1. Introduction
In 1979, the Academic Institute for Applied Research (MIGAL) was established in northern Israel to promote peripheral areas in various fields, including agriculture, industry, higher education, etc. [1]. Over the years, the Institute has grown; the number of employees has increased in parallel with its budget. MIGAL’s contribution to the development of academia in northern Israel, as well as its overall contribution, is described in the work of [2]. MIGAL staff includes over 90 PhDs and 190 researchers in 44 research groups, and it operates as an innovative research ecosystem that encourages collaboration across scientific, industrial, agricultural, academic, and technological specializations. The influence of universities and research institutes on regional development is well known (e.g. [2] [3]). Collaborations with the Institute and its researchers cover different fields of agriculture, industry, and academia, through MIGAL’s departments of Soil, Water and Environment, Molecular and Computational Biosciences and Biotechnology, Plant Sciences, Nutrition and Natural Products, and Northern R & D, which includes various research groups led and supervised by senior researchers. In this article, we will focus on MIGAL’s research related to fish and aquaculture, i.e., research groups working directly or indirectly on the biology and physiology of fish and amphibians in water and agricultural biotechnology under specific field conditions in northern Israel and other regions of the country [4] [5] [6] (Figure 1). Research and development are focused on fish with global export marketing potential alongside import prevention [5]. This paper explores the relationship between the MIGAL aquaculture research group and Precision Agriculture—Caviar Galilee regarding acclimatization of the Russian sturgeon (A. gueldenstaedtii) in northern Israel: introduction, food development [7], reproduction, hormonal systems that control reproduction and growth [8] - [15], molecular markers of gonadal development and sex determination [16] [17] [18], and an economic assessment of caviar production and its viability [9] [19] (Photo 1). The emergence of Russian sturgeon is due to their decreasing numbers in their natural habitat and the rising price of meat and caviar [12], resulting in the global development of sturgeon aquaculture [5] [20]. In 2017, there were over 2300 industrial sturgeon farms [21]. From an economic
![]()
Figure 1. Seven years of male and female Russian sturgeon growth at Caviar Galilee [5] [9].
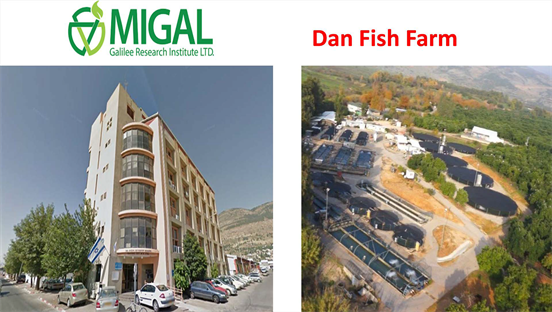
Photo 1. Galilee Research Institute (MIGAL) and Dan Fish Farm.
point of view, breeders are interested in identifying females that produce caviar of high economic value and culling males with low economic value. Endoscopic methods of sex determination can only be used after gonadal differentiation, i.e., at 3 years of age or later. The sample required for the proposed early sex determination is not taken from the gonads, but from the dorsal fin (tissue) of 6-month-old fish, using molecular methods that allow sex discrimination in the months before sex differentiation concerning based on DNA variation (this was confirmed in a study on a Dan River sturgeon farm, unpublished data) [19].
2. Food Development for the Hybrid
Food development for Russian sturgeon (Acipensergueldenstaedtii) hybrids [7], and the study of genes encoding sex hormones (kisspeptin, GnRH, FSH, LH) have been described [9] [10] [12] [15] [22] [23] [24]. The hormones controlling growth are described in [14] and insulin-like growth factor receptor 1 (IGF-1R) is described in [23] [25]. The major product of the industry is caviar, so female reproduction is an important aspect, and methods of separating males and females have been developed (Diagram 1). Under the growth conditions of Kibbutz Dan and Caviar Galilee fish farms, fish are grown at a temperature of 20˚C - 22˚C and differentiation occurs over approximately 3 years (Diagram 1). At this point, the females and males are separated [9]. Before gonadal differentiation, identification is mainly based on histological examination, as described for the genitalia [25] [26], followed by endoscopy [11] (Diagram 1). In the search for genes that differentiate males and females, methods of expressing genes in the gonads [16] [17] [19] [27] and finding genes in the genome [18] have been used.
2.1. Summary
The construction of water-conservation projects may change the transport of water, sediment and nutrients, and food web dynamics in river systems, thereby
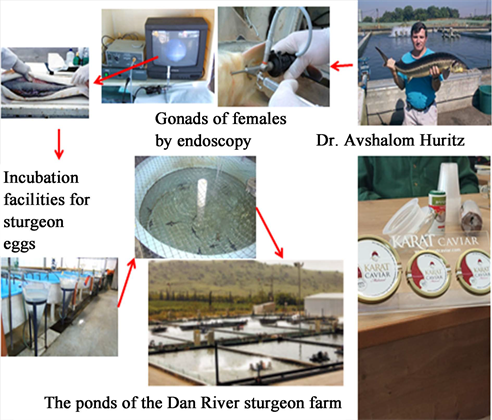
Diagram 1. Description of the various stages of Russian sturgeon reproduction and growth occurring at Dan Fish Farm and Galilee Caviar. Separation of male and female Russian juvenile sturgeons adapting the Russian Sturgeon to Growth Conditions in Northern Israel.
affecting downstream ecosystems, especially fish habitats. This study proposed an improved habitat model that considers both habitat quality and quantity and applies it to assess the impact of water-conservation projects on fish habitats. Fragmentation indices emphasizing core habitat patchiness and connectivity, based on minimum spanning trees, appear to be the most suitable indicators of fish habitat quality [13] [28] [29] [30] [31]. The Chinese sturgeon (Acipensersinensis) was used as the indicator species, and the spawning sites between Gezhouba Dam and Yichang Station were selected as the study area. The relationship between habitat quality, water temperature, and flow was established in those areas. The results showed that the recommended flow rate for spawning and hatching of Chinese sturgeon was 13,100 to 24,200 m3/s, with a water temperature of 17.1˚C to 20.0˚C. The improved habitat model showed that both the water-storage operation of the Three Gorges Dam and the construction of the separation levee project led to qualitative deterioration of the Chinese sturgeon spawning sites. Compared with previous studies, improved habitat models provide a promising intermediate step to reducing the uncertainty in assessing and quantifying the impacts of water-conservation projects on fish habitats [13] [28] [29] [30] [31].
2.2. Adapting the Hybrid Acipensergueldenstaedtii is Recommended for Aquaculture in Northern Israel
All steps for the extradition of eggs of Acipensergueldenstaedtii to the Dan River waters and their cultivation are described in the work of [5]. Adaptation of this hybrid to the growing conditions includes: bringing eggs from Russia to Israel, hatching them in Dan River water (northern Israel), handling larvae hatched from the eggs, training and fattening the fry by preparing food for different growth stages (Diagram 1) [5] [7] [9].
2.3. Incubation and Nursery Stages
The 70,000 fertilized eggs from Russia were shipped in ice-filled trays. Eggs averaged 0.056 g. Eggs were hatched in the same type of incubation jars used to cultivate trout, at a density of 10,000 eggs per container. The water temperature was 16˚C (Dan River water temperature). Eggs were kept at this temperature for 5 days before arriving in Israel, and hatched 3 - 5 days after their arrival. The 20,000 hatched larvae were then transferred to six large 2 m3 (4 m2) fiberglass tanks, and were ready to receive food about 14 days after the beginning of incubation (8 days after hatching). The mortality rate was 14% - 18% at this stage. Dry trout starter (feed no. 0, EWOS Ltd.) and Artemia nauplii [5] [9] were given to the larvae. Large fish weighing 1 kg achieved a weight of close to 5 kg during their third year of growth, making them fit for selling. Three years are required to raise a giant fish weighing 4 - 8 kg (Figure 1). Market demand and product development influenced the selection of the appropriate size and weight for cultivation [5] [9].
2.4. The Development of Gonads in Acipensergueldenstaedtii
During growth at the Dan fishery, hybrids were investigated for suitable development under the growing conditions. The gonads of three different sizes of fish (1 g, 50 g, and 150 g) were examined histologically. The females were around 13 months old. Gonadal development was positively correlated with body size. Only 28% of Acipensergueldenstaedtii gonads had differentiated into sex cells in all three fish studied. It was difficult to tell if the gonads were young and immature based on investigations [26]. The gonads were difficult to differentiate from the entire histological pictures of 4-year-old and 8-year-old sterile hybrid sturgeons based on the results.
2.5. Sturgeon Food
Sturgeon need food that is high in protein. The idea is to adapt food available in the Israeli market—trout, carp, and tilapia—to fish in aquaculture. The diets were trout (40% - 45% protein), carp (30% - 35% protein), and tilapia (approximately 25% protein). The sturgeon grew best with the trout diet [5] [7]. Food composition and feed amount are described in [7]. The food composition prepared at MIGAL was 50% basic diet and 50% soy meal, fish meal, or poultry. The protein and energy digestibility coefficients and the ratio of feed digestibility to consumption were higher for soybean and fish meal than for poultry meal, but lower than for other carnivorous fish [5] [7]. The chemical analysis of fish (trout, carp, and tilapia) food products on the market were modified, indicating the importance of these ingredients, in addition to the very important protein. Acipensergueldenstaedtii mastered energy (5132 - 5178 kg/g), lipid (3.9% - 12.9%) and fiber (7.42 - 10.3). Further experimentation is required to determine the optimal protein percentage and source (fish meal, soybean meal, or meat meal). Another important parameter is the optimal feed ration relative to body weight [5]. In the second experiment, three sturgeon diets were studied: low protein (35%), trout (40%), and high protein (45%). The importance of lipids and fatty acids for oocyte development and gonadal maturation could not be ascertained for Acipensergueldenstaedtii. The effect of fatty acids on the juvenile Russian sturgeon was studied [32].
3. Oogenesis and Spermatogenesis under Aquaculture Conditions
Description of the Various Stages of Oocytes in the Ovary of Russian Sturgeon (Acipensergueldenstaedtii) in the Dan Fish Farm and Caviar Galilee under Constant Conditions at 19˚C - 22˚C
The described gonadal development primarily involves development of the ovary and oocyte, with less emphasis placed on testis development [10] [11] [26]. During the first 3 years of growth (Figure 1), the gonads undergo ovarian and testicular differentiation under conditions of 19˚C - 22˚C (Figure 2), as shown by histological studies of the gonads (Figure 3). However, the exact timing cannot be determined from these studies [10] [11] [26].
Ovarian development and egg production have been better studied year-round under these relatively constant conditions than male gonadal development. Development of the egg at different stages (oogenesis) until the oocyte reaches the stage of vitellogenesis (black caviar) has been widely described [11] [12]. In a 7-year-old female’s ovaries, eggs appear at different stages of development. The presence of oocytes in the ovaries, confirmed by endoscopy and histologically (Figure 3), exhibited five stages of oocyte development: previtellogenic; white oocytes; yellow oocytes; gray oocytes; E. black oocytes [11]. A total of 180 eggs were of various sizes (200 - 600 µm) with wide oviparous lamellae, narrow surrounding fat pads, oil droplets, and cortical alveolar stages (Figure 3(A)). Yolk-deposited oocytes containing yolk globules larger than 600 µm were distributed throughout the cytoplasm (Figure 3(B) & Figure 3(C)). Egg yolk platelets were distributed everywhere (Figure 3(D)). Gray-stage oocytes with a diameter of 1600 - 2600 μm were in the late vitellogenesis stage, with yolk platelets throughout, and the nucleus was in the center (Figure 3(E)). The vitellogenesis black oocyte stage contains fully developed oocytes with the nucleus in a central position or migrating toward the animal poles. Stages of gonadal development (oogenesis and spermatogenesis) are shown in Table 1 [11]. These results suggest that sex differentiation for these conditions (fish growth on Galilean caviar farms) occurs during the first year of growth. Previtellogenesis occurs at 1 - 4 years of age, and the vitellogenesis phase begins at 6 years of age. In males, the process of spermatogenesis begins in the second year, and after 3 years, males reach sexual maturity (Table 1).
![]()
Figure 2. Stages of female and male gonadal development in Russian sturgeon. Histology and hematoxylin & eosin staining were performed as described in [26]. (A) Histological section of the juvenile sturgeon ovary, typical for a 3-year-old fish; scale bar = 100 lµM. (B) Typical ovarian section for a 4-year-old fish; scale bar = 100 lµM. Labels: GN, oogonia; AC, adipocytes; OC, oocyte; NL, nucleoli; GV, germinal vesicle; and CA, cortical alveoli. (C) Histological section of a typical juvenile (1-year-old) sturgeon testicle; stage A, scale bar = 100 lµM. (D) A typical sturgeon juvenile (3-year-old) testis tissue section; initiation of meiosis; stage B, scale bar = 100 lµ M. (E). Histological section of a typical juvenile (4-year-old) sturgeon testis; stage C, scale bar = 100 lµM. Labels: AC, adipocyte; GN, spermatogonia; CS, cyst; and SC [9].
![]()
![]()
Figure 3. Using an endoscope to examine the various stages of an oocyte in the Russian sturgeon ovary. Scale bar = 1 mm. Oocyte growth process at various stages during the development of Russian sturgeon eggs. A. Previtellogenic oocyte (pvo). B. White oocyte stage (wo). C. Magnification of the white oocyte envelope layers. D. Yellow oocyte stage. (yo) E. Gray oocyte (go). F. Black oocyte (bo). ad: adipose tissue, zri: internal radiating zone, zre: external radiating zone, gr: granulosa cells, tl: theca layer, bl: basal lamina, yg: yolk globule, yp: yolk platelet, n: nucleus, nu: nucleolus, ve: vitelline envelope, gc: gelatinous coat [11].
![]()
Table 1. Body weight and gonadal stage, 1 - 6 years.
4. Involvement of Somatotropins and Gonadotropins in the Brain-Pituitary-Gonadal (BPG) Axis that Controls Oogenesis
4.1. Hormones Involved in Gonadotropic Axes
Oogenesis in Russian sturgeon is regulated by the anterior pituitary in the BPG axis: the Kiss1 gene belongs to the RF-amide peptide family of kisspeptins, which act primarily in the hypothalamus [22] to stimulate GnRH neurons’ follicle-stimulating hormone (FSH) [9] [12] [15] [24] and luteinizing hormone (LH) [10] [15] [24], prolactin, growth hormone (GH) and IGF I [14] [24], adrenocor-ticotropic hormone (ACTH) and thyroid-stimulating hormone (TSH), similar to other fish [24]. Based on research on the Russian sturgeon, a model for somatotropin and gonadotropin control is proposed here (Diagram 2).
4.2. Russian Sturgeon (Acipensergueldenstaedtii) Kisspeptin and Gonadotropin Receptor Transcription during Oogenesis
Like other vertebrates, teleost reproduction is tightly regulated, primarily through the BPG axis. In the brain, kisspeptin has recently received considerable attention as a potential key factor in the indirect control of reproductive neuroendocrine [33]. Its effects on the hypothalamic decapeptide gonadotropin-releasing hormones GnRH 1 and 3 control the release of pituitary gonadotropins FSH and LH, and therefore control gametogenesis [34]. The Kiss1 gene, originally identified as a mammalian metastasis suppressor, produces several peptides called kisspeptins in mammals. Kisspeptin-54 and its endogenous variants Kisspeptin-14, Kisspeptin-13, and Kisspeptin-10 are produced by proteolytic cleavage of the Kisspeptin precursor from the Kiss1 gene. They share a common core sequence of 10 amino acids (Kisspeptin-10) at their C terminus, which enables them to bind to their cognate G-protein-coupled receptors (GPR54 or Kiss1r) [35] [36]. Therefore, Kiss1 controls the BPG axis, acts on the caudal hypothalamus, and appears to affect GnRH receptors [37]. In Perciformes, there are only three GnRH paralogous groups, namely GnRH1, GnRH2, and GnRH3 (previously named snapper GnRH (sbGnRH), chicken GnRH II (cGnRHII), and salmon GnRH (sGnRH), respectively) [38] and GnRH receptors bind to the pituitary and affect the secretion
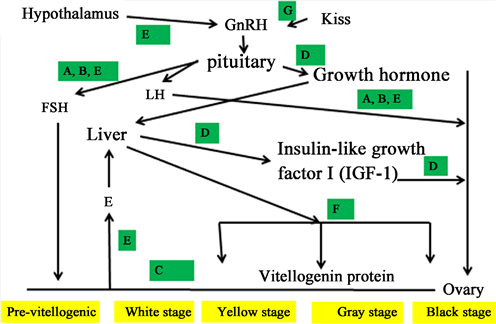
Diagram 2. Proposed model for regulation of somatotropin- and gonadotropin-producing cells involved in oogenesis of growing Russian sturgeon in Dan fish Farm and Caviar Galilee. A: [8] [10], B: [26], C: [11] [12], D: [14] [25], F: [25], G: [22].
of LH and FSH. Also, TNg-GnRH neurons possess two of the three types of GnRH receptors, suggesting that GnRH3 may have an autocrine/paracrine mechanism of action [39]. It regulates the secretion of pituitary gonadotropins, such as FSH and LH, which regulate gametogenesis [34] through steroids [40] [41].
Because of the relatively wide systematic class and significant variability of hormones involved in reproduction, investigations on kisspeptins in teleosts have revealed some diversity in their function in reproduction; further extensive studies are needed. The brain of the zebrafish (Daniorerio), one of the most extensively studied model fish, contains Kiss1 and Kiss2, as well as two kiss receptors (GPR54), Kiss1r and Kiss2r [36] [42], which are similar to those of other fish such as the lamprey (Petromyzonmarinus) [42] , medaka (Oryziaslatipes) [43], goldfish (Carassiusauratus) [44], sea bass (Moronesaxatilis) [45] and blue gourami (Trichogastertrichopterus) [46].
Multiple sequence alignment of the amino acid sequences of Russian sturgeon GPR54 with fish and mammalian GPR54 showed that the Russian sturgeon sequence was 74% identical to that of platypus GPR54, 66% identical to the European eel, and only 53% - 58% identical to fish GPR54. Sequence comparisons were performed using the Clustal W method (Figure 4).
Autocrine and paracrine production of GnRH, FSH, and LH from the gonads has received little attention. In human and fish ovaries, GnRH acts as an autocrine/paracrine regulator. The marine teleost gilthead seabream is an interesting model because gonadal tissues grow first as testes, then regress to allow the formation of ovarian follicles over the fish’s lifetime [47]. In vertebrates, ovarian GnRH appears to have an autocrine/paracrine regulatory role in modulating ovarian function (fish and mammals). The gilthead seabream ovary has a GnRH gonadotropin axis, according to Wong and Zohar [48], and FSH and LH,
![]()
Figure 4. Relative expression levels of GPR54 (A), FSH R (B), and LHR (C) in the ovary during oogenesis and growth in Dan Fish Farm. P—previtellogenic, W—white, Y—yellow, G—gray, and B—black oocytes. Each bar represents the mean (+SEM) of six oocytes completed in triplicate at each stage during the real-time quantitative RT-PCR assay. Data were normalized to RPL22. Statistically significant differences in normalized GULO data between groups were analyzed using one-way ANOVA and Tukey’s multiple comparison test. There are significant differences in the bars of different letters. p < 0.05 indicates a significant difference [22] [24].
well-known pituitary hormones, may play critical new roles in teleost intraovarian communication between the oocytes and ovarian follicle cells. GnRH1 mRNA levels were more significant in oocytes at the previtellogenic stage in blue gourami females (Trichogastertrichopterus) than at maturation of the vitellogenic and follicular oocyte stages [49]. There were no significant changes in GnRH3 mRNA levels among oocytes at various stages of development. βLH and βFSH mRNA levels in oocytes in the vitellogenic stage were lower than those in the previtellogenic stage [49]. Kiss1 and Kiss1r gene expression in the ovary has been shown in fish [50] [51]. Cloning and transcription of Kiss receptors during oogenesis have been documented [22] [24], and a model incorporating the kisspeptin hormone and oogenesis in Russian sturgeon has been developed (Diagram 2).
Kisspeptin and Gonadotropin Receptor Transcription during Oogenesis
An open reading frame was found in the nucleotide sequence of the isolated stGPR54, LH, and FSH receptors’ cDNA [24]. The 2190-bp stGPR54 gene encodes a projected 357-amino-acid peptide, as well as a 539-bp 5'UTR and 1.2-kb 3'UTR [22]. The neighbor-joining method was used to create a phylogenetic tree of vertebrate GPR54 prepropeptides (Figure 4), and the location of the Russian sturgeon in evolution was revealed (Figure 4); the amino acid sequence demonstrated that the GPR54 sequence of the Russian sturgeon is between mammal and fish.
Interestingly, when the gonad matured, Kiss1r, LH, and FSH receptor mRNA levels in the ovary rose significantly (Figure 4(A)). The greatest amounts of GPR54 were found in the black follicle stage. This suggests these genes’ importance in puberty and reproduction [22] [24].
4.3. Transcription of FSHβ, LHβ, and Glycoprotein α Subunits in Pituitary Glands of Russian Sturgeon under Stable Aquaculture Conditions
Two cDNAs were cloned from the pituitary of the Russian sturgeon, encoding the β and α subunits of the gonadotropins FSH and LH (stFSH β, stLH β, and stGPa) [9] [10] [15] [24]. Figure 5 shows relative mRNA levels measured by real-time quantitative PCR (qRT-PCR) for stFSH β and stLH β in males and females over 4 years of growth. The level of stFSH β mRNA was significantly higher in females than in males and changed during the first 4 years. These results support the proposed model that FSH affects the process of oogenesis, as described in qualitative models of hormone control of this process (Figure 4 and Figure 6), and that LH mRNA transcription in females occurs more modestly during 4 years of growth changes; there was no significant difference in mRNA levels between years in this growth process (Figure 5). From a comparison of FSH and LH expression, FSH controls vitellogenesis and LH at maturity, very similar to teleost fish [34] [52]. Significant increases in FSH mRNA levels were found in the third year of growth [10] (Figure 5), when the fish reached puberty [9] [10] (Figures 3-5). No significant levels of FSH mRNA were found during the first 2 years of growth, and no difference was found in LH mRNA levels during 4 years of growth. These results suggest that FSH is more involved in the control of spermatogenesis than LH. However, more research is needed to understand the differences between FSH and LH that control Russian sturgeon oogenesis. Several studies support a distinct role for FSH and LH in the control of oogenesis in white sturgeon (Acipensertransmontanus), which complements the distinct roles of these two gonadotropins and may also occur in Russian sturgeon [52] [53] [54].
![]()
Figure 5. FSH and LH β-subunit gene transcription changes during growth in northern Israel for quantitative RT-PCR. S rRNAs were obtained by the CT cycle method, where 2-ct CT reflects relative amounts of relative β-subunit transcripts. Results are the mean ± SEM (n = 4 - 9). Means marked with different letters were significantly different (p < 0.05) [10].
![]()
Figure 6. During growth in permanent aquaculture settings, the plasma E2 (I) and 11-KT (II) levels of Russian females and males were measured. At the age of 6 years, oocytes are present at the start of vitellogenesis [9] [12]. The effect of hormonal therapies on E2 plasma levels in Russian sturgeon females aged 6 years. Treatments with hormones were administered at times 0, 3, and 5 weeks. Slow-release salmon GnRH analog implants (10 g/kg), hCG: human chorionic gonadotropin (500 IU/kg, twice, 14 days apart), Met: metoclopramide (20 mg/kg), Ctrl: control (0.2 ml/kg 0.85% saline). Different letters denote substantially different means (p < 0.05) [9] [12].
FSH and LH secretions regulate the synthesis and secretion of gonadal sex steroids in females, with FSH influencing estradiol-17 (E2) (Figure 6) and LH 17,20-dihydroxy-4-pregnen-3-one (17,20β-P) documented exclusively in white sturgeon [9] [52] [53] [54]. The variations in E2 in Russian sturgeon females are neither linear nor optimal for egg development. E2 accumulation did not rise considerably during 4 years, and the increase was gradual. The concentration of E2 in the plasma increased significantly in the fifth and sixth years. At the start of vitellogenesis, the greatest and most significant amount was recorded at 6 years of development (Figure 6) [9] [12]. The rise in plasma 11-ketotestosterone (11-KT) levels was associated with percent GSI and stage of spermatogenesis (Figure 6). With respect to male growth at the Dan Fish Farm (22˚C - 24˚C) [10], mature sperm cells were observed in the testis after around 4 years of development. The findings in Figure 6 confirm the theory that 11-KT is involved in spermatogenesis [9] [12].
Several sex hormones were injected into 4- and 5-year-old Russian sturgeon females to examine whether they can promote puberty under these conditions, but this had no significant effect on oogenesis (Creating caviar). We measured various levels of E2 in the plasma as an important hormone involved in the vitellogenesis process (Figure 6). The injected hormones were: GnRH analog ([D-Arg6, Pro9-NEt]-salmon GnRH; sGnRHa, Bachem Inc., Torrance, CA) in an extended-release implant (Evac), sGnRHa, and the D2 dopamine receptor antagonist metoclopramide, or without human chorionic gonadotropin (hCG) [12]. There was no significant difference in plasma E2 levels at 4 and 5 years after treatment. However, in the plasma of a 6-year-old female treated with GnRHa or GnRHa + metoclopramide, E2 plasma levels were significantly elevated 7 weeks after the first injection (Figure 6) [9] [12]. The added D2 dopamine receptors did not affect E2 levels.
4.4. Growth Hormone in Russian Sturgeon
In vertebrates, GH is a pituitary hormone that controls development and somatic growth [55]. It is a single-chain polypeptide with two disulfide links. IGF-1, which is structurally identical to insulin and has three intramolecular disulfide bonds [56], is the main mediator of GH action. GH is an obvious choice when looking for genetic variables that influence growth rate. This 22-kDa single-chain polypeptide, along with prolactin [57], placental lactogen, and somatolactin [58], belong to a group of similar polypeptide hormones whose sequences appear to have developed from a common ancestor [14]. GH has been widely researched, and various teleost GH cDNA nucleotide sequences are available [57] [59] [60].
The cloning and sequence analysis of full-length cDNA was described for Russian sturgeon growth hormone (rsGH) (EMBL accession no. AY941176). The cDNA was found to be 980 bp in length, with an open reading frame of 642 bp; the first ATG codon was at position 39, and the stop codon was at position 683 [14].
The rsGH-derived amino acid sequence was compared with the homologous GH sequences from several vertebrate species (Figure 7). The rsGH sequence was most similar to that of GH in mammals (66% - 70% identity), followed by Anguilliformes and amphibians (61%), and then other fish (39% - 47%) (Figure 7).
Transcripts of rsGH mRNA in males and females were collected by qRT-PCR (Figure 8). Compared to males, females had higher relative mean levels of rsGH
![]()
Figure 7. Russian sturgeon GH and that of other vertebrates. Dendrograms were created from similarity matrices resulting from alignments of corresponding sequences by the unweighted pair group method and the arithmetic mean method. The scale bar is a measure of the estimated number of amino acid substitutions per 100 residues found when comparing sequences in a pairwise fashion [14].
![]()
Figure 8. Gene transcript levels of GH mRNA in male and female sturgeons during 5 years of growth. Results are expressed as mean ± SEM. No significant difference was found between females and males (p > 0.05 by ANOVA with Student-Newman-Keuls) [14].
mRNA after 3 years of growth than males, but no significant differences were found. This is because the standard error was high, and because Russian sturgeon is newly domesticated and the population still behaves as it would in the wild, with large changes in growth, which may affect the results [14].
4.5. Insulin-Like Growth Factor 1 (IGF-1) Russian Sturgeon
IGF-1 mediates many of the growth-promoting effects of GH. Pituitary GH stimulates the liver and other tissues to produce the mitogenic protein hormone IGF-1. Like mammals and birds, IGF-1 mRNA is produced in a variety of tissues in fish, with the liver being the major site. GH is a major positive regulator of hepatic IGF-1 synthesis [14] [24] [61]. A partial sequence of an IGF-1 cDNA clone was used to measure mRNA levels in various tissues of 1- and 5-year-old male Russian sturgeon. The partial cDNA sequence of IGF-1 was compiled from data obtained from 5’ RACE, and its deduced amino acid sequence is shown in [14].
The cDNA was 445 bp in length, with an open reading frame of 396 bp, and the first ATG codon started at position 56. The predicted position of the signal peptide cleavage site is at this position, resulting in a signal peptide of 44 amino acids. The dendrogram graphically shows the relationship between rsIGF-1 in Russian sturgeon and IGF-1 in other vertebrates (Figure 9) [14] [24] [61].
Figure 10 shows rsIGF-1 mRNA levels measured by qRT-PCR in various tissues of male Russian sturgeon. High transcript levels were detected in the kidneys of adult fish. There were significant differences in IGF-1 mRNA levels in tissues of mature Russian sturgeon (ANOVA; p < 0.05). IGF-1 transcript levels in the kidney, pituitary, and intestine differed significantly from those in other tissues (p < 0.05). Tiny differences in IGF-1 transcription were found in the brain, heart, and muscle, and no significant differences in IGF-1 level of mRNA levels were found in the intestine, pituitary, or liver (p > 0.05; by ANOVA). Moreover, there was no significant difference in mRNA levels between 1-year-old and 5.5-year-old fish (p > 0.05; by ANOVA) [14].
4.6. Vitellogenin (Vg) Levels in Russian Sturgeon Plasma
In fish, vitellogenesis occurs toward the end of oogenesis, when Vg, a liver-produced
![]()
Figure 9. The dendrogram graphically shows the relationship of Russian sturgeon rsIGF-1 to IGF-1 of other vertebrates. Dendrograms were created from similarity matrices resulting from alignments of corresponding sequences by the unweighted pair group method and the arithmetic mean method. The scale bar is a measure of the estimated number of amino acid substitutions per 100 residues found when comparing sequences in a pairwise fashion [14] [24] [61].
![]()
Figure 10. Expression of IGF-1 mRNA in different tissues of 1-year-old Russian sturgeon (young male) and 5.5-year-old male (adult). Total RNA was reverse-transcribed and used for quantitative RT-PCR. The relative amount of rsIGF-1 mRNA was normalized to the amount of 18S rRNA by the threshold cycle method, where 2-ACT reflects the relative amount of Russian sturgeon (rs) IGF-1 transcript. Results are expressed as mean ± SEM (number of juvenile and adult male fish = 10). There were significant differences in IGF-1 transcript levels in different tissues of mature fish (ANOVA; p < 0.05). Transcript levels were significantly different in the kidney, pituitary, and intestine compared to other tissues (p < 0.05; Student-Newman-Keuls) [14].
protein, is released into the blood plasma and accumulates in oocytes as yolk, similar to other vertebrates [62]. Yolk deposition is the term for this effect on oogenesis. This is highly crucial in Russian sturgeon oocytes are used for processing black oocytes or producing caviar (Figure 3) [11] [62]. A few hormones are involved in modulating vitellogenesis in Russian sturgeon, including those of the gonadotropin and somatotropin axes (Figure 3) [62]. The amount of Vg in the Russian sturgeon at various stages of oogenesis was studied under stable aquaculture conditions (18˚C - 22˚C). Gel electrophoresis and Western blot tests were used to determine the amount of Vg in the plasma of a 5-year-old female in the previtellogenic stage and during vitellogenesis [62]. No Vg was found in the previtellogenic stage (Figure 11). A higher Vg level was found in the plasma of oocytes at the yellow oocyte stage and up to the gray oocyte stage. Diagram 2 [63] shows a proposed model for the correlation between vitellogenesis and the two gonadotropin and somatotropin axes.
4.7. Conclusion
In summary, this section describes the connections between somatotropin and gonadotropin, the two hormonal axes that control oogenesis in Russian sturgeon, supported by studies conducted at MIGAL and Dan Fish Farm where Russian sturgeon are farmed under constant conditions for the production of caviar [8] - [12] [14] [15] [24] [25] [26] [63]. The correlation between the two is summarized
![]()
Figure 11. SDS-PAGE of Vg in white sturgeon plasma. As a positive control, a female with an ovary full of gray eggs was used (P, C). The 250-kD band represents the Vg of female sturgeon. Females whose ovaries are full of gray eggs (left panel) were used as positive controls (PC), and male plasma was used as a negative control. Numbers 141 - 154 refer to the individual females sampled, and + or − marks the endoscopically determined gonadal stage. GO—gray oocytes, YO—yellow oocytes, + a few yellow ovaries, and +++, all yellow or gray ovaries [63]. Gel electrophoresis and Western blot tests were used to determine Vg.
in a quality model (Diagram 2) involving somatotropins and gonadotropins in the BPG axis that controls oogenesis.
5. Whole-Genome Sex-Based Variation and Gene Expression in Russian Sturgeon (Acipensergueldenstaedtii)
Early sex determination (ESD) in fish for early male segregation is important to increase the profitability of sturgeon farms, so that more females can be grown and more caviar produced per unit area. This study used data from Russian sturgeon farmed in northern Israel and results from molecular markers developed for ESD. Two sturgeon sex-determination technologies were examined and compared from an economic point of view: 1) Using ESD molecular DNA markers at 0.5 years of age; 2) Using an endoscopic approach at 3.5 years of age, as is currently done on many sturgeon farms. A bioeconomic model of sturgeon farms was developed and an economic assessment of those farms was performed to compare the two technologies. ESD technology significantly improved the economic performance of sturgeon farms.
When caviar costs are lower, this benefit is much larger [20]. The study focused on three important ESD technology indicators: 1) differences in male and female gene expression in various organs; 2) differences in gonad transcription (mRNA level) [17] [18] [27] [64]; 3) genome variation between males and females (DNA sequences) to discover molecular markers [16] [18] [19].
5.1. Gene Transcription Differences in the Gonads of Russian Sturgeon (Acipensergueldenstaedtii) Grown under Stable Aquaculture Conditions
The gonadal transcripts of male and female Russian sturgeon raised under steady aquaculture conditions were investigated in [15] [16] [17] [63].
Two cDNA libraries were prepared from pooled gonadal RNA from five females and four males. After trimming and quality control, 69.7 M and 39.1 M sequenced reads were obtained from the two libraries, respectively. The combination of the two pools assembled the transcriptome, while sex-specific pools were compared to determine the differential abundance of specific transcripts.
Of the 28,170 unique transcripts identified, 16,191 could be annotated by similarity to genes in other species. Homologous sequences were mainly from mammals (71%), fish (13%), amphibians (8%), and reptile sources (6%), with all other sequences accounting for less than 2% (Figure 12) [63]. Between the sexes, 392 transcripts were differentially abundant (20-fold or more); 272 of them were annotated, of which 175 and 97 were upregulated 20-fold or more in the ovary and testis, respectively. A subset of 60 annotated genes with highest relative abundance (based on RNA-seq reads from ovary or testis), were 20-fold or more abundant in the ovary compared to testis.
Other genes involved in sex differentiation in fish previously identified in the gonad transcriptome of Russian sturgeon include sox3, sox9, and star. Among them, the sox9 transcript was 5.6-fold more abundant in the testis compared to ovary; there was no significant difference in star transcript abundance between ovary and testis, and sox3 transcript abundance could not be quantified for either sex. In the samples of this study, other genes previously described to be involved in the sexual differentiation of fish could not be identified in the samples. These included ar, dmrt1, and cyp17a1. Annotation reports and lists of differentially abundant transcripts are included in the Supplementary Information. Functional annotation and clustering of genes with differentially abundant transcripts were identified in several clusters.
Overall, a group of transcriptional regulators and factors involved in cell division, in particular, septins 6, 7, and 9, were more abundant in the ovary, while a diverse set of transcription factors (including sox6 and sox30) and a set of protein kinases were more abundant in the testis [63] [64].
![]()
Figure 12. Summarized distribution of classes in transcript sequences for annotation of sturgeon gonad transcripts identified by RNA-seq. Detailed annotation statistics appear in Supplementary Information [63].
![]()
Figure 13. Transcription of two genes previously known to be involved in reproduction, cyp19 (p450 aromatase) and Foxl2, were also measured by quantitative RT-PCR. Of these, five (including cyp19 and Foxl2) were significantly upregulated in the ovary, while IGHM1 mRNA levels were significantly higher in the testis [63].
5.2. Differential Transcripts by qRT-PCR of Gonad Genes in Russian Sturgeon
To validate several genes with differential transcript abundance identified by RNA-seq in a single sample, qRT-PCR measured nine transcripts with the greatest relative abundance and differential abundances between pooled ovary and testis samples. The relative abundance of transcripts for two genes previously known to be involved in reproduction, cyp19 (p450 aromatase) and foxl2, was also measured in a single sample by qRT-PCR. Of these, 5 transcripts (including cyp19 and foxl2) were relatively more abundant in the ovary, while the ighm1 transcript was relatively more abundant in the testis [17] [63].
5.3. Sex-Based Variation of Gene Transcription in the Fin of Russian Sturgeon
In the fins of 4-year-old males and 7-year-old females, 22 of 32 genes could be reliably quantified by qRT-PCR. Of these, six genes (ATP6, IGFRM, LIS1A, GAPDH, NPL1A, and SOX9) had significantly higher mRNA levels in females than in males [17] (Figure 14). However, when comparing male fin samples with fins from females of similar age (4 years old), the only significant difference in expression was observed for ATP6, which showed approximately 5-fold higher expression in female samples (Figure 15).
5.4. Whole Genome and DNA Variations between the Sexes in Russian Sturgeon (Acipensergueldenstaedtii)
The whole genome of the Russian sturgeon consists of 46 to 590 million 100-bp
![]()
Figure 14. Relative mean mRNA levels (based on qRT-PCR) of the listed genes in fin samples from 11 female (7-year-old) and 8 male (4-year-old) Russian sturgeon. Arrow: p < 0.05 (t-test). Mean + standard error (SE).
![]()
Figure 15. Relative mean mRNA levels (based on qRT-PCR) of the listed genes in fin samples from 10 female (4-year-old) and 8 male (4-year-old) Russian sturgeon (t-test, mean + standard error (SE)). There was no statistically significant difference in mRNA levels between males and females, p > 0.05.
DNA segments. When analyzing the genomic variation between 5 females and 5 males, 536 of these DNA segments differed in females compared to males, and 339 in males compared to females (Figure 16) [16] [18].
DNA expression results of 200 of these segments from the female genomes and a comparison between males and females were performed using RT-PCR. Only the ATP gene showed significantly different expression between the sexes (p < 0.05) (Figure 17, Table 2). Four genes were highly expressed in females (n = 10 females) and 3 in males (n = 10 males).
Figure 18 and Figure 19 show the two genes that had distinct expression in males and females, respectively. Male dissociation curve temperatures differed significantly from those of females (Figure 18 and Figure 19). Males had a melting point of 82.5˚C, while females had a melting point of 74˚C [17].
Ten individual libraries were aligned to the assembled genome using BWA [REF]. In general, the overall alignment rate for all libraries was very high (over 99.6%). According to SAMtools, about 82% - 84% of the reads were correctly paired, and 15% - 17% of them belonged to the category of “with mate mapped to a different chr”, probably because the contigs in the assembled genome were tiny [16]. Genome coverage and average depth were measured by aggregation of individual and sex-specific libraries and all libraries together.
For the 10 individual samples, genome coverage varied from 92.2% to 93.3%, with an average depth of 17.4 to 22.9 reads. There were no significant differences between males and females. The overall coverage for all species was 99.65%, with
![]()
Figure 16. Comparison of 100-bp segments of male and female DNA [16] [18].
![]()
Figure 17. DNA expression of the 100-bp gene fragments showing significant differences between sexes (mean + SE) (see Table 2) [17].
Table 2. Genes showing gonad and fin differences between male and female Russian sturgeon [17].
![]()
![]()
Figure 18. DNA dissociation curves of 10 male segments. No. K119_1143363 was obtained by qPCR [17].
![]()
Figure 19. qPCR DNA dissociation curves from 10 female segments. No. K119_1143363 [17].
an average depth of 165.8 reads (Figure 20) [16] [65].
For the male genome reference, 10 samples had genome coverage ranging from 94.9% to 95.3%, with an average depth ranging from 22.9 to 30.2 reads. No significant differences were observed between female and male libraries. The total coverage of all fish species was 97.5% with an average depth of 228.6 readings (Figure 21 and Figure 22) [16] [65].
In conclusion, at the genome-scale, no significant differences were observed between using male or female genomes as references, or between mapping or coverage results using libraries of different genders.
A previous study identified a conserved female-specific region in the Acipenser ruthenus female genome on contig CACTIG010000179.1: 61,230,000 - 61,285,000. We examined the coverage of libraries in the region in our study. The total coverage for 5 males and 5 females is shown in Figure 23. As can be seen from the figure, the coverage supports the previous findings [65].
![]()
Figure 20. Sequencing depth distribution, all genomic positions, when reads are aligned with the assembled genome, reads cover all genomic positions. (A) Male M1. (B) Female F1. (C) Pooled male samples. (D) Pooled female samples. (AG) Acipenser gueldenstaedtii, references for all 5 males and all 5 females AG genome [16].
![]()
![]()
Figure 21. Sequencing depth distribution across all genome sites when readings are matched to the male reference genome. (A) Male M1. (B) Female F1. (C) Female samples (n=5). Acipeser ruthenus (AR) (references male genome) (AR) combined. (D) Male samples (n=5) (references male genome) (AR) combined [16].
![]()
![]()
Figure 22. When reads from all genome locations are matched to the female reference genome, the distribution of sequencing depth is: (A) Male M1; (B) Female F1; (C) Female samples combined (n = 5) (references females genome (AR)); (D) Male samples combined (n = 5) (references females genome (AR)) [16].
![]()
Figure 23. Female-specific loci were previously discovered in a region of the Acipenser ruthenus female genome. The Y-axis depicts the total coverage, which includes 5 males and 5 females [16].
5.5. Russian Sturgeon Sex Determination Using Molecular Markers
There is a genetic difference between males and females, according to much research. It is feasible to extract a piece of DNA from the Russian sturgeon that also functions as a genetic marker [18] [65] [66] (Figure 24). Endoscopy revealed that the genetic marker AllWSEX2 tested by PCR differs between male and female Russian sturgeon, and Caviar Galilee showed a good correlation with female Russian sturgeon [18] (Figure 24).
As reported by [19], DNA was extracted from 3 females and 3 males, and the products were separated on a 0.5% agarose gel stained with ethidium bromide.
![]()
Figure 24. A PCR assay for determining the sex of the Russian sturgeon.
![]()
Diagram 3. Qualitative description of the sex-determination process.
These findings, together with the capacity to detect a fragment of DNA in each somatic cell, an ancient molecular marker, allow for an identification rate of roughly 80% of the sturgeon’s sex at any age. If put to good use, this discovery is extremely valuable. It presents a strategy for using PCR in Russian sturgeon that might need several improvements (Diagram 3).
6. Conclusion
This paper highlights a significant contribution to the Galilee caviar industry’s joint effort between a research institute (MIGAL) and applied biotechnology. This partnership resulted in the research articles featured in this review, as well as thesis works and other publications. Articles addressing different theoretical and applied aspects of introducing a new species of sturgeon fish (Russian sturgeon), overcoming many issues linked to caviar production and bringing the farm to economic viability were produced as a result of the collaborative work. The information in this report covers all stages of black caviar cultivation under the unique conditions in northern Israel. The primary processes mentioned here, as well as the citations of literature sources, result in the creation of a distinct industrial sector in Israel. Collaboration between the industry and MIGAL has resulted in this sector's success. The topics covered in this review: 1) Food at various stages of cultivation; 2) Sex separation using a marker to distinguish between males and females; 3) Regulation of reproduction under artificial conditions; 4) The role of hormones in egg development and caviar production; 5) Russian sturgeon growth in specified temperature ranges of 18˚C to 21˚C, suggest the relevance of aquaculture, and feasible management strategies for boosting the productivity and profitability of Russian sturgeon culture in Israel.
Acknowledgments
I would like to thank the Ph.D. students working in my laboratory who contributed directly or indirectly to this paper: Dr. Gal Levy, Dr. Karen Jackson, Dr. Svetlana Yom Din, Dr. Tzchori Itai, Dr. David Kushnirove, Dr. Avshalom Hurvitz, Dr. Oren Pearlson, and Dr. Tali Goldberg; and the M.Sc. students, Meital Ezagouri, Lior Sahder, and Dali David; my colleagues at MIGAL and Tel-Hai College Prof. Dani Bercovich, Dr. Doron Goldberg and Dr. Ari Meerson, Prof. Dan Levanon, and my colleagues for their past and current collaboration on the study of fish endocrinology, Prof. Berta Levavi-Sivan, Prof. Yoav Gothilf, Dr. Isana Veksler-Lublinsky, and Prof. Yonathan Zohar.