Assessment of the Concentration of Petroleum Hydrocarbon in Oily Wastes Residual Ash at Bodo-Ogoni Remediation Site, Nigeria ()
1. Introduction
Hydrocarbon is the principal component of petroleum wastes formed when crude oil’s properties change as a result of changes in eternal conditions. The formation of oily wastes are commonly caused by cooling below the cloud point, evaporation of light ends, mixing with in compatible materials, and the introduction of water to form emulsions (Johnson & Affam, 2019). In the formation of oily wastes from remediation of hydrocarbon pollutants, involving thermal treatment, the process include drying, followed by pyrolysis and disintegration of organic structures into tars, gasses and char with the evolvement of volatile inorganics such as alkali metals, where reduction in levels of heavy metals is determined later on as higher temperatures are attained (Nzikhou & Stanmore, 2013).
In the exploration and production of petroleum, wastes are generated which includes drilling fluids, drill cutting, produced water, petroleum effluent treatment plant sludge, crude oil spills emulsions (Johnson & Affam, 2019). Other hydrocarbon wastes generated in the value-chain of crude oil production are those arising from the remediation of oil contaminated sites. Hydrocarbon wastes contained several to toxic compound; TPH, PAH Polychlorinated biphenyls (PCBs), heavy metal; barium, lead, zinc, mercury, arsenic, chromium and nickel (Bojes & Pope, 2007; API, 1989). Admons et al. (2001) reported that petroleum wastes from refineries contain high concentration of metals; Zn (1299 mg/kg), Cu (500 mg/kg), Pb (565 mg/kg), Cr (480 mg/kg) and Ni (480 mg/kg).
The improper disposal of petroleum hydrocarbon wastes in the environment creates a modification in the chemical and physical properties of the surrounding soils (Robertson et al., 2007), deficiency in nutrients and stunted growth in vegetation of receiving soils as the oily wastes are fixed into soil pores (Trofimov & Rozanova, 2003), reduction in hygroscopic moisture, hydraulic conductivity, wetting power of soils and decrease in the diversity of soil microorganisms (Suleimanov et al., 2005).
Several methods; thermal, mechanical, biological and chemical have been applied for processing and disposing petroleum hydrocarbon wastes generated from remediation programs, with the purpose of reducing its toxic concentrations. Reduction in concentration of heavy metals in oily wastes has been reported to be influenced by the treatment facilities, length of exposure to high temperature of the particles (Zeuthen, 2007), and behavior of heavy metals (Nzikhou & Stanmore, 2013; Obernberger et al., 2006). Heavy metals caused by applications of some biomass materials may become concentrated after use into residual wastes (Nzikhou & Stanmore, 2013), and during combustion Pb, Zn and Cd tend to vaporize and re-condense on the surface of fine particles or ash when incinerated (Obernberger et al., 2006).
Low temperatures in treatment facilities caused increase in the concentration of Cd, Pb, in the ash, but at temperature above 1250˚C a reduction in concentration occurred (Obernberger et al., 2006). Zeuthen (2007) showed that a combination of type of furnace, length of exposure to high temperature plays key roles in the reduction of the concentration of heavy metals in hydrocarbon waste.
Accordingly, Cd, Pb are among the metals that increased in concentration in the finer aerosol particles formed in a 22 mwt grate incinerator, but thermodynamic equilibrium is attained in large particle from grate furnaces because of the length of exposure to high temperature of the particles, and under incineration conditions Cd and Pb read almost ten minutes to diffuse out of an ash particle of the size found in incinerators.
In 2008, two major oil spills from shell petroleum development company (SPDC) pipeline occurred in Bodo Creeks with devastated impacts on over 1000 hectares of mangroves and their biological inhabitants. The oil spills were caused by leaks in the trans-niger pipeline which transports an estimated 120,000 - 150,000 barrels of crude oil per day (bpd) through ogoniland (UNEP, 2011). The impacted habitats are cleaned up with remediation activities and hydrocarbon wastes are generated, consequently.
The generated wastes are taken to treatment facilities for the production of residual ash to assess the concentrations of petroleum hydrocarbon chemicals and ascertain if it exceeds permissible limits. However, there is little or no information on the chemical composition of such wastes after treatment in the Nigeria Nation, in spite of the fact that the non-reduction of the concentration of hydrocarbon wastes generated from remediation activities disposed on the soil will have a devastating impact on the functioning of the soil ecosystem and its services.
This present study was undertaken with an objective to determine the concentration of petroleum hydrocarbon in the residual ash from the treated (incinerated) oily wastes from the Bodo-Ogoni remediation activities. This present study is important as it indicates the concentration of the TPH, PAH and heavy metals contained in treated oily wastes. The efficiency of the treatment facilities in the reduction of the concentration of the hydrocarbon wastes is also exposed by the study.
2. Materials and Methods
2.1. Description of Study Site
The study was conducted at collection site located at Bodo (N4˚36'29.7"N; E7˚15'30.2"), and characterized by low-lying mangrove vegetation and numerous tidal creeks lined with soft mud, substrates on the shorelines and abandoned fish ponds.
The treatment sites were located Mosab and Networld (N4˚46'10"; E7˚8'39"), MSTSC and paschal (N4˚55'44"; E7˚O'16"), ICREN (N4˚54'25"; E7˚5'33") and ITS (N4˚54'20") (Figure 1). All treatment sites applied both thermal desorption and incinerator treatment method.
2.2. Research Design
The six treatment sites were each replicated four times in a Completely Randomized Design.
2.3. Sample Collection
The petroleum hydrocarbon solid wastes which comprised contaminated soil, glasses, plastics, vegetation generated during the clean-up at Bodo Creek were collected in 50 cm polythene bags. Liquid oily wastes were also collected from the collection sites in 50 liters jerry cans. Both solid and, liquid oily wastes were segregated at Bodo Patrick waterside segregation point and thereafter to the various treatment sites. At the end of treatments, residual ash-waste samples were collected from stored batches at each of the treatment activities. Untreated samples were also collected before treatment.
![]()
Figure 1. Locations of waste source and treatment facilities.
All collected samples were labelled and preserved in ice-cube parked containers and taken to the reference laboratory of National Oil Spill Detection and Response Agency, Port Harcourt, Nigeria for Extraction. Quality control measures were carried out by using trowel cleaned with ethanol and water to reduce cross-contamination (NOSDRA, 2006).
2.4. Extraction of TPH and PAH
Ten grams each of aliquot of untreated and treated well-mixed ash samples were placed in a solvent rinsed beaker and added anhydrous sodium sulphate until the ash particles were loosened. Twenty millitres of 50 ml dichloromethane solvent was added and shaken in a vortex mixer for 5 minutes. The samples were then placed in a sonicator for 10 minutes at 70˚C. At the end of the duration, the extract was filtered through a glass funnel containing glass wool and anhydrous sodium sulphate. Thereafter, the extract was transferred to a Teflonlined screw cap for analysis.
2.5. Extraction of Heavy Metals
One gram of ash samples and untreated samples were weighed and transferred into test tubes and 20 ml aqua regial (hydrochloric acid and nitric acid; 3:1) solution was added. The resulting mixtures were digested for about I hour at 100˚C on a hot plate. The resulting digests were allowed to cool and then filtered into a 100 ml flask and made up to mark with deionized water and analysed in an atomic absorption spectrophotometer (AAS) (AAS Raleigh WF × 320) after calibrating the equipment with different standard concentrations.
The concentration of four element Cd, Pb, Zn and Mn were determined in both untreated and treated hydrocarbon wastes. The selected metals concentrations were calculated in mg/kg being the closet benchmark in EGASPIN measurement.
2.6. Statistical Analysis
The data obtained from the extraction and analysis were subjected to statistical analysis using analysis of variance (ANOVA). This was done to determine significant differences in concentrations of TPH and PAH between the ashes collected from the treatment sites.
3. Results and Discussion
3.1. Results
A total of six (6) residual pollutants comprising four (4) heavy metals; cadmium, lead, Zinc and manganese and two petroleum hydrocarbons (TPH and PAH) were obtained in all the samples. All the results are expressed in from of mean ± standard deviation for four replicates per parameter. The total concentration of TPH and PAH obtained from the six sites indicate that the concentration of the residual ash was below the department of petroleum resource intervention (DPR, 2002) level of Nigeria but exceeded the DPR target value for TPH and PAH in sediments.
Table 1 showed the TPH and PAH of the residual ash generated before (untreated) and after treatment of remediation wastes in TDU and incinerators. The mean result showed that the untreated samples contained concentrations of TPH and PAH as 12,000 mg/kg and 23 mg/kg, respectively.
In the treated wastes, the concentrations of TPH in ashes collected from two treatment sites were greatly above 500 mg/kg; Mosab (704.46 ± 219.52 mg/kg) and ITS (668.74 ± 309.66 mg/kg), slightly above 500 mg/kg in two treatment sites; Paschal (598.02 ± 399.66 mg/kg) and net world (501.11 ± 355.13 mg/kg), and below 500 mg/kg in two treatment sites; ICREN (463.25 ± 205.29 mg/kg) and TMCH (320.18 ± 355.13 mg/kg).
The least concentrations of PAH in ashes collected from treatment sites was 1.24 ± 2.4 mg/kg (paschal) followed by 4.75 ± 7.48 mg/kg (ITS) (Table 1). The PAH concentrations of above 10 mg/kg in descending order were recorded in ashes collected from three treatment sites; Mosab (16.14 ± 6.36 mg/kg) net world (14.11 ± 14.24 mg/kg) and TMCH (10.46 ± 14.68 mg/kg) (Table 1). In Table 2 the concentration of heavy metals (Cd, Pb, Zn and Mn) in the residual ash from the treated ash and untreated wastes are presented. The results showed that only two sites; ITS and Paschal were able to detect the level of Cd. The highest levels of Pb and Zn obtained were 45.97 ± 18.71 mg/kg. (ITS) and 45.25 ± 12.75 mg/kg (paschal) and 84.29 ± 17.81 mg/kg (1ICREN), respectively. A high level of 136.7 ± 81.61 mg/kg was recorded from Mn (ITS). The target values; 0.8 mg/kg, 85 mg/kg and 140 mg/kg for Cd, Pb and Zn, respectively. In the untreated wastes,
![]()
Table 1. Total petroleum hydrocarbon (TPH) and poly aromatic hydrocarbon (PAH) in the residual ash from oily wastes generated in the remediation of Bodo.
Exceedance Value; TPH (5000 mg/kg); PAH (40 mg/kg); BDL (Below Detection Limit).
![]()
Table 2. Concentration of Cd, Pb, Zn and Mn in the residual ash from oily wastes generated in the remediation of Bodo.
Exceedance Value; Cd (12 mg/kg); Pb (530 mg/kg); Zn (720 mg/kg); Mn (N/A), BDL: Below Detection Limit.
the levels were 0.15 mg/kg (Cd), 0.59 mg/kg (Pb), 3.45 mg/kg (Zn) and 2.8 mg/kg (Mn).
3.2. Discussion
Olawuyi and Zibima (2018) stated that the values of TPH and PAH stipulated by EGASPIN, the regulatory agency in Nigeria for which intervention or remediation would be conducted is extremely high as it is 5000 mg 1 kg (TPH) and 40 mg 1kg (PAH) our study agrees with this report particularly as the concentration of TPH recorded at two treatment sites; ICREN (463.25 ± 205.29 mg 1 kg); and TIMCH (320.18 ± 355.13 mg 1 kg) is high enough, yet it is below the intervention limit. This is caused by inefficiency of treatment facilities or process. The marginal or slight significant difference recorded in statistical data give credence to this study.
The results obtained in our study implies that the treated oily wastes (ash) still contain higher levels of TPH and PAH in spite of the reduction in concentration over the untreated oily wastes.
This is because the values obtained though were below those obtained in the untreated oily wastes, they were all above the target values (50 mg/kg TPH and 1.0 mg 1 kg PAH). The high level of TPH recorded affirm the report of Jebeli et al. (2019) that TPH is the main pollutant in oily wastes collected from treatment plants which was higher in concentration than the acceptable clean up levels for TPH.
Nzikhou & Stanmore (2013) have posited that concentrations of heavy metals increased in residual wastes due to what Obernberger et al. (2006) described as type of vaporization and re-condensation. The lower values or concentrations of heavy metals recorded in the untreated samples in our study which is also below the target values is in line with the studies of earlier authors. The high level of heavy metals in the treated ash recorded in our study supports the report that Pb, and Cd usually vaporize and re-condense on the surface of ash when incinerated Obernberger et al. (2006).
The increased in concentration of ash after treatment is caused by the temperature of the treatment facilities which were not more than 100˚C and could not stop the re-condensation. This probably led Zeuthen (2007) to report that, high temperatures of 1250˚C, under incineration conditions made Cd and Pb to diffuse out of ash within 10 minutes of exposure. Our study indicates the inefficiency of treatment facility pointed out.
The increase values of heavy metals recorded in our study were all above target values and this is supported by the statistical data which pointed out significant difference (P < 0.05) between heavy metal content across sites and their target values (Appendixes 1-6).
4. Conclusion
The concentration of TPH, PAH, and heavy metals contained in treated hydrocarbon wastes after remediation was determined in this present study. The results showed that the remediation activities did not reduce the concentration of heavy metals to permissible limit, indicating that the treatment facilities were not efficient, though a reduction in TPH and PAH was achieved. With reference to available literature, the high metal concentration is caused by non-application of required temperature in the treatment process and the weakness of the treatment facilities.
The study has added to knowledge that the remediation of Bodo-Ogoni hydrocarbon polluted sites is yet to achieve the reduction of metal concentration, and therefore requires a more appropriate approach.
Appendix 1
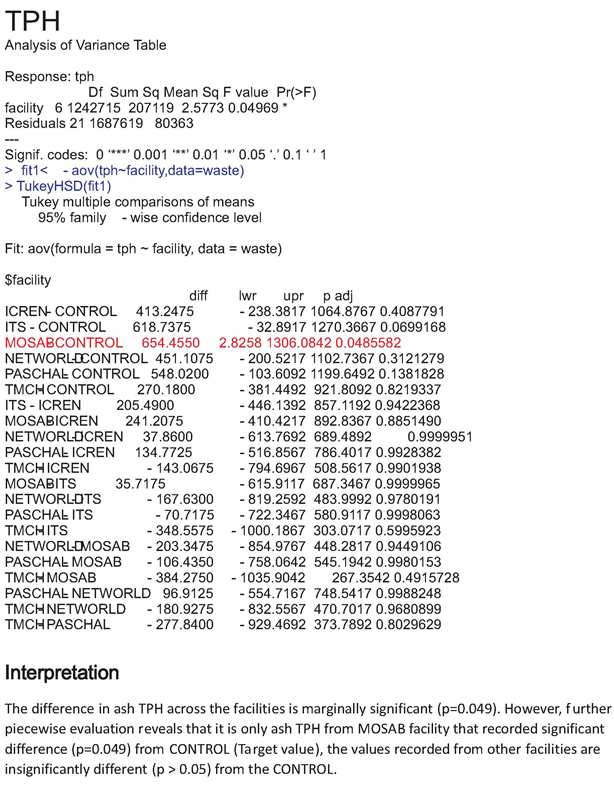
Appendix 2
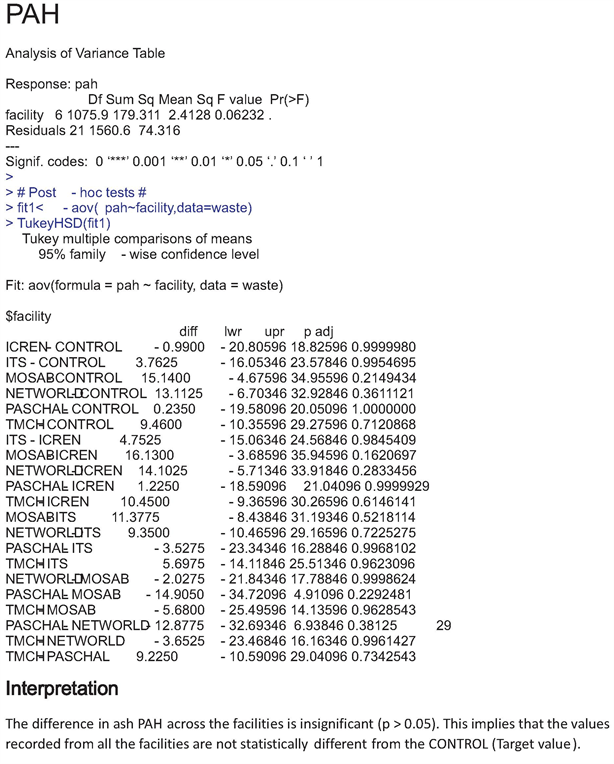
Appendix 3
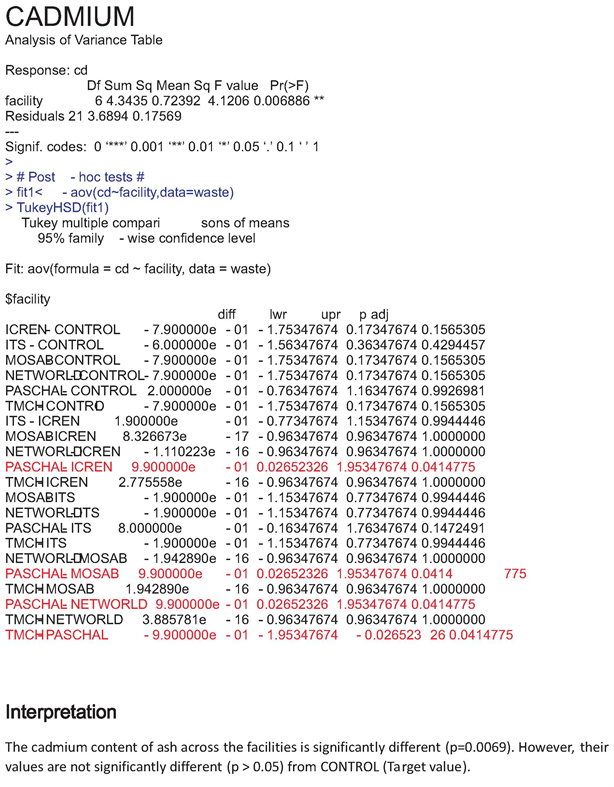
Appendix 4
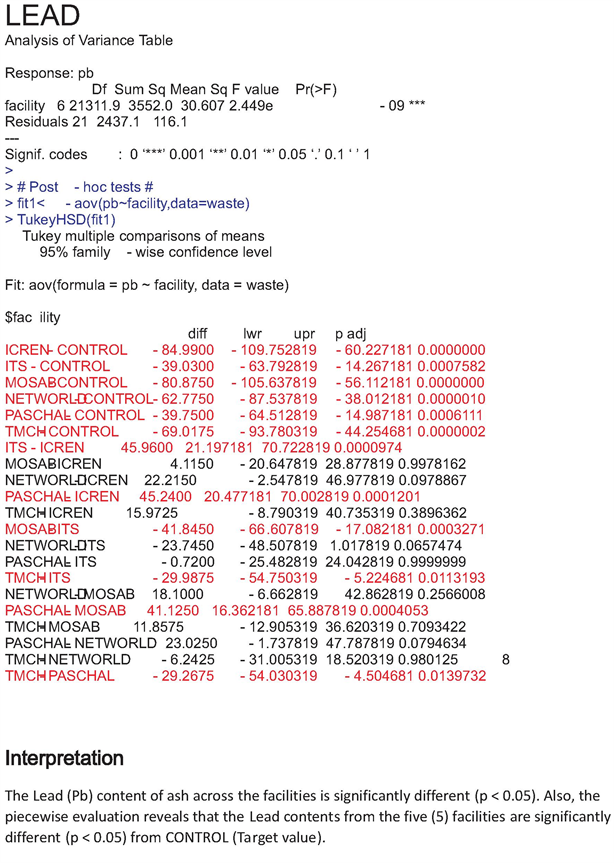
Appendix 5
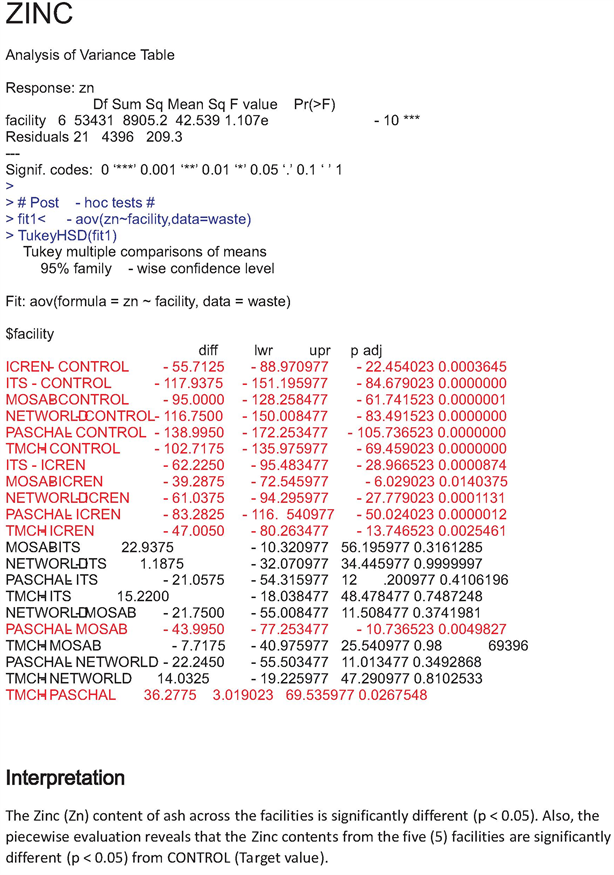
Appendix 6
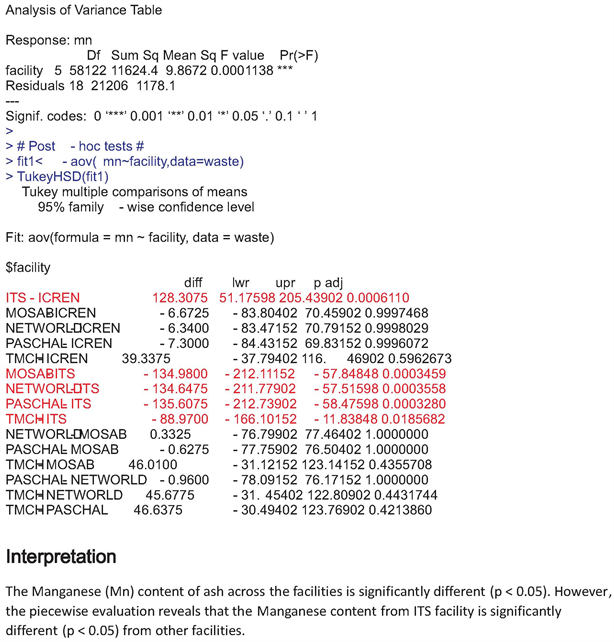