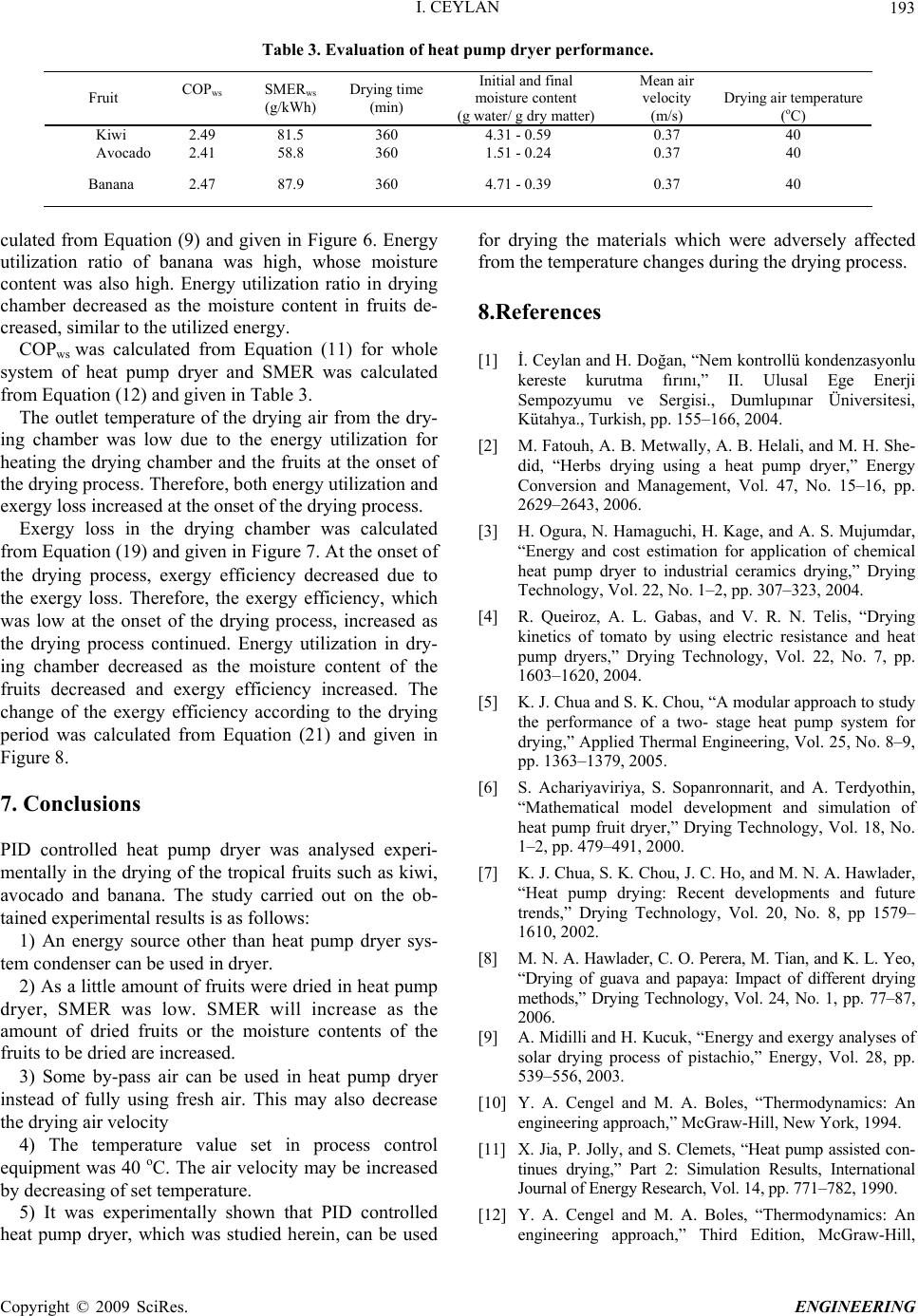
I. CEYLAN 193
Table 3. Evaluation of heat pump dryer performance.
Fruit COPws
SMERws
(g/kWh)
Drying time
(min)
Initial and final
moisture content
(g water/ g dry matter)
Mean air
velocity
(m/s)
Drying air temperature
(oC)
Kiwi 2.49 81.5 360 4.31 - 0.59 0.37 40
Avocado 2.41 58.8 360 1.51 - 0.24 0.37 40
Banana 2.47 87.9 360 4.71 - 0.39 0.37 40
culated from Equation (9) and given in Figure 6. Energy
utilization ratio of banana was high, whose moisture
content was also high. Energy utilization ratio in drying
chamber decreased as the moisture content in fruits de-
creased, similar to the utilized energy.
COPws was calculated from Equation (11) for whole
system of heat pump dryer and SMER was calculated
from Equation (12) and given in Table 3.
The outlet temperature of the drying air from the dry-
ing chamber was low due to the energy utilization for
heating the drying chamber and the fruits at the onset of
the drying process. Therefore, both energy utilization and
exergy loss increased at the onset of the drying process.
Exergy loss in the drying chamber was calculated
from Equation (19) and given in Figure 7. At the onset of
the drying process, exergy efficiency decreased due to
the exergy loss. Therefore, the exergy efficiency, which
was low at the onset of the drying process, increased as
the drying process continued. Energy utilization in dry-
ing chamber decreased as the moisture content of the
fruits decreased and exergy efficiency increased. The
change of the exergy efficiency according to the drying
period was calculated from Equation (21) and given in
Figure 8.
7. Conclusions
PID controlled heat pump dryer was analysed experi-
mentally in the drying of the tropical fruits such as kiwi,
avocado and banana. The study carried out on the ob-
tained experimental results is as follows:
1) An energy source other than heat pump dryer sys-
tem condenser can be used in dryer.
2) As a little amount of fruits were dried in heat pump
dryer, SMER was low. SMER will increase as the
amount of dried fruits or the moisture contents of the
fruits to be dried are increased.
3) Some by-pass air can be used in heat pump dryer
instead of fully using fresh air. This may also decrease
the drying air velocity
4) The temperature value set in process control
equipment was 40 oC. The air velocity may be increased
by decreasing of set temperature.
5) It was experimentally shown that PID controlled
heat pump dryer, which was studied herein, can be used
for drying the materials which were adversely affected
from the temperature changes during the drying process.
8.References
[1] İ. Ceylan and H. Doğan, “Nem kontrollü kondenzasyonlu
kereste kurutma fırını,” II. Ulusal Ege Enerji
Sempozyumu ve Sergisi., Dumlupınar Üniversitesi,
Kütahya., Turkish, pp. 155–166, 2004.
[2] M. Fatouh, A. B. Metwally, A. B. Helali, and M. H. She-
did, “Herbs drying using a heat pump dryer,” Energy
Conversion and Management, Vol. 47, No. 15–16, pp.
2629–2643, 2006.
[3] H. Ogura, N. Hamaguchi, H. Kage, and A. S. Mujumdar,
“Energy and cost estimation for application of chemical
heat pump dryer to industrial ceramics drying,” Drying
Technology, Vol. 22, No. 1–2, pp. 307–323, 2004.
[4] R. Queiroz, A. L. Gabas, and V. R. N. Telis, “Drying
kinetics of tomato by using electric resistance and heat
pump dryers,” Drying Technology, Vol. 22, No. 7, pp.
1603–1620, 2004.
[5] K. J. Chua and S. K. Chou, “A modular approach to study
the performance of a two- stage heat pump system for
drying,” Applied Thermal Engineering, Vol. 25, No. 8–9,
pp. 1363–1379, 2005.
[6] S. Achariyaviriya, S. Sopanronnarit, and A. Terdyothin,
“Mathematical model development and simulation of
heat pump fruit dryer,” Drying Technology, Vol. 18, No.
1–2, pp. 479–491, 2000.
[7] K. J. Chua, S. K. Chou, J. C. Ho, and M. N. A. Hawlader,
“Heat pump drying: Recent developments and future
trends,” Drying Technology, Vol. 20, No. 8, pp 1579–
1610, 2002.
[8] M. N. A. Hawlader, C. O. Perera, M. Tian, and K. L. Yeo,
“Drying of guava and papaya: Impact of different drying
methods,” Drying Technology, Vol. 24, No. 1, pp. 77–87,
2006.
[9] A. Midilli and H. Kucuk, “Energy and exergy analyses of
solar drying process of pistachio,” Energy, Vol. 28, pp.
539–556, 2003.
[10] Y. A. Cengel and M. A. Boles, “Thermodynamics: An
engineering approach,” McGraw-Hill, New York, 1994.
[11] X. Jia, P. Jolly, and S. Clemets, “Heat pump assisted con-
tinues drying,” Part 2: Simulation Results, International
Journal of Energy Research, Vol. 14, pp. 771–782, 1990.
[12] Y. A. Cengel and M. A. Boles, “Thermodynamics: An
engineering approach,” Third Edition, McGraw-Hill,
C
opyright © 2009 SciRes. ENGINEERING