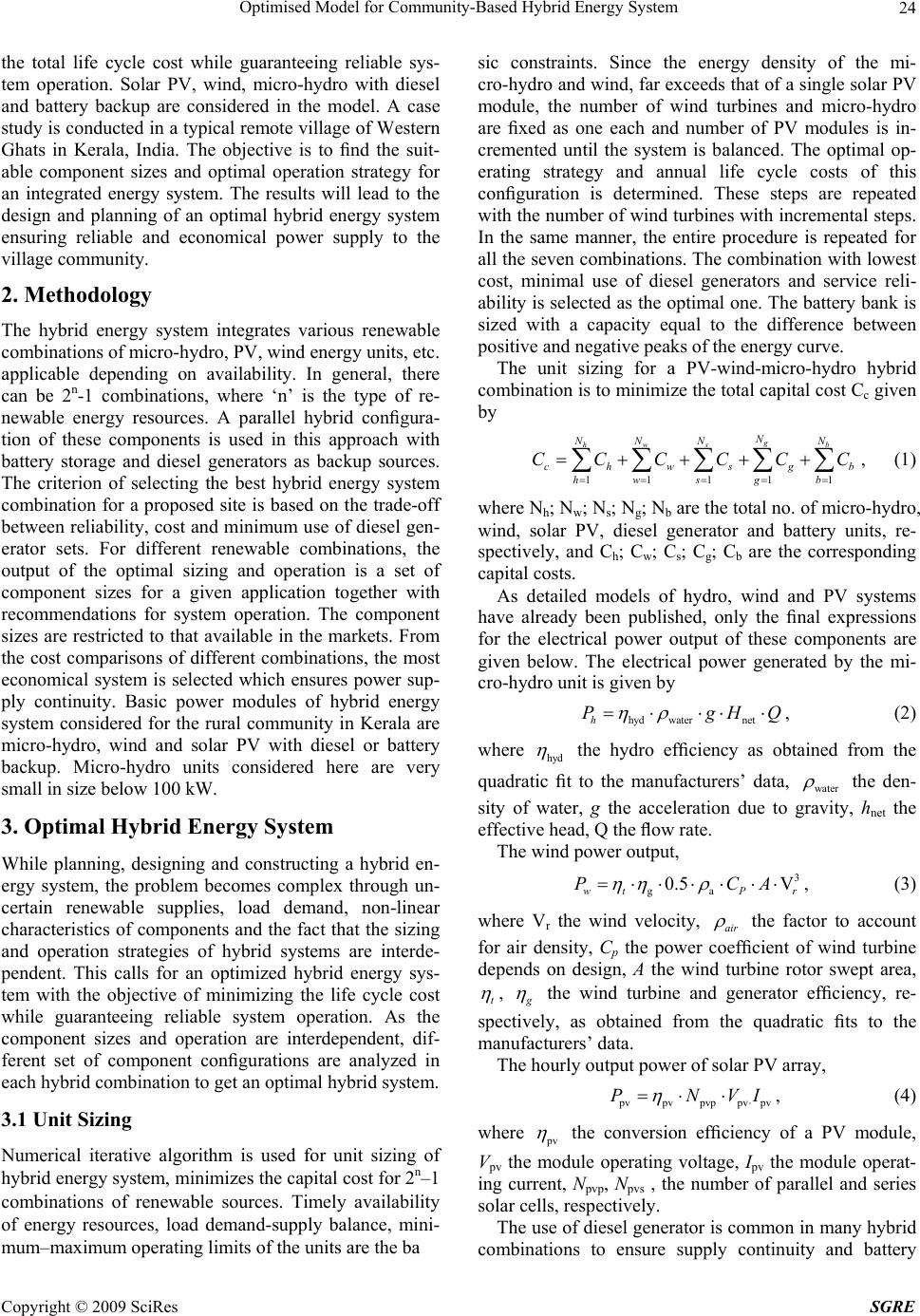
Optimised Model for Community-Based Hybrid Energy System 24
the total life cycle cost while guaranteeing reliable sys-
tem operation. Solar PV, wind, micro-hydro with diesel
and battery backup are considered in the model. A case
study is conducted in a typical remote village of Western
Ghats in Kerala, India. The objective is to find the suit-
able component sizes and optimal operation strategy for
an integrated energy system. The results will lead to the
design and planning of an optimal hybrid energy system
ensuring reliable and economical power supply to the
village community.
2. Methodology
The hybrid energy system integrates various renewable
combinations of micro-hydro, PV, wind energy units, etc.
applicable depending on availability. In general, there
can be 2n-1 combinations, where ‘n’ is the type of re-
newable energy resources. A parallel hybrid configura-
tion of these components is used in this approach with
battery storage and diesel generators as backup sources.
The criterion of selecting the best hybrid energy system
combination for a proposed site is based on the trade-off
between reliability, cost and minimum use of diesel gen-
erator sets. For different renewable combinations, the
output of the optimal sizing and operation is a set of
component sizes for a given application together with
recommendations for system operation. The component
sizes are restricted to that available in the markets. From
the cost comparisons of different combinations, the most
economical system is selected which ensures power sup-
ply continuity. Basic power modules of hybrid energy
system considered for the rural community in Kerala are
micro-hydro, wind and solar PV with diesel or battery
backup. Micro-hydro units considered here are very
small in size below 100 kW.
3. Optimal Hybrid Energy System
While planning, designing and constructing a hybrid en-
ergy system, the problem becomes complex through un-
certain renewable supplies, load demand, non-linear
characteristics of components and the fact that the sizing
and operation strategies of hybrid systems are interde-
pendent. This calls for an optimized hybrid energy sys-
tem with the objective of minimizing the life cycle cost
while guaranteeing reliable system operation. As the
component sizes and operation are interdependent, dif-
ferent set of component configurations are analyzed in
each hybrid combination to get an optimal hybrid system.
3.1 Unit Sizing
Numerical iterative algorithm is used for unit sizing of
hybrid energy system, minimizes the capital cost for 2n–1
combinations of renewable sources. Timely availability
of energy resources, load demand-supply balance, mini-
mum–maximum operating limits of the units are the ba
sic constraints. Since the energy density of the mi-
cro-hydro and wind, far exceeds that of a single solar PV
module, the number of wind turbines and micro-hydro
are fixed as one each and number of PV modules is in-
cremented until the system is balanced. The optimal op-
erating strategy and annual life cycle costs of this
configuration is determined. These steps are repeated
with the number of wind turbines with incremental steps.
In the same manner, the entire procedure is repeated for
all the seven combinations. The combination with lowest
cost, minimal use of diesel generators and service reli-
ability is selected as the optimal one. The battery bank is
sized with a capacity equal to the difference between
positive and negative peaks of the energy curve.
The unit sizing for a PV-wind-micro-hydro hybrid
combination is to minimize the total capital cost Cc given
by
11111
g
hw sb
N
NN NN
chwsg
hw sg b
CCCCC
b
C
, (1)
where Nh; Nw; Ns; Ng; Nb are the total no. of micro-hydro,
wind, solar PV, diesel generator and battery units, re-
spectively, and Ch; Cw; Cs; Cg; Cb are the corresponding
capital costs.
As detailed models of hydro, wind and PV systems
have already been published, only the final expressions
for the electrical power output of these components are
given below. The electrical power generated by the mi-
cro-hydro unit is given by
hyd waterneth
PgHQ
, (2)
where hyd
the hydro efficiency as obtained from the
quadratic fit to the manufacturers’ data, water
the den-
sity of water, g the acceleration due to gravity, hnet the
effective head, Q the flow rate.
The wind power output,
3
ga
0.5 V
wt P
PC
r
A
, (3)
where Vr the wind velocity, air
the factor to account
for air density, Cp the power coefficient of wind turbine
depends on design, A the wind turbine rotor swept area,
t
,
the wind turbine and generator efficiency, re-
spectively, as obtained from the quadratic fits to the
manufacturers’ data.
The hourly output power of solar PV array,
pvpvpvppv pv
PNVI
, (4)
where pv
the conversion efficiency of a PV module,
Vpv the module operating voltage, Ipv the module operat-
ing current, Npvp, Npvs , the number of parallel and series
solar cells, respectively.
The use of diesel generator is common in many hybrid
combinations to ensure supply continuity and battery
Copyright © 2009 SciRes SGRE