption peak at 572 cm–1 in the FT-IR spectrum of MACC is related to the Fe-O bond stretching vibration. After Cr(VI) adsorption, some peaks shifted to another frequencies with a decrease in the transmittance intensity.
3.2. Surface Characterization
The nitrogen adsorption-desorption isotherm and pore distribution of the adsorbents are presented in Figure 2(a) inset. The International Union of Pure and Applied Chemistry classification indicated Type IV nitrogen adsorption isotherms associated with hysteresis loops, suggesting the adsorbent-adsorbate interaction weaknesses [19] . The specific surface area, pore diameter average and pore volume of biosorbents determined BET method were presented in Table 1. The BET surface areas of raw, activated and decorated corn cobs were found to be 5.87, 14.85 and 35.22 m2/g, respectively. This indicates that coating the activated biosorbent surface with magnetite increases the surface area thus enhancing its efficiency for adsorption application. This could be due to the nanostructure of magnetite particles [20] [21] . The pore volume was determined to be 0.031, 0.035 and 0.147 cm3∙g–1 for RCC? ACC and MACC, respectively. The pore diameter average of the biosorbents indicates the presence of mesopores.
SEM analysis plays an important role in material surface characterization. As it can be seen, Figure 2(b) and Figure 2(c) display the well deposit of magnetite nanoparticles over the surface of the modified corn cobs. After the attempt of Cr(VI) removal from aqueous medium, EDS spectrum (Figure 2(d)) shows a peak corresponding to Cr thus confirming its adsorption on MACC.
3.3. High-Resolution Transmission Electron Microscopy
To acquire more informations about the internal structure of magnetically activated corn cob, TEM observation was carried out and shown in Figure 3. Quasi-spherical shape of magnetite nanoparticles with a size of around 47 nm was observed. The distance between two lattice fringes of 0.295 nm corresponding to the (220) plan of magnetite nanoparticle was observed. The lattice plane (220), (311), (511) and (440) corresponding to the crystalline lattice of magnetite nanoparticles were found in the selected area electron diffraction (SEAD) pattern.
![]()
Table 1. Biosorbent BET surface area properties.
(a)
(b)
(c)
Figure 3. (a) TEM and (b) HR-TEM images with lattice fringes for (220) plane of MACC and (c) SAED pattern.
3.4. X-Ray Photoelectron Spectroscopy
The exact chemical elements present in the biosorbents were determined using X-ray photoelectron spectroscopy analysis as shown in Figure 4. The full XPS survey spectrum indicates peaks at 285.36, 530.09 and 710.87 eV which are assigned to C1s, O1s and Fe2p indicating the presence of C and O in the raw and activated corn cobs and C, O and Fe in the magnetite decorated one. It was clear that the Cr2p peaks appeared at 578.14 eV after the adsorption of Cr(VI), indicating the successful adsorption of Cr(VI) ions on MACC. The high resolution Cr2p spectrum shows two distinct peaks at 587.3 and 577.4 eV which correspond to Cr2p1/2 and Cr2p3/2, respectively. This phase suggested that Cr(VI) was not reduced to Cr(III) due to rapid adsorption [22] . According to the survey spectrum of MACC after Cr(VI) adsorption the relative atomic percentage was found to be 47.22%, 15.41%, 35.62% and 1.75% for O, Fe, C and Cr respectively.
(a)
(b)
Figure 4. (a) XPS survey spectra of RCC, ACC, MACC and MACC-Cr and (b) high-resolution Cr2p XPS spectrum of MACC-Cr after adsorption.
3.5. Thermal Behavior
The TG and DTG thermograms of the biosorbents are given in Figure 5. The DTG curve indicates that the biosorbent degrades in two significant stages. The first stage centered at about 50˚C with weight loss less than 5% is due to the loss of physically adsorbed water molecules and the second stage corresponding to the degradation of cellulose and hemicellulose occurs at a maximum value around 340˚C with rapid weight loss of about 70% for raw and activated corn cobs and 25% for decorated one. This suggests that the deposit of magnetite nanoparticles increases the thermal stability of the biosorbent which will be helpful in its thermal regeneration.
(a)
(b)
Figure 5. (a) TG and (b) DTG curves of RCC, ACC and MACC.
4. Conclusion
A value was added to waste environment polluting corn cob to produce biosorbent. The biosorbent was coated with magnetite nanoparticles to enhance its adsorption capacity. BET surface area indicated a maximum surface 35.22 m2/g. FE-SEM and HR-TEM micrographs confirmed the well distribution of the magnetite nanoparticles with a size of around 47 nm. XPS and EDX spectra have shown that Cr(VI) can be adsorbed on the prepared green biosorbent. Therefore, the proposed biosorbent could be a promising candidate for Cr(VI) removal from wastewater.
Acknowledgements
The authors gratefully acknowledge Director, CSIR-NEIST, Jorhat for the facilities for the work. We express our heartfelt thanks to Dr. R.L. Goswamee, Head and Group Leader of Materials Science and Technology Division for support.
Appendix
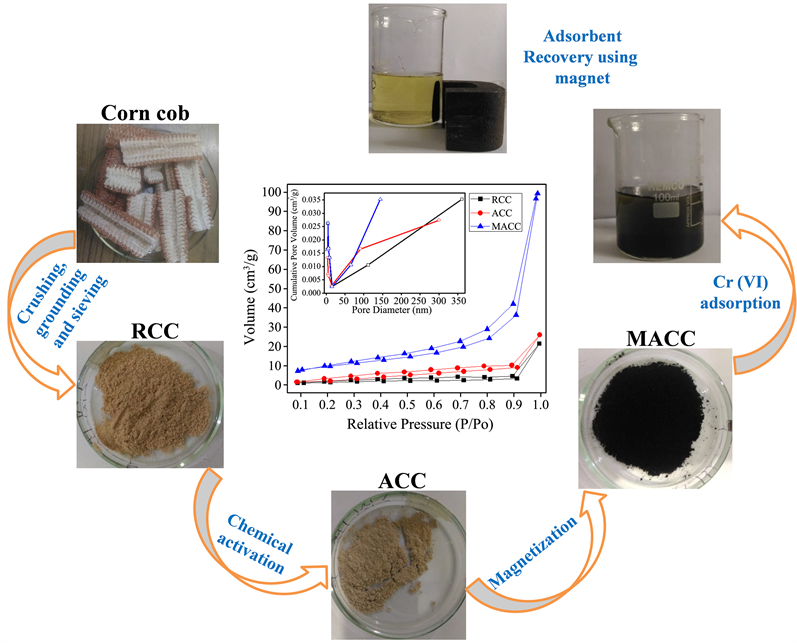