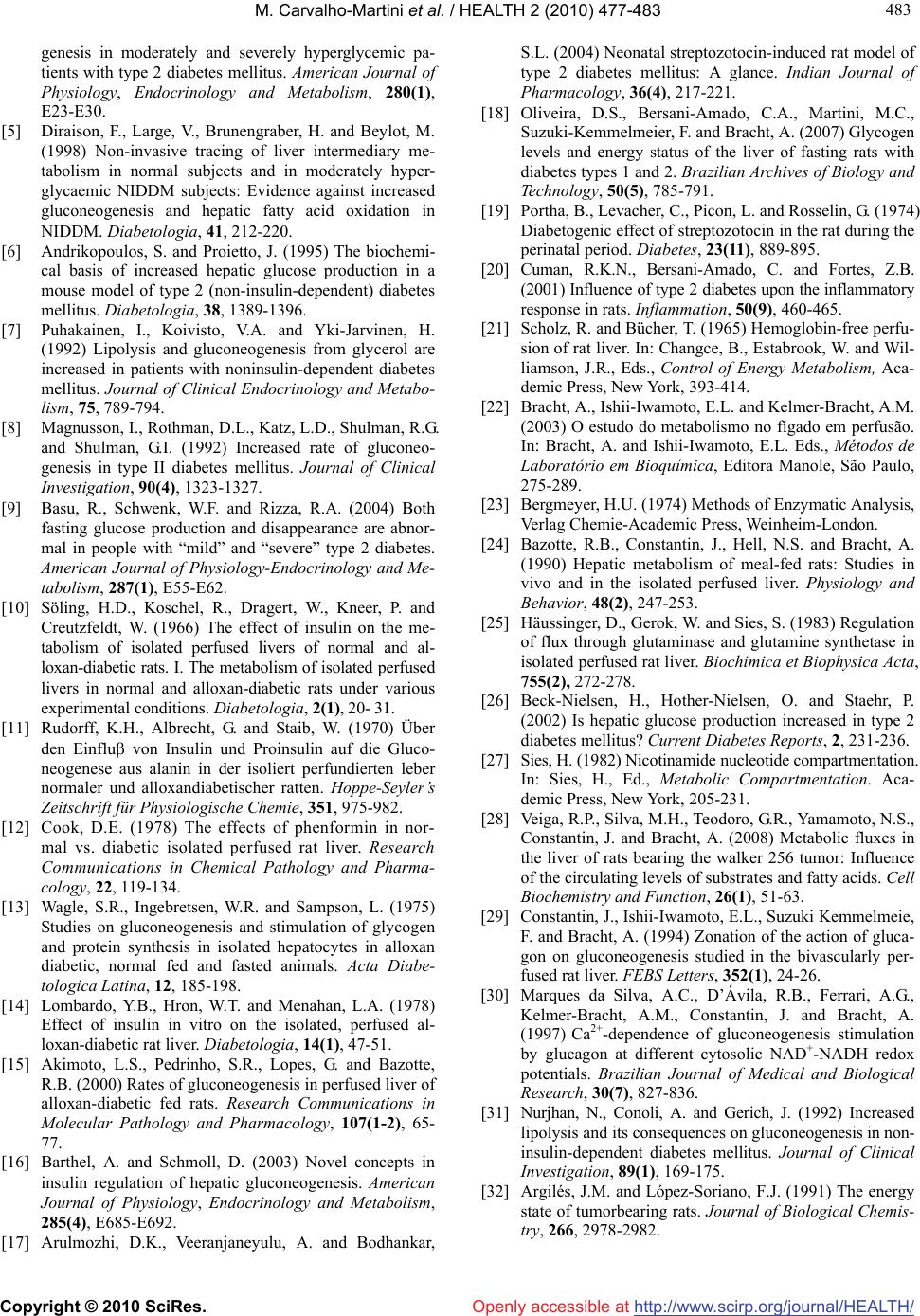
M. Carvalho-Martini et al. / HEALTH 2 (2010) 477-483
Copyright © 2010 SciRes. http://www.scirp.org/journal/HEALTH/Openly accessible at
483
483
genesis in moderately and severely hyperglycemic pa-
tients with type 2 diabetes mellitus. American Journal of
Physiology, Endocrinology and Metabolism, 280(1),
E23-E30.
[5] Diraison, F., Large, V., Brunengraber, H. and Beylot, M.
(1998) Non-invasive tracing of liver intermediary me-
tabolism in normal subjects and in moderately hyper-
glycaemic NIDDM subjects: Evidence against increased
gluconeogenesis and hepatic fatty acid oxidation in
NIDDM. Diabetologia, 41, 212-220.
[6] Andrikopoulos, S. and Proietto, J. (1995) The biochemi-
cal basis of increased hepatic glucose production in a
mouse model of type 2 (non-insulin-dependent) diabetes
mellitus. Diabetologia, 38, 1389-1396.
[7] Puhakainen, I., Koivisto, V.A. and Yki-Jarvinen, H.
(1992) Lipolysis and gluconeogenesis from glycerol are
increased in patients with noninsulin-dependent diabetes
mellitus. Journal of Clinical Endocrinology and Metabo-
lism, 75, 789-794.
[8] Magnusson, I., Rothman, D.L., Katz, L.D., Shulman, R.G.
and Shulman, G.I. (1992) Increased rate of gluconeo-
genesis in type II diabetes mellitus. Journal of Clinical
Investigation, 90(4), 1323-1327.
[9] Basu, R., Schwenk, W.F. and Rizza, R.A. (2004) Both
fasting glucose production and disappearance are abnor-
mal in people with “mild” and “severe” type 2 diabetes.
American Journal of Physiology-Endocrinology and Me-
tabolism, 287(1), E55-E62.
[10] Söling, H.D., Koschel, R., Dragert, W., Kneer, P. and
Creutzfeldt, W. (1966) The effect of insulin on the me-
tabolism of isolated perfused livers of normal and al-
loxan-diabetic rats. I. The metabolism of isolated perfused
livers in normal and alloxan-diabetic rats under various
experimental conditions. Diabetologia, 2(1), 20- 31.
[11] Rudorff, K.H., Albrecht, G. and Staib, W. (1970) Über
den Einflu von Insulin und Proinsulin auf die Gluco-
neogenese aus alanin in der isoliert perfundierten leber
normaler und alloxandiabetischer ratten. Hoppe-Seyler’s
Zeitschrift für Physiologische Chemie, 351, 975-982.
[12] Cook, D.E. (1978) The effects of phenformin in nor-
mal vs. diabetic isolated perfused rat liver. Research
Communications in Chemical Pathology and Pharma-
cology, 22, 119-134.
[13] Wagle, S.R., Ingebretsen, W.R. and Sampson, L. (1975)
Studies on gluconeogenesis and stimulation of glycogen
and protein synthesis in isolated hepatocytes in alloxan
diabetic, normal fed and fasted animals. Acta Diabe-
tologica Latina, 12, 185-198.
[14] Lombardo, Y.B., Hron, W.T. and Menahan, L.A. (1978)
Effect of insulin in vitro on the isolated, perfused al-
loxan-diabetic rat liver. Diabetologia, 14(1), 47-51.
[15] Akimoto, L.S., Pedrinho, S.R., Lopes, G. and Bazotte,
R.B. (2000) Rates of gluconeogenesis in perfused liver of
alloxan-diabetic fed rats. Research Communications in
Molecular Pathology and Pharmacology, 107(1-2), 65-
77.
[16] Barthel, A. and Schmoll, D. (2003) Novel concepts in
insulin regulation of hepatic gluconeogenesis. American
Journal of Physiology, Endocrinology and Metabolism,
285(4), E685-E692.
[17] Arulmozhi, D.K., Veeranjaneyulu, A. and Bodhankar,
S.L. (2004) Neonatal streptozotocin-induced rat model of
type 2 diabetes mellitus: A glance. Indian Journal of
Pharmacology, 36(4), 217-221.
[18] Oliveira, D.S., Bersani-Amado, C.A., Martini, M.C.,
Suzuki-Kemmelmeier, F. and Bracht, A. (2007) Glycogen
levels and energy status of the liver of fasting rats with
diabetes types 1 and 2. Brazilian Archives of Biology and
Technology, 50(5), 785-791.
[19] Portha, B., Levacher, C., Picon, L. and Rosselin, G. (1974)
Diabetogenic effect of streptozotocin in the rat during the
perinatal period. Diabetes, 23(11), 889-895.
[20] Cuman, R.K.N., Bersani-Amado, C. and Fortes, Z.B.
(2001) Influence of type 2 diabetes upon the inflammatory
response in rats. Inflammation, 50(9), 460-465.
[21] Scholz, R. and Bücher, T. (1965) Hemoglobin-free perfu-
sion of rat liver. In: Changce, B., Estabrook, W. and Wil-
liamson, J.R., Eds., Control of Energy Metabolism, Aca-
demic Press, New York, 393-414.
[22] Bracht, A., Ishii-Iwamoto, E.L. and Kelmer-Bracht, A.M.
(2003) O estudo do metabolismo no fígado em perfusão.
In: Bracht, A. and Ishii-Iwamoto, E.L. Eds., Métodos de
Laboratório em Bioquímica, Editora Manole, São Paulo,
275-289.
[23] Bergmeyer, H.U. (1974) Methods of Enzymatic Analysis,
Verlag Chemie-Academic Press, Weinheim-London.
[24] Bazotte, R.B., Constantin, J., Hell, N.S. and Bracht, A.
(1990) Hepatic metabolism of meal-fed rats: Studies in
vivo and in the isolated perfused liver. Physiology and
Behavior, 48(2), 247-253.
[25] Häussinger, D., Gerok, W. and Sies, S. (1983) Regulation
of flux through glutaminase and glutamine synthetase in
isolated perfused rat liver. Biochimica et Biophysica Acta,
755(2), 272-278.
[26] Beck-Nielsen, H., Hother-Nielsen, O. and Staehr, P.
(2002) Is hepatic glucose production increased in type 2
diabetes mellitus? Current Diabetes Reports, 2, 231-236.
[27] Sies, H. (1982) Nicotinamide nucleotide compartmentation.
In: Sies, H., Ed., Metabolic Compartmentation. Aca-
demic Press, New York, 205-231.
[28] Veiga, R.P., Silva, M.H., Teodoro, G.R., Yamamoto, N.S.,
Constantin, J. and Bracht, A. (2008) Metabolic fluxes in
the liver of rats bearing the walker 256 tumor: Influence
of the circulating levels of substrates and fatty acids. Cell
Biochemistry and Function, 26(1), 51-63.
[29] Constantin, J., Ishii-Iwamoto, E.L., Suzuki Kemmelmeie,
F. and Bracht, A. (1994) Zonation of the action of gluca-
gon on gluconeogenesis studied in the bivascularly per-
fused rat liver. FEBS Letters, 352(1), 24-26.
[30] Marques da Silva, A.C., D’Ávila, R.B., Ferrari, A.G.,
Kelmer-Bracht, A.M., Constantin, J. and Bracht, A.
(1997) Ca2+-dependence of gluconeogenesis stimulation
by glucagon at different cytosolic NAD+-NADH redox
potentials. Brazilian Journal of Medical and Biological
Research, 30(7), 827-836.
[31] Nurjhan, N., Conoli, A. and Gerich, J. (1992) Increased
lipolysis and its consequences on gluconeogenesis in non-
insulin-dependent diabetes mellitus. Journal of Clinical
Investigation, 89(1), 169-175.
[32] Argilés, J.M. and López-Soriano, F.J. (1991) The energy
state of tumorbearing rats. Journal of Biological Chemis-
try, 266, 2978-2982.