Synthesis, Characterization and Bioactivity of 1,1-bis(2-Carbamoylguanidino)furan-2-ylmethane ()
1. Introduction
Bacteria and fungi resistance to antimicrobial agents pose a great challenge to the world. In the midst of the earlier decades, the global death rate increased significantly, due to the infectious diseases caused by drug-resistant micro-organisms [1]. Hence, in the recent years, much consideration has been devoted to the problem of multi-drug resistant bacteria and fungi, resulting from the extensive use and abuse of classical anti-microbial agents.
The treatment of bacterial infections can be significantly complicated by the ability of bacteria to develop resistance to antimicrobial drugs. Bacteria may be inherently resistant to antimicrobial drugs (inherent resistance) or may acquire resistance by de novo mutation or by receiving genetic material from resistant bacteria (acquired resistance) [2]. Acquired resistance can be manifested as the production of enzymes which degrade the antibacterial drug, the expression of the efflux system which prevents the drug to reach the target site of action within the cell, the modification of the drug action target site, or the development of the alternative metabolic pathway to which the drug has no effect [3].
Synthesis of an organic compound from renewable resources has attracted the attention of many research workers because of the escalating price of petrochemicals. Furfural derivatives are obtained from a biomass-based agricultural by-product furfural [4]. Furfural is an indispensable chemical that is potentially relevant to the economy of a developing country on the platform that it is a biorenewable chemical, readily available, cheap, and that could also be seen as a possible feedstock for most consumers in developing countries [5] [6]. Recently, furfural has gained attention as a potential chemical for organic synthesis and its derivatives have vast applications both in chemical and pharmaceutical industries [7] [8]. Some of the evidences where furfural derivatives were used for synthesis are: Synthesis of furfurylamine [9], furfurymaleic acid [7], 5-(3-nitrophenyl)-4-(2-furyl)-3-buten-2-one [8], nitrofurazone [10], etc. Derivatives of furfural have been potently used in bioactivities of microorganism and corrosion inhibitions of various metals at different temperatures [11]. 1,1-bis(2-carbamoy-lguanidino)furan-2-ylmethane in this study is synthesized via a product, 1-((2-Carba-moylguanidino)(furan-2-ylmethyl)urea that was serviced as an intermediate [6]. 1-((2-Carbamoylguanidino)(furan-2-ylmethyl)urea that was not naturally available was synthesized with purified furfural and urea in the presence of sodium hydroxide (2 mL, 20%) [6]. In view of these data, we have undertaken the synthesis, characterization and antimicrobial evaluation of 1,1-bis(2-Carbamoylguanidino)furan-2-ylmethane and is shown in Figure 1 below.
![]()
Figure 1. 3D Structure of Synthesized of 1,1-bis(2-Carbamoylguanidino)furan-2-ylmethane (CFM).
2. Materials and Methods
2.1. Equipment Used in This Study
FTIR 8400S Fourier Transform Infrared spectrophotometer was used to identify the functional groups, Gas Chromatography/Mass Spectrometer-QP2010 PLUS SHIMADZU was used for molecular formula/mass identification, Nuclear Magnetic Resonance(Agilent-NMR-VNMRS400) was used for structural elucidation, Vacuum Pump (TKS-70), for vacuum distillation of furfural and Digital Melting Point Electrothermal IA9300X1, for melting point determination. Furfural was bought from a chemical store and purified by vacuum distillation. Other chemicals such as urea, ethanol, and sodium hydroxide were of Analar grade and used without further purification. Thin layer chromatography (TLC) was carried out using a Merck pre-coated silica gel plate (10 × 10 cm); the Rf value was obtained using solvent mixture of n-hexane and ethyl acetate in a ratio of 2:3. The chromatogram was visualized using an ultraviolet lamp at 256 nm.
2.2. Experimental Methods
2.2.1. Synthesis of 1-((2-Carbamoylguanidino)(furan-2-ylmethyl)) Urea
The procedure adopted in the synthesis of 1-((2-Carbamoylguanidino)(furan-2-ylmethyl)urea was reported by [6]. Furfural (40 mL, 0.48 mol), urea (40 g, 0.63 mol) and distilled water (10 mL) were placed in a flat-bottomed flask (250 mL). The mixture was heated in a water bath at a temperature of 60˚C for 1 hour, 40 minutes with vigorous stirring. Thereafter, NaOH (2 mL of 20% of solution) was added and the heating continued for 20 minutes. The mixture was cooled in ice bath and the resulting precipitate filtered, and washed with n-hexane, recrystallized using methanol and the crystal dried with the oven at 50˚C.
2.2.2. Synthesis of 1,1-bis(2-Carbamoylguanidino)furan-2-ylmethane (CFM)
The mixture of 1-((2-Carbamoylguanidino)(furan-2-ylmethyl) urea (5.0 g, 0.021 mol), urea (1.2 g, 0.021 mol), and ethanol (10 mL) was placed in a conical flaskand refluxed for 1 hour at a temperature of 70˚C with a magnetic stirrer. After completion, the reaction was placed in an ice bath for fast precipitation. Thereafter, it was filtered and washed with n-hexane, recrystallized from methanol. The crystal was dried in an oven at the temperature of 50˚C. The dried sample was analysed using Gas Chromatography-Mass Spectrometer, Infra-red spectrometer, Nuclear Magnetic Resonance, and Electrothermal Melting point instrument.
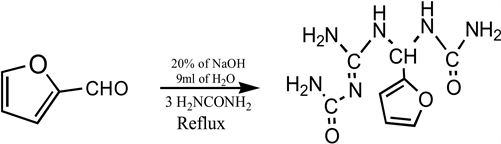
Equation 1. Synthesis of 1-((2-Carbamoylguanidino)(furan-2-ylmethyl)) urea.
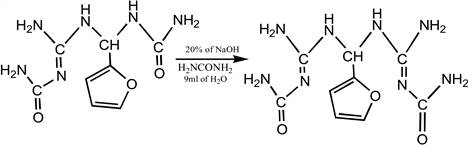
Equation 2. Synthesis of 1,1-bis(2-Carbamoylguanidino)furan-2-ylmethane.
2.2.3. Pathogen Isolation
The test pathogens (Escherichia coli, Salmonella typhi, Staphylococcus aureus and Bacillus subtilis) that were used for the antimicrobial screen were isolated from clinical samples obtained from Beracah Hospitals, Rukpokwu, Port Harcourt, Rivers State.
2.2.4. DMSO Solubility Screen
This was done to determine the concentrations of DMSO (ranging from 10% v/v to 100% v/v) that best dissolves test compounds/agents to be used for the screen. To carry out this screen, 0.1 g of each synthesized agent was suspended in 100 ml of the different concentrations of DMSO at room temperature with continuous agitation for 12 hours. After incubation period, the observed solubility patterns were recorded.
2.2.5. DMSO Toxicity Screen
This screen was carried out to determine the concentrations of DMSO (amongst those that achieved the best agent solubility) that didn’t show any inhibition pattern on the test microbial pathogens. The well-in-agar diffusion method was used to carry out this screen, where 10 µL of these different DMSO concentrations were introduced into wells (5 mm diameter) dug into Mueller Hinton agar plates inoculated with the different test pathogens (108 cells). After introduction, the plates were incubated at 37˚C for 24 hours. After incubation, observed zones of inhibition for the different DMSO concentrations screened, for the different test pathogens were recorded, with their average inhibition being determined. The DMSO concentration with the best solubility pattern on the antimicrobial test agents and also non-toxic to the test pathogens was used as the solvent for preparing the different concentrations of the antimicrobial test agents in order to determine their Minimum Inhibition Concentration (MIC) and Minimum Bactericidal Concentration (MBC).
2.2.6. Antimicrobial Test Agent Preparation
Different concentrations (100 mg∙L−1, 10 mg∙L−1, 1 mg∙L−1, 0.1 mg∙L−1, 0.01 mg∙L−1, and 0.001 mg∙L−1) of the antimicrobial test agents (Sample A) were prepared using serial dilution method with 50% v/v DMSO contained in different sterile test tubes and labeled appropriately.
2.2.7. Minimum Inhibitory Concentration (MIC) Screen
This screen was done to determine the least concentration of the test agents that is capable of inhibiting the growth of each microbial test pathogens. The screen was also carried out using the well-in-agar diffusion method (Vincent, 2005). For each test pathogen that was screened, a cell concentration of 108 cells was first inoculated into Mueller Hinton agar plates, after which wells (5 mm diameter) were dug and filled (10 µL) with the different concentrations of each of the test agent. The plates were then incubated for 12 - 24 hours at 37˚C. After incubation, the observed zones of inhibition for each concentration of each test agent on each test pathogen were recorded. MIC concentration was then determined by looking out for that particular minimum concentration of the test agent that was capable of inhibiting growth of a given test pathogen.
2.2.8. Minimum Bactericidal Concentration (MBC) Screen
This screen was carried out to determine the least concentration of the test agents that is capable of inhibiting the growth of each microbial test pathogen by killing their cells and the method adopted was by (Andrews, 2001). For each microbial pathogen, 0.1 ml of the cells (108) was introduced into test tubes containing 5 ml of the different concentrations of the different samples and incubated for 24 hours at 37˚C. After incubation, a loopful of the incubated concentrations was spread onto the surface of freshly prepared Mueller Hinton agar plates and further incubated 37˚C for 24 hours. After incubation, the minimum concentration of each sample that showed cell death (determined by the absence of visible colonies on Mueller Hinton agar plates after incubation) for a given pathogen, was considered the MBC.
3. Results and Discussions
3.1. Spectra Analysis Synthesized of 1-((2-Carbamoylguanidino)(furan-2-ylmethyl)) Urea
The IR data analysis of the product identified the presence of imine group (1532.87 cm−1), a carbamoyl group (1666.55 cm−1) and methine (2885.23 cm−1). This IR data analysis is in line with Orie et al. (2018). The 1H-NMR analysis of the product in DMSO showed multiplet peaks in the range (δ) 6.54 - 7.53 ppm which corresponds to the furan ring. The peak at 6.23 ppm is due to the proton around the methine group while the peak in the 5.6 ppm is assigned to proton around carbamoyl group. 13C-NMR data analysis indicates the presence of carbon from furan (106.12 - 154.75 ppm), imine (158.52 ppm), carbamoyl group (160.15 ppm) and methane (50.6 ppm). The meeting point analysis was at the range of 106˚C - 108˚C, the rf value in a solvent system hexane: ethylacetate (2:3) is 0.70. The physcochemical and spectroscopic data analysis showed are in line with Emmanuel et al. (2013), Orie et al. (2018) and Don-Lawson et al. (2020).
3.2. Spectra Analysis Synthesized 1,1-bis(2-carbamoylguanidino)furan-2-ylmethane(CFM)
The IR data analysis of the product identified the presence of imine group (1555.87 cm−1), a carbamoyl group (1672.0 cm−1) and methine (2770.60 cm−1) and amine group (3324.0) (Figure 2). This IR data analysis is in line with Orie et al. (2019). The 1H-NMR analysis of the product in DMSO showed peaks at 7.55 ppm, 6.60 ppm and 6.2 ppm, thus, these correspond to the furan ring. The peak at 6.40 ppm is associated with the proton around the methine group while the peak in the 5.9 ppm is assigned to proton around carbamoyl group. 13C-NMR data analysis indicates the presence of carbon from furan (154.20 ppm, 142.50 ppm, 110.7 ppm and, 106.09 ppm), imine (160.152 ppm), carbamoyl group (172.73 ppm) and methane (50.65 ppm). The meeting point analysis was at the range of 145˚C - 147˚C, the rf value in a solvent system hexane:ethylacetate (2:3) is 0.60, the percentage yield of the product was 73. These observations were in line with Orie et al. (2019).
3.3. Bioactivity of 1,1-bis(2-carbamoylguanidino)furan-2-ylmethane(CFM)
From the results presented in the tables (Tables 1-3) and figure (Figure 2) below, it was seen that CFM has an antibacterial activity on all the test pathogens with the exception of Staphylococcus aureus, given that all concentrations of it showed no inhibition zones on the pathogen (Table 1 and Figure 2).
The efficacy of this activity on the different test pathogens also varied as indicated by their MIC values (Table 3). The compound CFM showed the most activity on Escherichia coli and Salmomellatyphi because they were the organisms that had the least concentration of the CFM (1.0 mg∙L−1) as their MIC. The least susceptible pathogen however was Bacillus subtilis, which had the MIC value of 100 mg∙L−1. The synthesized CFM showed no bioactivity against Staphylococcus aureus.
Table 2 above shows Minimum Bactericidal Concentration (MBC) of CFM on Test Pathogens. The compound CFM exhibited both bactericidal and bacteriostatic properties when screened on the pathogens (Table 2). Bactericidal mode of inhibition was observed with Escherichia coli and Salmonella typhi, while bacteriostatic mode of inhibition was observed with Bacillus subtilis (Table 3).
![]()
Figure 2. Antibacterial properties of CFM on selected bacterial pathogens.
![]()
Table 1. Activity of CFM on test pathogens.
Well diameter: 5 mm. * = Minimum Inhibitory Concentration (MIC).
![]()
Table 2. Minimum bactericidal concentration (MBC) of CFM on test pathogens.
* = Minimum Bactericidal Concentration (MBC).
![]()
Table 3. MIC and MBC of CFM on test pathogens.
For Escherichia coli, the concentrations that are equal or greater than 100 mg∙L−1 will bring about the death of cells, while for Staphylococcus and Salmonella typhi, the concentrations that are equal or greater than 1.0 mg∙L−1 will bring about the death of cells. For Bacillus subtilis, the only concentration that inhibited the pathogen (100 mg∙L−1) could not bring about inhibition by cell death. Staphylococcus aureus was completely resistant to the CFM.
4. Conclusion
The compound 1,1-bis(2-carbamoylguanidino)furan-2-ylmethane have the ability to prevent metabolic growth of Escherichia coli, Salmomellatyphi and Bacillus subtilis to different extent and is inactive on Staphylococcus aureus. Due to this broad spectrum of activity, 1,1-bis(2-carbamoylguanidino)furan-2-ylmethane can be used for various medicinal purposes and is therefore encouraged. The presences of bioactive moieties and pharmacological activities have proved the potency of furfural derivatives in the development of novel drug in future.