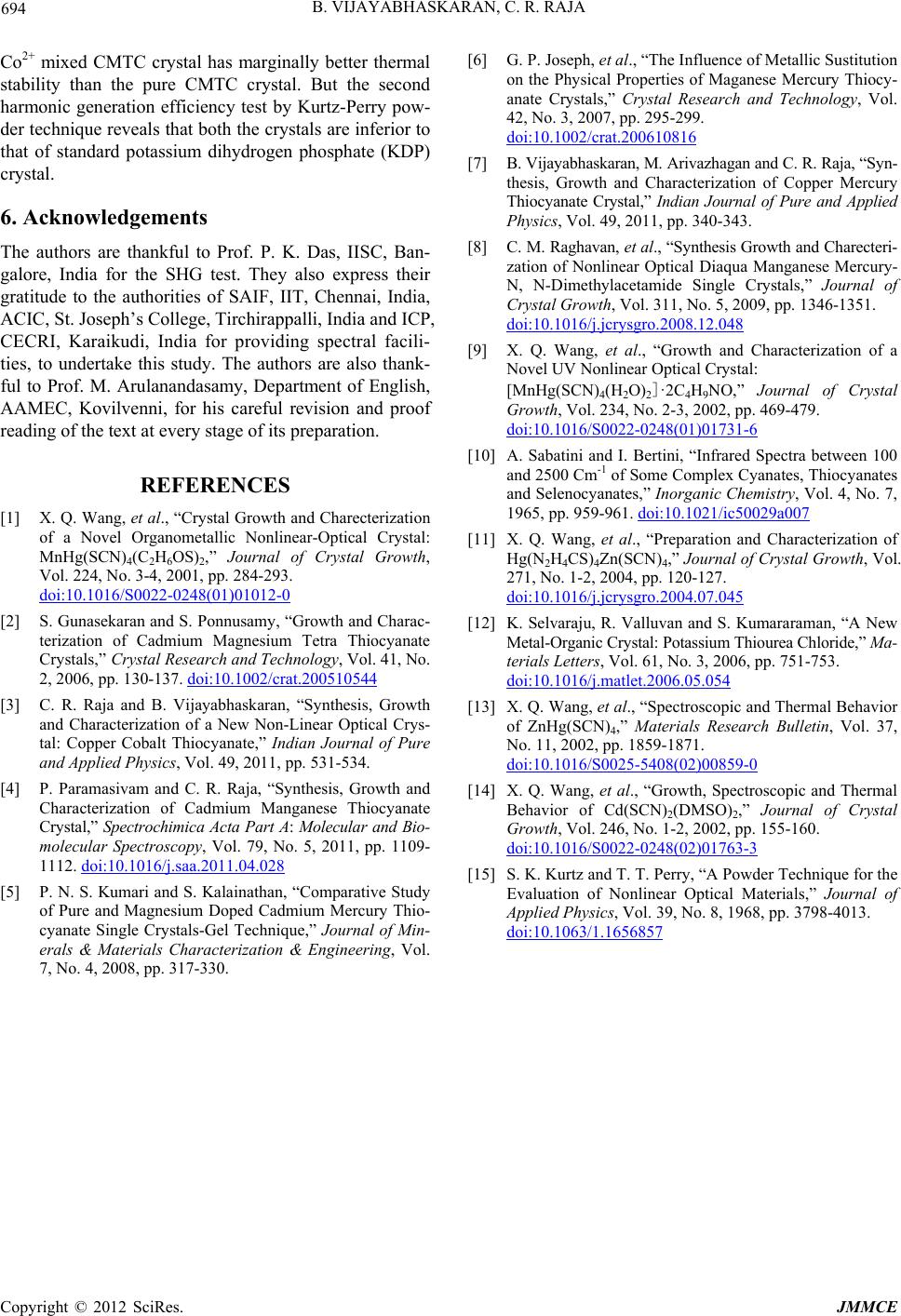
B. VIJAYABHASKARAN, C. R. RAJA
Copyright © 2012 SciRes. JMMCE
694
Co2+ mixed CMTC crystal has marginally better thermal
stability than the pure CMTC crystal. But the second
harmonic generation efficiency test by Kurtz-Perry pow-
der technique reveals that both the crystals are inferior to
that of standard potassium dihydrogen phosphate (KDP)
crystal.
6. Acknowledgements
The authors are thankful to Prof. P. K. Das, IISC, Ban-
galore, India for the SHG test. They also express their
gratitude to the authorities of SAIF, IIT, Chennai, India,
ACIC, St. Joseph’s College, Tirchirappalli, India and ICP,
CECRI, Karaikudi, India for providing spectral facili-
ties, to undertake this study. The authors are also thank-
ful to Prof. M. Arulanandasamy, Department of English,
AAMEC, Kovilvenni, for his careful revision and proof
reading of the text at every stage of its preparation.
REFERENCES
[1] X. Q. Wang, et al., “Crystal Growth and Charecterization
of a Novel Organometallic Nonlinear-Optical Crystal:
MnHg(SCN)4(C2H6OS)2,” Journal of Crystal Growth,
Vol. 224, No. 3-4, 2001, pp. 284-293.
doi:10.1016/S0022-0248(01)01012-0
[2] S. Gunasekaran and S. Ponnusamy, “Growth and Charac-
terization of Cadmium Magnesium Tetra Thiocyanate
Crystals,” Crystal Research and Technology, Vol. 41, No.
2, 2006, pp. 130-137. doi:10.1002/crat.200510544
[3] C. R. Raja and B. Vijayabhaskaran, “Synthesis, Growth
and Characterization of a New Non-Linear Optical Crys-
tal: Copper Cobalt Thiocyanate,” Indian Journal of Pure
and Applied Physics, Vol. 49, 2011, pp. 531-534.
[4] P. Paramasivam and C. R. Raja, “Synthesis, Growth and
Characterization of Cadmium Manganese Thiocyanate
Crystal,” Spectrochimica Acta Part A: Molecular and Bio-
molecular Spectroscopy, Vol. 79, No. 5, 2011, pp. 1109-
1112. doi:10.1016/j.saa.2011.04.028
[5] P. N. S. Kumari and S. Kalainathan, “Comparative Study
of Pure and Magnesium Doped Cadmium Mercury Thio-
cyanate Single Crystals-Gel Technique,” Journal of Min-
erals & Materials Characterization & Engineering, Vol.
7, No. 4, 2008, pp. 317-330.
[6] G. P. Joseph, et al., “The Influence of Metallic Sustitution
on the Physical Properties of Maganese Mercury Thiocy-
anate Crystals,” Crystal Research and Technology, Vol.
42, No. 3, 2007, pp. 295-299.
doi:10.1002/crat.200610816
[7] B. Vijayabhaskaran, M. Arivazhagan and C. R. Raja, “Syn-
thesis, Growth and Characterization of Copper Mercury
Thiocyanate Crystal,” Indian Journal of Pure and Applied
Physics, Vol. 49, 2011, pp. 340-343.
[8] C. M. Raghavan, et al., “Synthesis Growth and Charecteri-
zation of Nonlinear Optical Diaqua Manganese Mercury-
N, N-Dimethylacetamide Single Crystals,” Journal of
Crystal Growth, Vol. 311, No. 5, 2009, pp. 1346-1351.
doi:10.1016/j.jcrysgro.2008.12.048
[9] X. Q. Wang, et al., “Growth and Characterization of a
Novel UV Nonlinear Optical Crystal:
[MnHg(SCN)4(H2O)2]·2C4H9NO,” Journal of Crystal
Growth, Vol. 234, No. 2-3, 2002, pp. 469-479.
doi:10.1016/S0022-0248(01)01731-6
[10] A. Sabatini and I. Bertini, “Infrared Spectra between 100
and 2500 Cm-1 of Some Complex Cyanates, Thiocyanates
and Selenocyanates,” Inorganic Chemistry, Vol. 4, No. 7,
1965, pp. 959-961. doi:10.1021/ic50029a007
[11] X. Q. Wang, et al., “Preparation and Characterization of
Hg(N2H4CS)4Zn(SCN)4,” Journal of Crystal Growth, Vol.
271, No. 1-2, 2004, pp. 120-127.
doi:10.1016/j.jcrysgro.2004.07.045
[12] K. Selvaraju, R. Valluvan and S. Kumararaman, “A New
Metal-Organic Crystal: Potassium Thiourea Chloride,” Ma-
terials Letters, Vol. 61, No. 3, 2006, pp. 751-753.
doi:10.1016/j.matlet.2006.05.054
[13] X. Q. Wang, et al., “Spectroscopic and Thermal Behavior
of ZnHg(SCN)4,” Materials Research Bulletin, Vol. 37,
No. 11, 2002, pp. 1859-1871.
doi:10.1016/S0025-5408(02)00859-0
[14] X. Q. Wang, et al., “Growth, Spectroscopic and Thermal
Behavior of Cd(SCN)2(DMSO)2,” Journal of Crystal
Growth, Vol. 246, No. 1-2, 2002, pp. 155-160.
doi:10.1016/S0022-0248(02)01763-3
[15] S. K. Kurtz and T. T. Perry, “A Powder Technique for the
Evaluation of Nonlinear Optical Materials,” Journal of
Applied Physics, Vol. 39, No. 8, 1968, pp. 3798-4013.
doi:10.1063/1.1656857