Enigma of Alluvial Gold Mining in Pre-Contact Peru—The Present Is Key to the Past ()
1. Introduction
The ore of mercury, cinnabar (HgS), is a sulfide mineral that was widely used in the ancient world: 1) it was mined, selectively ground, and widely used as a blood-red pigment (vermilion) on ceramics, gold masks, murals, statues, burial rites ( Petersen, 1970/2010 ; Bonavia, 1985 ; Brooks et al., 2008 ; Spindler, 2018 ) and, 2) it was retorted to produce mercury ( Cabrera la Rosa, 1954 ; Craddock, 1995 ; Brooks, 2012 ). Retorting cinnabar to obtain mercury has been documented more than 8000 years ago in ancient Türkiye ( Barnes & Bailey, 1972 ; Brooks et al., 2017 ); during Roman time (Pliny the Elder, 77 AD); the Middle East ( Al-Hassan & Hill, 1986 ), in ancient Mexico ( Langenscheidt, 1986 ); medieval Europe ( Agricola, 1556/1912 ); California in the 1840s ( Bailey & Everhart, 1964 ); and present-day Indonesia ( Paddock, 2019 ). Mercury has been used to recover gold by amalgamation with mercury for centuries ( Ahern, 2016 ; Fernández-Lozano et al., 2021 ).
The most important present-day use of mercury world-wide is for small-scale alluvial gold mining ( United Nations Environment Programme, 2017 ). Other uses include: auto switches, batteries, chlor-alkali production, dental amalgam, explosives, fluorescent lamps, light-up toys, medical equipment, medicines, mirror-backing, and neon lights. Therefore, since cinnabar was mined and retorted in the past, and none of the above uses existed in the past, it is logical to conclude that mercury mined in the past had but one use-amalgamation of alluvial gold.
Alluvial gold is known to have been the main source of gold for ancient man and provided two-thirds of the gold ever produced ( Boyle, 1979 ; Marsden & House, 2006 ). In Peru, alluvial gold deposits are widespread; however, the most well-known districts include Marañon in northeastern Peru, Rio Huallaga in east-central Peru, and Madre de Dios in southeastern Peru ( Noble & Vidal, 1994 ; Atlas, 1999 ). These alluvial gold sources likely provided much of the gold used by Atahualpa as ransom for his release from the Spanish before his execution in 1533.
Peru was the leading gold producer in Latin America in 2018 and has produced metals for thousands of years ( Burger & Gordon, 1998 ). For example, gold foils and gold workers tools were reported from Waywanka, a site dated 6000 - 1800 BCE ( Grossman, 2013 ). In 2018, Peru’s large-scale open-pit gold mines produced 123,767 kg of gold using cyanide and 18,875 kg of gold were produced from small-scale alluvial gold mines that used only gravity separation and mercury ( Soto-Viruet, 2018 ). Therefore, since cyanide use only dates to 1880s, mercury amalgamation must be considered as the indigenous mining technology that produced industrial amounts of gold in ancient Peru.
2. Gold Mining Methods
Even though alluvial gold is widely acknowledged as a source of pre-contact gold, Saenz & Martinon-Torres (2011) indicate that little is known about gold mining methods in the past. However, Charles Lyell’s (1830) Principle of Uniformitarianism or “The Present is Key to the Past” leads to understanding pre-contact gold mining by study of gold mining methods that are used today.
For example, in California in the 1850s, the importance of mercury in alluvial gold mining is indicated by the production of approximately 26,000,000 pounds (or 342,000 seventy-six-pound flasks) of mercury from New Almaden and other California mercury mines. This mercury was essential for amalgamation of alluvial gold produced from hydraulic mining during the California Gold Rush in 1849 ( Davis, 1957 ; Bailey & Everhart, 1964 ; Bailey et al., 1973 ; Lanyon & Bulmore, 1967 ; Alpers et al., 2005 ). Similarly, mercury produced from Alaskan cinnabar mines was used for the Klondike Gold Rush in 1896 ( National Park Service, 2019 ). Mercury is used today to produce alluvial gold in Brazil, Colombia, Ecuador, Ghana, Indonesia, Peru, Venezuela, and many other countries.
Present-day gold mining methods are key to gold mining in the past. For example, mercury amalgamation, which is used widely today in Peru, was also used to amalgamate placer gold at the ancient Roman gold mines in Spain ( Fernández-Lozano et al., 2021 ). Specifically, industrial amounts of gold are produced in only two ways: 1) gravity separation, first by washing and then addition of mercury (amalgamation) and 2) cyanide ( Craig et al., 2001 ). Gravity separation is the oldest method and utilizes the high specific gravity of the gold (~19) first in water and then with mercury. Sparse native gold found in streams ( Petersen, 1970/ 2010, Tables 2, 3 ) could easily be removed by hand or, as in the ancient Andes, washing in water using a wooden gold pan or “batea” provided a concentrate of the fine-grained, heavier, gold-bearing sediment. Lighter material was washed out of the gold pan. The gold flakes or “chispitas” which are mm-sized and smaller, can only be efficiently recovered from the washed mineral separate using the ages-old method of mercury amalgamation. This begins with initial gravity separation by washing the gold-bearing sediment to produce a “pay dirt” concentrate of black sand or “arena negra” that includes the mm-sized and smaller gold flakes as well as apatite, magnetite, zircon, and other heavy minerals. Mercury is then added to the heavy-mineral concentrate and selectively removes only the gold. Then, this silvery, gold-mercury amalgam clot is squeezed through a cloth to recover excess mercury. Finally, this amalgam is burned or “refogado” to volatilize the remaining mercury. This leaves a sponge-textured anthropogenic gold “nugget” that could then be used for artifact production. To extract a given amount of alluvial gold, approximately twice as much mercury is required ( Roskill, 1990 ; Cánepa, 2005 ).
Gravity separation techniques include the legendary Golden Fleece, as well as other variances that use carpet, sheep or other animal skins, and rely on the high specific gravity of gold to concentrate the gold in water ( Healy, 1979 ). Another is the aventadero method that uses wind to separate the heavier gold from the lighter minerals, much like grain is separated from chaff ( Petersen, 1970/2010 ). In Chocó, Colombia, plant juices added to water are used in place of mercury to concentrate the gold ( Brooks et al., 2015 ) and in the Philippines, borax is used as a flux, to concentrate the heavier gold in the bottom of a heated crucible ( Appel & Na-Oy, 2012 ).
Cyanide is the newer and more efficient method; however, its use only dates to the 1880s. Metals such as gold and silver are removed in solution from the crushed ore from large-scale, hard-rock porphyry (Au-Cu-Ag) mines. In precontact Peru, surficial outcrops of porphyry ores would have provided a gold-copper-silver alloy known as “tumbaga”, which, through depletion gilding, would have resulted in enhanced surficial gold ( Petersen, 1970/2010 ).
As an example of the importance of cyanide in mining, alluvial gold was discovered in Aruba in 1824. The gold was successfully recovered from alluvial and vein deposits using crushing, gravity separation, and mercury amalgamation until ~1880 when profits declined and mining ceased. Then, in 1897 the cyanide method was introduced, mining resumed profitably, and continued until 1916 ( Gold Mine Ranch, 2021 ).
3. Previous Work
The enigma of pre-contact gold production in the ancient Andes was first addressed by the scholar Márques (1590) who said “Lavaban la tierra en bateas de madera hasta obtener un residuo que contenía el oro. Los indios son más expertos para sacar el oro por ser naturales de este tierra así por la experiencia que ellos tenian como por la noticia de sus antepasados cuya voz corre en ellos.” [They wash the sediments in wooden gold pans until a gold-bearing concentrate is obtained. The indigenous people are experts at getting gold because they are native to these lands and because the experience of their ancestors guides them.] Historian Garcilaso de la Vega wrote that “Gold was gathered by the Incas from the streams….no idea of the virtues of mercury” ( Prescott, 1847/2005 ). However, while it is certain that ancient Andean gold was produced from alluvial sources, de la Vega’s comment regarding mercury is equivocal:
· Given, the tons of gold mined and used for artifacts such as backflaps, crowns, and masks that were produced in pre-contact Peru and the availability of cinnabar-mercury occurrences, Posnansky (1945/1957) proposed the use of cinnabar as a source of mercury for amalgamation of the fine-grained alluvial gold in the ancient Andes.
· Larco Hoyle (1945/2001) wrote that “...el beneficio de oro es todavía primitivo…incluyendo el empleo del azogue, que fue usado desde muy remota antigüedad.” [...gold mining is still primitive…including the use of mercury which was used since very ancient times.] Mercury is sold as azogue (from the Arabic “azzáuq” or quicksilver, or from agogae indicating where gold was washed and recovered at Las Médulas, the ancient Roman gold mines in Spain) which was the word used for mercury produced at the Almaden mercury mine during the Moorish occupation of Spain and suggests Spanish origin for the mercury. In the present-day small-scale mining areas in Peru, mercury or “mercurio” is also be sold with a brand name suggestive of the supposedly superior Spanish commodity, for example, “Mercurio El Español” ( Brooks et al., 2007 ).
· Cabrera la Rosa (1954) said: “Asimismo es posible suponer que los peruanos de aquellas tierras conocián, ya en épocas remotas, el método de la amalgamación, empleando para ello el azogue que lograban obtener del cinabrio cuyas menas existián en Buldibuyo.” [It is possible that ancient Peruvians knew about the use of mercury amalgamation in ancient time and they used mercury obtained from cinnabar ore found near Buldibuyo.] Ravines (1978) refined Cabrera la Rosa’s location and indicated that “…azogue se encuentra en Buldibuyo al pie del gran nevado de Pelagatos.” […mercury can be found at Buldibuyo at the base of great snow-covered Pelegatos peak.]
· Petersen (1970/2010) sketched several ancient quimbaletes which are 1 - 2 m, 1-2 t, crescent-shaped stones that pulverize the gold-bearing ore as they are rocked back and forth on a stone base. Petersen (1970/2010, Figure 10) shows several quimbalates that were used in pre-contact Peru and also photographed a modern quimbalete ( Petersen, 1970/2010, Figure 2 ) in use. Mercury is added to the watery slurry at the base and the weight and rocking movement of the quimbalete amalgamates the fine-grained gold and mercury. The amalgam is recovered for refogado and recovery of the gold ( Atlas, 2000 ; Cánepa, 2005 ). Therefore, since mercury is used with the present-day quimbalete, it is logical to conclude that mercury was available and similarly used for gold amalgamation in the past using the ancient quimbaletes.
· Petersen (1970/2010) wrote “…data suggest that mercury was retorted from cinnabar” and his spectrographic analyses of alluvial gold ( Petersen, 1970/ 2010, Table 2 ) and artifact gold ( Petersen 1970/2010, Table 18 ) were, respectively, high (0.1-1%) in mercury and low (<0.01%) in mercury. This is consistent with amalgamation and lowering the initial high mercury content of the alluvial gold by the refogado process.
· ICP (Inductively Coupled Plasma) analyses of gold composition showed that similarly low levels of mercury (<20 ppm Hg) in pre-contact gold artifacts and modern refogado gold, where mercury is used, are consistent with amalgamation of alluvial gold in the past ( Brooks et al., 2013 ).
With the exception of mercury found in an ancient Maya tomb ( Pendergast, 1982 ), native mercury has rarely been reported in the archaeological record. However, this does not mean that it wasn’t geologically available ( Roberts & Irving, 1957 ) and used. Given, that the use of mercury in gold processing is dissipative, then the tons of gold produced in pre-contact Peru is the hard evidence for amalgamation and is consistent with the above data. Peru produced 16-22 tons of gold annually from small-scale alluvial gold mines during 2007-2011, mainly in Madre de Dios, using mercury amalgamation ( Gurmendi, 2012 ). And today, Peru produces ~1.5 tons of gold per month from small-scale alluvial mines that use the ages-old technique of gravity separation and mercury amalgamation ( Cánepa, 2005 ; Brooks et al., 2007 ; Ahern, 2016 ; Chauvin, 2018 ; Soto-Viruet, 2018 ). From ancient-to-modern time in Peru and elsewhere, the primary use of mercury has been for small-scale alluvial gold mining.
4. Mercury and Human Health
In the present-day small-scale gold mining areas in Colombia, Peru, and other countries where mercury is used to amalgamate gold, the dissipative refogado process releases toxic mercury vapor that severely affects the brain, nervous system, kidneys, and other organs ( CDC, n.d. ). Gold workers in Antioquia, Colombia who are exposed to mercury vapors have classic mercury poisoning symptoms that include depression, kidney problems, and trembling ( Webster, 2012 ).
Knowledge of the effects of mercury toxicity; however, is not new. Cinnabar is toxic ( Sax, 1984 ; Brown, 2001 ) and was used as a preservative, for example, in ancient Hellenistic burials ( Maravelaki-Kalaitzaki & Kallithrakis-Kontos, 2003 ) and at the ~7000 BC archaeological site of Çatalhöyük, near the mercury district of Konya, Türkiye ( Barnes & Bailey, 1972 ). Cinnabar and hematite pigment use dates to 5300 BC in Spain ( Domingo et al., 2012 ). Cinnabar workers in the ancient world were advised to wear a mask to prevent inhalation of the dust (Pliny the Elder, 55 AD) which was a very serious health hazard. Agricola (1556/1912) warned retort workers to avoid breathing mercury fumes that were known to cause loose teeth and Barba (1640/1923) warned that “mercury would pass through flesh and the hardest bone.” Mercury was available in colonial Peru and was used for silver amalgamation. In Lima, it was also sold as a cure for syphilis; however, it caused salivation, dehydration, and eventually destroyed the jawbone without actually curing the disease ( De Peralta, 2018 ).
In pre-contact Peru cinnabar mining and use of mercury for amalgamation may be inferred from similar health warnings regarding toxic cinnabar dust and mercury vapors. The nervous system, in particular, was affected and resulted in “…el temblar y perder los sentidos”, […shaking and loss of the senses] therefore, cinnabar mining was prohibited by the Inca and its uses were forgotten only to be revived in 1567 by the Europeans ( Larco Hoyle, 1945/2001 ). Exposure to toxic cinnabar dust and mercury vapors would have occurred during: 1) firesetting and cinnabar mining, 2) retorting cinnabar to obtain mercury, and 3) during the refogado process to produce gold. The Inca health warnings therefore indicate that cinnabar was mined, retorted, and mercury was used before the arrival of the Europeans.
5. Map Compilation
Mercury occurrences are known widely in South America and Mexico: Bolivia ( Barba, 1640/1923 ; Ahlfeld & Schneider-Scherbina, 1964 ), Chile ( McAllister et al., 1950 ), Colombia ( Wilson, 1941 ; Lozano, 1987 ; Brooks, 2014 ), Ecuador (T ruhan et al., 2005 ), and Peru ( Arana, 1901 ; Garbín, 1904 ; Yates et al., 1955 ; Petersen, 1970/2010 ; Giles, n.d. ). The most well-known occurrences include Huancavelica and Chonta, Peru ( Arana, 1901 ; Garbín, 1904 ; Cobbing et al., 1996 ); Aranzazu (Nueva Esperanza) and El Cinabrio, Colombia ( Singewald, 1950 ; Buitrago & Buenaventura, 1975 ; Brooks, 2014 ); and Azogues, Ecuador ( Brooks, 2018 ). Mercury occurrences are also known in Queretaro, Mexico ( Langenscheidt, 1986 ; Consejo de Recursos Minerales, 1992 ) and in Central America ( Roberts & Irving, 1957 ). Herein, the term “occurrence” is used to indicate any geochemical anomaly of cinnabar and includes mines as well. The crustal average for mercury in igneous rocks is 0.08 ppm and for sedimentary rocks it is 0.03 - 0.4 ppm ( Turekian & Wedepohl, 1961 ).
Over 3000 flasks of mercury were produced annually throughout the 1960s at Huancavelica, the most well-known cinnabar-mercury occurrence in Peru ( Arana, 1901 ; Whitaker, 1941 ; Yates et al., 1955 ; Roskill, 1990 ; Brown, 2001 ). And, in the 1840s mercury production also took place at Chonta ( Garbín, 1904 ; Deustua, 2010 ). Therefore, a map showing these and other occurrences is basic to establishing the availability of this mineral resource in Peru, whether used for pigments, as a source of cinnabar for retorting mercury, or later colonial silver amalgamation. A map is also important for evaluation of point sources of mercury for present-day environmental studies.
Peruvian mineral resource maps are available for gold, copper, silver, and lead-zinc occurrences, but not cinnabar-mercury ( Atlas, 1999 ); however, approximately 20 cinnabar-mercury occurrences are listed by Petersen (1970/2010) . Many of these occurrences might be called districts because they include numerous mines and workings, such as the 10 mines at Chonta ( Garbín, 1904 ). And, in most cases, since only geographic names were given, and not latitude and longitude, the locations are approximate. Locations were compiled (Figure 1) along with data from additional reports, some unpublished, and maps ( Vercelli et al., 1977 ; Cobbing et al., 1996 ) from the Instituto Geológico Minero y Metalúrgico (INGEMMET), Lima.
Some of the cinnabar occurrences may have initially been ancient mines for pigments, native mercury, or other metals and have now been obscured or overprinted by modern mining. There is no present-day mercury production in Peru from the occurrences shown on Figure 1. However, mercury is produced as a byproduct from treatment of porphyry copper-gold ores with cyanide from mines such as Pierina and Yanacocha. However, because of global environmental and human health considerations, Peru’s byproduct mercury is exported for treatment and retirement ( Brooks et al., 2007 ; Ahern, 2016 ).
Buldibuyo/Pelagatos/Pampas (2) site visit— Cabrera la Rosa (1954) indicated a cinnabar occurrence near Buldibuyo. Examination of the geologic report for the area did not list a mercury occurrence ( Balarezo, n.d. ); however, the report by Ravines (1978) did include “Buldibuyo” as being near Pelagatos in central Peru, and near the village of Pampas. This occurrence is important given the specific geographic reference to “Buldibuyo” that was provided by both Cabrera la Rosa (1954) and Ravines (1978) . Additionally, it is near Pataz where pre-Inca and Inca alluvial gold mining has been documented ( Zarate, 2006 ). On Figure 1 this occurrence is indicated as Buldibuyo/Pelagatos/Pampas.
After leaving Pampas along a road paralleling Lago Pelagatos, samples with cinnabar were taken at a northeast-trending fault with abundant rusty water and pyrite. An outcrop with cinnabar was found along this fault that extended for several kilometers (Figure 2). The fault was iron-stained and rusty water drained from the fault. Pyrite was also found along the fault and decomposition of the pyrite is the likely source of the rust-stained water. Two samples contained 24-82 ppm Hg (Table 1) and are above the crustal average of 0.08 ppm Hg ( Turekian & Wedepohl, 1961 ). Tungsten and other large-scale mining in the area limited
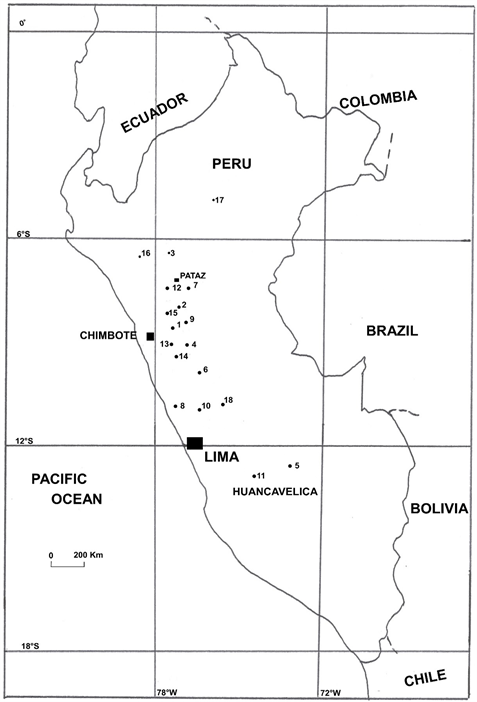
1—Baños de Jesus (BañosTermales de Monterrey?), 9˚32'S/77˚32'W ( Ravines, 1978 ); 2—Buldibuyo/Pelagatos/Pampas, 8˚07'S/ 77˚23'W ( Cabrera La Rosa, 1954 ; Ravines, 1978 ); 3—Chachapoyas (Sonche?), 6˚13´S/77˚52´W ( Bolétin, 1900 ; Petersen, 1970/2010 ; Ravines, 1978 ; Deustua, 2010 ). “Pinturasrupestre de Pollurua…color rojoocre” [Pollura rock art....color of red ochre] may indicate cinnabar as pigment; 4—Chonta/Huallaca/Queropalca, 12˚38'S/74˚26'W ( Petersen, 1970/2010 ; more than 15 named mines are noted at Queropalca by Garbín, 1904; Giles, n.d. ); 5—Chuschi, 13˚35'S/74˚21'W ( Petersen, 1970/2010 ); 6—Cuipan/Cuypan/Quipán, 10˚34'S/76˚29'W (cinnabar was taken from “bocaminas y socavonesantiguos” [mine openings and ancient adits] at Cuipan which is ~30 - 37 km northwest from Cerro de Pasco towards Yanahuanca, Garbín, 1904 ; Giles, n.d. ; Petersen, 1970/2010 ; INGEMMET, 1999 ); 7—Huacrachuco, 8˚30'S/77˚04'W ( Petersen, 1970/2010 ); 8—Huara, 11˚06'S/77˚36'W ( Giles, n.d. ); 9—Huaraz, 9˚31'S/ 77˚31'W ( Petersen, 1970/2010 ); 10—Huarochiri, 11˚50'S/76˚22'W ( Petersen 1970/2010 ); 11—Huancavelica/Villa Rica de Oropesa/Santa Barbara, 12˚47'S/74˚54'W ( Arana, 1901 ; Yates et al., 1955 ; Petersen, 1970/2010 ; Brown, 2001 ; locations and descriptions of other mines and prospects in the region that include: Camarada, Excelsior, Carniceria, Azulcocha, ChaqaOreco/Ventanilla 7, Huajoto, Torres Orgo, San Antonio, and Pequeña are given in INGEMMET, 1999 ); 12—Paccha, 7˚59'S/77˚42'W ( Petersen, 1970/2010 ); 13—Pampas, 9˚40'S/77˚49'W ( Petersen, 1970/2010 ); 14—Punabamba, 9˚31'S/77˚31'W ( Petersen, 1970/2010 ); 15—Santa, 9˚04'S/78˚35'W ( Petersen, 1970/2010 ); 16—Santa Apolonia, 7˚09'S/78˚31'W ( Petersen, 1970/2010 ); 17—Santa Cruz, 5˚33'S/75˚48'W ( Petersen, 1970/2010 ); 18—Yauli, 11˚40'S/76˚05'W ( Petersen, 1970/2010 ); The following occurrences are not shown on the map: 19—Cerro Azoguines, 15˚45'S/70˚01'W ( Petersen, 1970/2010 ; INGEMMET, 1999 ; Diggings, n.d. ); 20—Carachugo/Cajamarca, 7˚09'S/78˚30'W ( INGEMMET, 1999 ); 21—Cangallo/Minascucho/Chauschi, 13˚37'S/74˚08'W ( INGEMMET, 1999 )
Figure 1. Approximate locations of cinnabar-mercury occurrences in Peru.
![]()
Figure 2. Samples of cinnabar were taken at northeast-trending fault along road that parallels Lago Pelagatos. The rust-stained water is from the decomposition of pyrite associated with cinnabar.
![]()
Table 1. ICP data for Pelagatos and Chonta cinnabar-mercury occurrences, Peru.
Multi-element ICP analyses in parts per million (detection limit given to right of element, in parentheses, Au-fire-assay); American Assay, Sparks, NV [ICP-2A024-Pelagatos SP0124038; Chonta SP0130401]. Sample Descriptions: PE181 [0192625/9095652 UTM] Pelagatos, dark fg quartzite, spot sample along road parallel to lake, cinnabar exposed along N 30˚ E fault in roadcut, abundant pyrite and Fe-stained water. PE182 [0192625/9095652 UTM] Pelagatos, dark fg quartzite, area sample along road parallel to lake, cinnabar exposed along N 30˚ fault in roadcut, abundant pyrite and Fe-stained water. PE191 [298130/8883304 UTM] Chonta, altered, quartzite, clay, along road at first main adit, area sample of 20 m wide breccia zone, altered with Fe stain. PE192 [298130/8883304 UTM] Chonta, at adit, area sample, vuggy, Fe stain. PE193 [298130/8883304 UTM] Chonta, at adit, float sample, dark, with pyrite. PE194 [738108/4293829 UTM] Chonta, quartzite, mine waste, with cinnabar on surface. PE196 [738108/ 4293829 UTM] Chonta, roadside, spot sample with cinnabar and pyrite. PE197 [738108/4293829 UTM] Chonta, at mine near plant, spot sample with abundant pyrite.
further access and likely eliminated or overprinted any traces of ancient mines.
Chonta/Huallaca/Queropalca (4) site visits—Chonta and Queropalca (Garbín, 1904; Giles, n.d.) may be accessed by a well-marked dirt road from Huallanca, to La Unión, and Baños or from Huánuco. Chonta was supposedly “discovered” upon orders from Spain in 1756 to find new sources of mercury to be used for colonial silver amalgamation. However, it is very likely that occurrences such as Chonta or Palcas, which is near Huancavelica, were first worked by pre-Inca people as a source of vermilion or vermellón pigment ( Arana, 1901 ) as well as native mercury—similarly, the early use of cinnabar as a red pigment by the Ohlone people in California led to the “discovery” of the New Almaden mercury mines in California by Spanish explorers ( Lanyon & Bulmore, 1967 ; Boulland & Boudreault, 2006 ).
In the 1840s, there were over 2000 miners and more than 20 individual mines at Chonta (Figure 3). There were 11 retorts and fuels included locally available coal, peat, and a grass called ichu. Water for condensers came from a nearby lagoon, Chonta Cocha. The mines produced 8-10 flasks (one flask contains ~76 pounds of mercury) of mercury per day. The grass roofs (ichu) of the buildings collected droplets of mercury lost during retorting ( Garbín, 1904 ) and some of the buildings still remain (Figure 4).
At Chonta “trabajos antiguos” [ancient workings] likely indicates pre-contact cinnabar mining; however, it is unclear as to the exact location or how old these workings might be. Similarly, “…bocaminas y socavones antiguos” […mine
![]()
Figure 3. One of several adits at Chonta, note high-angle structure at entry.
![]()
Figure 4. Buildings and mine tailings at Chonta, smelter stack in middle-distance with ground chimney and stack to right.
opening and adits] likely indicate pre-contact mining at Cuipan (Cuypan, Quipan) ~30 km from Cerro de Pasco towards Yanahuanca ( Garbín, 1904 ; Giles, n.d. ). However, samples from a small exploration pit, and surely not the mine referenced by Garbín (1904) , indicated only low gold and no mercury values (Figure 5, Table 2). Chonta closed in 1843; however, not because the ore was exhausted, but because of opportunities for miners willing to immigrate and
![]()
Figure 5. Exploration pit at Cuipan, contact between limestone (left) and dacite (right).
![]()
Table 2. ICP data for Cuipan exploration pit, Peru.
Multi-element ICP analyses in parts per million (detection limit given to right of element, in parentheses); American Assay, Sparks, NV [ICP-2A024-SP0143538]. Sample Note: B220-223 [10˚34.162 S/76˚29.373 W] Cuipan, area sample at 4 m × 2 m shallow pit, Fe-stained, west strike, limestone-dacite contact, >14300 ft.
work at the mercury mines at New Almaden, California ( Garbín, 1904 ; Giles, n.d. ). New Almaden mercury would be needed for the 1849 California Gold Rush.
Samples from Chonta for this reconnaissance contained > 1000 ppm Hg; 10 - 199 ppm Ag; 84 - 6391 ppm As; 21 - 367 ppm Sb, and 31 - 9503 ppm Zn. Gold values are 0.01 - 0.07 ppm, but may increase with depth (Table 1). These elements are all above background and indicate further study focused on silver and gold in association with mercury ( Turekian & Wedepohl, 1961 ; Noble & Vidal, 1990 ). Specifically, the high arsenic is a pathfinder for gold and the high mercury content is an indicator of Pb-Zn-Ag ore ( Rose et al., 1979 ). Chonta is an altered plagioclase-quartz dacite stock that intrudes sedimentary rocks of the Oyon and Casalpaca Formations ( Cobbing et al., 1996 ). Minerals are hosted in quartzite and sedimentary rocks and include pyrite, sphalerite, galena, cinnabar, native mercury, and silver in mainly NS structures.
Queropalca is a polymetallic Au-Ag occurrence hosted in quartzites of the Chimu Formation that was supposedly “discovered” in 1736; however, there are several adits that indicate pre-contact mining. Evidence includes: dipping floors; with only daylight for work the adits were shallow; and the adits were spaced along the vein (Figure 6(a)). Mineralization is hosted in veins that are several meters wide and contain gold, pyrite, chalcopyrite, and copper. There were 15 named mines at Queropalca; however, production ended by 1894 ( Garbín, 1904 ). It is important to indicate that mercury was used for amalgamation at Queropalca, likely from the mines at nearby Chonta, to treat the minerals. Exploration drilling showed several meters of gold-silver mineralization with values as high as 10 ppm Au, 1500 ppm Ag, and 0.35% Pb ( Candente Gold Corp., 2009 ). A hot-spring was mapped in the area and this suggests a hot-spring Au-Ag exploration model for the district, especially given the high antimony and arsenic at Chonta ( Berger, 1986 ; Candente Gold Corp., 2009 ). Surface samples from Queropalca for this study indicated as much as 6.4 ppm Au; >100 ppm Ag, 700 ppm Cu, >1600 ppm As, and 31 ppm Hg (Figure 6(b), Table 3).
Mercury was also found at Huallanca but was not produced because of its
(a)
(b)
Figure 6. (a) Multiple adits at Queropalca, along steeply dipping vein in quartzite; (b) Queropalca sample B2210 with 6.44 ppm Au, >100 ppm Ag, and 31.8 ppm Hg (Table 3). Brassy chalcopyrite on left and white hot-spring travertine on right.
low-grade and limited extent ( Arana, 1901 ). There are several mines at present-day Huallanca, for example Minera Santa Luisa, where mining dates to 1721 and gold, silver, and copper were produced from three veins. Pyrite, chalcopyrite, sphalerite, and copper minerals are reported from well-named mines such as Komstock and Eureka ( Garbín, 1904 ).
Cerro Azoguines site visit—This occurrence, also known as the Cerro Azoguines quicksilver mine, is a small adit east of Alto Puno, in southern Peru. It was found in ~1640 and produced mercury, cinnabar, and tetrahedrite; however, now it is inactive ( Petersen, 1970/2010 ; INGEMMET, 1999 ; Diggings, n.d. ).
![]()
Table 3. ICP data for Queropalca gold-silver occurrence, Peru.
Multi-element ICP analyses in parts per million (detection limit given to right of element, in parentheses); American Assay, Sparks, NV [ICP-2A024-SP0143538]. Sample Note: B224-2212 [10˚10.115 S/76˚48.496 W] Queropalca area samples, veins with pyrite, chalcopyrite in quartzite, >12,500 ft.
The adit is small, bifurcated, and extends for only a few meters (Figure 7) into Miocene volcaniclastic rocks ( Rodriguez Mejía et al., 2020 ). Samples indicate 3 - 45 ppm Hg, up to 400 ppm As, and minor Sb (Table 4).
Apu Campana (Fe pigment) site visit—Apu Campana, near Trujillo, is a pre-contact adit that was initially considered as a source for cinnabar and other minerals used by the Moche ( Franco Jordán, 2012 ; Peruvian Times, 2012 ). Local inhabitants had identified the red mineral as mercury; however, analyses by
![]()
Figure 7. Adit at Cerro Azoguines quicksilver mine, Puno.
![]()
Table 4. ICP data for Cerro Azoguines cinnabar occurrence, Peru.
Multi-element ICP analyses in parts per million (detection limit given to right of element, in parentheses). American Assay, Sparks, NV [ICP-2A024-Cerro Azoguines SP0137487]. Sample Note: AZ1-8 [15˚45'S/78˚30'W] alt. volcanic and volcaniclastic rocks.
Prieto et al. (2016) showed no cinnabar but rather the iron mineral hematite which was also used as an ancient red pigment ( Petersen, 1970/2010 ). Hematite mining for pigment use on pottery dates to 2000 years ago in Peru ( Vaughan et al., 2007 ). Geochemical data from samples taken during a reconnaissance of the Apu Campana adit for this study similarly show no mercury (<0.5 ppm Hg; Table 5).
6. Retorting Mercury from Cinnabar
Some native mercury may have been obtained from the cinnabar outcrop; however, retorting mercury is a straightforward process that requires cinnabar ore, retorts, fuel, water, and a condenser to trap and cool the volatilized mercury vapors. The oldest mercury retort dates to 8000 years ago in the ancient Konya mercury district in Türkiye and it consisted of a large block of marble upon which the ore was placed along with charcoal fuel. A large clay bowl cooled and condensed the mercury vapors while allowing the sulfur vapor to escape through a chimney made of ceramic tubes ( Barnes & Bailey, 1972 ). Mercury was used to exploit the placer gold deposits in the region ~7000 years ago ( Healy, 1979 ).
Other retorts include rows of double ceramic pots as shown in Agricola (1556/1912, Book IX, p. 427) and a pre-contact double-ceramic mercury retort from Sierra Gorda, Queretaro, Mexico ( Langenscheidt, 1986 ) where there are many ancient cinnabar mines and retorting mercury dates to the 10th century BC ( Consejo de Recursos Minerales, 1992 ). Descriptions and sketches of a variety of mercury retorts from China, Germany, and Mexico were compiled by Craddock (1995) . Ancient retorts were known at Huancavelica ( Rivero & Tschudi, 1853) and at Chonta, a chimney and buildings that housed retorts and condensers in the 1840s still remain (Figure 4).
At New Almaden, California the retorts consisted of whaling oil-try pots that were inverted over the cinnabar ore, sealed, and then fired with wood. The cinnabar inside the metal pot was heated, the mercury volatilized, the vapors cooled and condensed, resulting in mercury ( Boulland & Boudreault, 2006 ). In Indonesia, up to a ton of mercury can be produced daily, using locally available cinnabar, from a simple, backyard wood-fired retort. This mercury is then sold directly to local small-scale gold miners or exported ( Paddock, 2019 ).
Droplets of native mercury could be obtained directly from the outcrop or
![]()
Table 5. ICP data for Apu Campana/Portachuelo Fe pigment occurrence, Peru.
Multi-element ICP analyses in parts per million (detection limit given to right of element, in parentheses); American Assay, Sparks, NV [ICP-2A024-Apu Campana SP0137017]. Sample Note: AC1-9 [7˚56'26"S/79˚07'59"W] ApuCampana/Portachuelo, coarse-grained, Fe-stained alt. granodiorite.
from hammering the ore and, though seldom used, cinnabar could be rubbed with vinegar in a copper mortar to obtain mercury ( Takacs, 2000 ). However, only retorting would have provided the amounts of mercury needed to produce the tons of alluvial gold produced before the arrival of the Europeans.
Therefore, given the geological evidence for the regional availability of cinnabar-mercury occurrences and the widespread use of mercury for gold amalgamation in the past that continues to the present, it remains only to show how cinnabar could easily be retorted to provide mercury using materials readily available in the ancient world. A simple retort was modeled from the racks of double-ceramic retorts shown in Agricola (1556/1912, Book IX, p. 427) and a pre-contact double-ceramic mercury retort from Sierra Gorda, Queretaro, Mexico ( Langenscheidt, 1986 ). This rudimentary process does not produce vermilion, only a sooty mercury-rich residue, and metallic mercury (Figures 8-11) that would have been collected and then used for ancient small-scale alluvial gold mining.
![]()
Figure 9. Double-ceramic retort with clay seal, vent to right.
![]()
Figure 10. Retort in place with charcoal fuel (~600˚ F).
![]()
Figure 11. Mercury droplets in black mercury-rich residue along rim of ceramic retort. The blackened interior of a mercury smelter chimney at Karaburun, Türkiye had >400 ppm Hg ( Brooks et al., 2017 ). At the retorts at New Almaden this black sooty residuewas scraped and removed to obtain additional mercury ( Boulland & Boudreault, 2006 ).
7. Conclusion
Present day mining technology provides insight into pre-contact alluvial gold production in ancient Peru. References and data herein are also consistent with amalgamation in pre-contact Peru. Given that the use of cyanide dates only to the 1880s, the volume of gold produced in pre-contact Peru indicates ages-old amalgamation as key to past gold production. Availability of mercury is indicated by compilation of cinnabar-mercury occurrences and is important to the study of mineral resources and their uses in the ancient Andes. Huancavelica remains as the most well-known mercury occurrence, now followed by Chonta.
The availability of cinnabar-mercury is applicable to the study of mineral resource uses, as a source of red pigment (vermilion) and as a source of ore for mercury in pre-contact Peru. Of these, the use of mercury for gold amalgamation in the present and past helps to explain the indigenous technology that resulted in the incredible gold production that took place in ancient Peru before the arrival of the Europeans. In addition to ancient cinnabar mining at Huancavelica, there are references and evidence for ancient mine workings at Chonta, Cuipan, and Queropalca as well as pre-Inca gold mining at Pataz.
Some native mercury was available, for example at Huancavelica or Chonta; however, retorting cinnabar was a simple process that dates to ancient times worldwide. In Peru, retorting would have utilized readily available materials such as cinnabar, clay for the ceramic retorts, water for cooling, and fuels such as charcoal, coal, grass, or wood. Much as mercury is used today in Peru’s small-scale alluvial gold mines in Madre de Dios or Marañon, in the past, mercury would have been sourced from the cinnabar occurrences in Peru, retorted, and used for pre-contact gold production.
Acknowledgements
Sincere thanks are expressed to Rolando Moreno, director of the INGEMMET library, in Lima for providing maps and geologic reports on mercury in Peru and to Sr. Christian Ormeño for security and transport in the field.