Novel Tandem Three Consecutive Reactions: Aza-Wittig, Imine Condensation and Electrophilic Aromatic Substitution Strategy to Indolizine Synthesis ()
1. Introduction
Synthetic organic chemistry presents a significant and still growing number of reports focused on the synthesis of molecules with biological and pharmaceutical potential [1] [2] [3] [4] [5]. In particular, the development of new routes to achieve both, new and improved synthesis of these products, is in constant demand [6] [7] [8]. In this context, synthesis of indolizines compounds has been reported using ingenious and sophisticated pyridine starting strategies [9] - [19].
Indolizine synthesis is of growing interest in medicinal chemistry. The indolizines nuclei are present for example in more complex molecules with potent analgesic [20], anti-inflammatory [21] [22] [23] [24], antimicrobial [25] [26], hypoglycemic [27], CNS depressor [28], antioxidant [29] [30] [31] and anti- cancerogenic activities [32] [33] [34], inhibitors that modulate gene transcription [35]. Indolizine derivatives obtaining have become an important topic in organic synthesis.
The indolizine containing molecules then became a challenging synthetic target and several strategic routes to build completely new structures in this family or simply to obtain known molecules in reduced number of steps or avoiding expensive reagents were proposed [36] [37] [38].
In this context, we developed in this study a new strategy toward the title compounds using the iminophosphorane intermediates and their reaction with 2,3-thiophenedicarboxaldehyde. This coupling is leading to the formation of the molecules derived from alkaloids core, specifically that of indolizines.
2. Experimental
2.1. General
All reactions were run under nitrogen atmosphere without moisture. Anhydrous solvents (ethanol, toluene) and reagents were used as from commercial bottles. Melting points were determined with a Kofler Hot Stage Apparatus and were not corrected. The Hydrogen Nuclear Magnetic Resonance (1H NMR) and Carbon-13 Nuclear Magnetic Resonance (13C NMR) spectra were recorded on a Bruker 300 Megahertz (MHz) spectrometer operating at observation frequency of 300 MHz for 1H and 75 MHz for 13C, also was used a Varian 500MHz spectrometer operating at observation frequency of 500 MHz for 1H and 125 MHz for 13C; chemical shifts for hydrogen are given in parts per million (ppm) relatives to tetramethyl silane (TMS) using the central peak of chloroform (CHCl3) (δ = 7.26 ppm) as internal standard. Chemical shifts for 13C NMR spectra are recorded in parts per million from tetramethyl silane using the central peak of CDCl3 (δ = 77.16 ppm) as the internal standard, all 13C NMR spectra were obtained with complete proton decoupling.
2.2. Synthesis of Indolizine Compounds
General procedure for the synthesis of indolizine compounds
A mixture of 0.1 mmol of the iminophosphorane intermediate (1-10) (Scheme 1 and Table 1) and 0.1 mmol of the corresponding 2,3-thiophenecarboxaldehyde in 2 mL of xylene was refluxed for 48 h. The mixture was allowed to reach the room temperature and then purified by column chromatography (silica gel 70 - 230 mesh) yielding solids in all cases (19-37).
Ethyl bisthieno [3,2-a:2,3-g]indolizine-5-carboxylate (19). Red solid yield 89%. Low-Resolution Mass Spectrometry (LRMS) Electronic Impact (E.I.) m/z (%): 301 (M+, 100), 273 (42); C15H10BrNO2S2; Molecular weight (MW) 380.28 g/mol. Fourier Transform Infrared (FTIR) (cm−1): ν = 3182, 3109, 1706, 1294, 1193, 1153, 1095, 1060. RMN 1H 500 MHz (CDCl3) (ppm) δ: 1.49 (t, 3H, J = 7.0 Hz); 4.48 (q, 2H, J = 7.0 Hz); 7.19 (d, 1H, J = 5.5 Hz); 7.49 (dd, 1H, J = 5.0, 0.5 Hz); 7.62 (dd, 1H, J = 5.0, 1.0 Hz); 7.76 (d, 1H, J = 5.0 Hz); 8.21 (d, 1H, J = 0.5 Hz); 9.02 (d, 1H, J = 0.5 Hz). RMN 13C 125 MHz (CDCl3) (ppm) δ: 14.35; 61.59; 106.15; 114.86; 116.26; 121.70; 122.30; 125.35; 125.68; 128.19; 131.45; 133.3; 137.0, 137.2; 162.79.
Ethyl bisthieno [2,3-a:2,3-g]indolizine-5-carboxylate (20). Red solid yield 89%. LRMS (E.I.) m/z (%): 301 (M+, 100), 273 (42); C15H10BrNO2S2; MW 380.28 g/mol. FT-IR ATR (cm−1): ν = 3182, 3109, 1706, 1294, 1193, 1153, 1095, 1060. RMN 1H 500 MHz (CDCl3) (ppm) δ: 1.48 (t, 3H, J = 7.0 Hz); 4.49 (q, 2H, J = 7.0 Hz); 7.26 (d, 1H, J = 5.5 Hz); 7.35 (d, 1H, J = 5.0 Hz); 7.75 (d, 1H, J = 5.0, Hz); 7.77 (dd, 1H, J = 5.0, 1.0 Hz); 8.22 (d, 1H, J = 0.5 Hz); 9.09 (s, 1H). RMN 13C 125 MHz (CDCl3) (ppm) δ: 14.35; 61.63; 106.50; 115.15; 116.53; 121.45; 122.3; 125.36; 125.68; 128.2; 131.45; 133.28; 137.00; 137.2, 162.8.
Ethyl bisthieno [3,2-a:3,2-g]indolizine-5-carboxylate (21). Red solid yield 70%. LRMS (E.I.) m/z (%): 301 (M+, 100), 273 (50); C15H10BrNO2S2; MW 380.28 g/mol. FT-IR ATR (cm−1): ν = 3007, 2929, 1702, 1627, 1469, 1447, 1370, 1194. 1159, 1095. RMN 1H 500 MHz (CDCl3) (ppm) δ: 1.48 (t, 3H, J = 7.0 Hz); 4.49 (q, 2H, J = 7.0 Hz); 6.67 (d, 1H, J = 5.5 Hz); 6.76 (d, 1H, J = 5.5, Hz); 6.87 (d, 1H, J = 5.0 Hz); 6.98 (dd, 1H, J = 5.0, 1.0 Hz); 8.22 (d, 1H, J = 1.0 Hz); 9.09 (d, 1H, J = 0.5 Hz). RMN 13C 125 MHz (CDCl3) (ppm) δ: 14.15; 61.19; 107.13; 115.49; 116.36; 119.11; 119.53; 121.84; 122.85; 124.21; 124.7; 126.4; 130.74; 135.12, 162.82.
Ethyl bisthieno [2,3-a:3,2-g]indolizine-5-carboxylate (22). Red solid yield 70%. LRMS (E.I.) m/z (%): 301 (M+, 100), 273 (50), C15H10BrNO2S2; MW 380.28 g/mol. FT-IR ATR (cm−1): ν = 3007, 2929, 1702, 1627, 1469, 1447, 1370, 1194. 1159, 1095. RMN 1H 500 MHz (C6D6) (ppm) δ: 1.47 (t, 3H, J = 7.0 Hz); 4.08 (q, 2H, J = 7.0 Hz); 6.65 (d, 1H, J = 5.5 Hz); 6.77 (d, 1H, J = 5.0, Hz); 6.98 (2H); 7.32 (dd, 1H, J = 5.0, 1.0 Hz); 7.89 (d, 1H, J = 1.0 Hz); 9.092 (d, 1H, J = 1.0 Hz). RMN 13C 125 MHz (CDCl3) (ppm) δ: 14.1; 62.2; 107.5; 115.8; 116.8; 119.1; 119.9; 121.8; 122.7; 123.3; 124.6; 129.8; 131.7; 136.2, 162.4.
Ethyl 1-methyldithieno[3,2-a:2,3-g]indolizine-5-carboxylate (23). Orange solid, yield 65%. Melting point 115˚C - 118˚C. LRMS (E.I.) m/z (%): 315 (M+, 10), 243 (100); C16H13NO2S2; MW 315.41 g/mol. FT-IR ATR (cm−1): ν = 3074, 3056, 2977, 2924, 2856, 1690 (C = O) 1284, 691. RMN 1H 300MHz (CDCl3) (ppm) δ: 1.4 (t, 3H, J = 9.0 Hz); 2.37 (s, 3H); 4.3 (q, 2H, J = 9.0 Hz); 7.05 (s, 1H,); 7.18 (s, 1H); 7.34 (s, 1H); 7.51 (d, 1H, J = 5.0 Hz); 7.94 (d, 1H, J = 5.0 Hz). RMN 13C 300 MHz (CDCl3) (ppm) δ: 14.1; 16.4; 61.5; 111.5; 118.99; 119.13; 120.73; 123.92; 124.07; 125.03; 128.05; 128.74; 128.78; 129.83; 134.63; 139.8; 159.7.
Ethyl 1-methyldithieno[2,3-a:2,3-g]indolizine-5-carboxylate (24). Orange solid, yield 65%. Melting point 115˚C - 118˚C. LRMS (E.I.) m/z (%): 315 (M+, 10), 243 (100); C16H13NO2S2; MW 315.41 g/mol. FT-IR ATR (cm−1): ν = 3074, 3056, 2977, 2924, 2856, 1690, 1284, 691. RMN 1H 300MHz (CDCl3) (ppm) δ: 1.4 (t, 3H, J = 9.0 Hz); 2.4 (s, 3H); 4.3 (q, 2H, J = 9.0 Hz); 7.06 (s, 1H); 7.26 (s, 1H); 7.32 (d, 1H, J = 5.5 Hz); 7.34 (s, 1H); 7.71 (d, 1H, J = 5.5 Hz). RMN 13C 300 MHz (CDCl3) (ppm) δ: 14.1; 16.5; 61.4; 111.5; 118.9; 119.13; 120.73; 123.92; 124.07; 125.03; 128.05; 128.74; 128.78; 129.83; 134.63; 136; 139.4; 159.9.
Ethyl 1-bromobisthieno [3,2-a:2,3-g]indolizine-5-carboxylate (25). Red solid, yield 82%. Melting point 96˚C - 98˚C. LRMS (E.I.) m/z (%): 381 (M+, 10), 43 (100); C15H10BrNO2S2; MW 380.28 g/mol. FT-IR ATR (cm−1): ν = 3171, 3092, 2957, 2924, 2855, 1706, 1209, 742. RMN 1H 300 MHz (CDCl3) (ppm) δ: 1.46 (t, 3H, J = 6.0 Hz); 4.46 (q, 2H, J = 9.0 Hz); 7.19 (d, 1H, J = 5.0 Hz); 7.30 (d, 1H, J = 5.0 Hz); 7.69 (s, 1H); 8.11 (s, 1H); 9.19 (s, 1H). RMN 13C 300 MHz (CDCl3) (ppm) δ: 13.73; 61.31; 106.82; 114.01; 114.98; 120.61; 121.37; 122.18; 124.20; 127.45; 128.19; 130.28; 131.83; 135.30; 161.84.
Ethyl 1-bromobisthieno [2,3-a:2,3-g]indolizine-5-carboxylate (26). Red solid, yield 82%. Melting point 96˚C - 98˚C. LRMS (E.I.) m/z (%): 381 (M+, 10), 43 (100); C15H10BrNO2S2; MW 380.28 g/mol; yield 82%. FT-IR ATR (cm−1): ν = 3171, 3092, 2957, 2924, 2855, 1706, 1209, 742. RMN 1H 300 MHz (CDCl3) (ppm) δ: 1.46 (t, 3H, J = 6.0 Hz); 4.46 (q, 2H, J = 9.0 Hz); 7.52 (d, 1H, J = 5.0 Hz); 7.54 (dd, 1H, J = 0.5, 5.0 Hz); 7.71 (s, 1H); 8.09 (d, 1H, J = 0.5 Hz); 9.20 (s, 1H). RMN 13C 300 MHz (CDCl3) (ppm) δ: 13.45; 61.24; 106.82; 113.85; 114.01; 114.98; 121.37; 122.18; 124.20; 127.45; 128.19; 130.05; 131.83; 135.30; 161.84.
Ethyl 1-phenyldithieno[3,2-a:2,3-g]indolizine-5-carboxylate (27). Brown solid, yield 81%. Melting point 114˚C - 118˚C. LRMS (E.I.) m/z (%): 377 (M+, 10), 43 (100); C21H15NO2S2; MW 377.48 g/mol. FT-IR ATR (cm−1): ν = 3055, 2958, 2926, 2871, 1692 (C = O) 1212, 691. RMN 1H 300 MHz (CDCl3) (ppm) δ: 1.33 (t, 3H, J = 6.0 Hz); 4.28 (q, 2H, J = 9.0 Hz); 7.34 - 7.38 (m, 2H); 7.45 - 7.50 (m, 5H); 7.57 (d, 1H, J = 6.0 Hz); 7.73 (s, 1H); 8.01 (s, 1H). RMN 13C 300 MHz (CDCl3) (ppm) δ: 14.1; 61.4; 111.7; 117.8; 118.2; 119.4; 120.9; 123.9; 125.2; 126.9; 127.8; 128.6; 130.7; 131.6; 131.7; 133.2; 133.5; 135.2; 142.6; 160.7.
Ethyl 1-phenyldithieno[2,3-a:2,3-g]indolizine-5-carboxylate (28). Brown solid, yield 81%. Melting point 114˚C - 118˚C. LRMS (E.I.) m/z (%): 377 (M+, 10), 43 (100); C21H15NO2S2; MW 377.48 g/mol. FT-IR ATR (cm−1): ν = 3055, 2958, 2926, 2871, 1692, 1212, 691. RMN 1H 300 MHz (CDCl3) (ppm) δ: 1.35 (t, 6H, J = 6.0 Hz); 4.28 (q, 2H, J = 9.0 Hz); 7.34 - 7.38 (m, 2H,); 7.45 - 7.50 (m, 6H); 7.57 (d, 1H, J = 6.0 Hz); 7.75 (s, 1H); 8.03 (s, 1H). RMN 13C 300 MHz (CDCl3) (ppm) δ: 14.13; 61.45; 111.78; 117.89; 118.23; 119.45; 120.98; 123.92; 125.23; 126.93; 127.83; 128.63; 130.70; 131.66; 131.77; 133.20; 133.95; 135.20; 141.95; 161.9.
Ethyl 2-phenyldithieno[3,2-a:2,3-g]indolizine-5-carboxylate (29). Brown solid, yield 78%. Melting point 114˚C - 118˚C. MS (IE) m/z (%): 377 (M+,10); 243 (100); C21H15NO2S2; MW 377.48 g/mol. FT-IR ATR (cm−1): ν = 3055, 2955, 2921, 2851, 1690, 1205, 690. RMN 1H 300 MHz (CDCl3) (ppm) δ: 1.33 (t, 3H, J = 6.0 Hz); 4.27 (q, 2H, J = 6.0 Hz); 7.36 (s, 1H, 138a y 138b); 7.43 - 7.46 (m, 6H); 7.47 (d, 1H, J = 5.0 Hz); 7.76 (d, 1H, J = 0.5, 5.0 Hz); 8.02 (d, 1H, J = 0.5 Hz); 8.38 (s, 1H). RMN 13C 300 MHz (CDCl3) (ppm) δ: 14.16; 61.47; 105.15, 111.85; 120.24; 120.25; 121.40; 124.84; 126.84; 128.60; 129.17; 130.34; 131.60; 134.08; 134.63; 135.15; 137.63; 139.23; 159.7.
Ethyl 2-phenyldithieno[2,3-a:2,3-g]indolizine-5-carboxylate (30). Brown solid, yield 78%. Melting point 114˚C - 118˚C. MS (IE) m/z (%): 377 (M+,10); 243 (100); C21H15NO2S2; MW 377.48 g/mol. FT-IR (cm−1): ν = 3055, 2955, 2921, 2851, 1690, 1205, 690. RMN 1H 300 MHz (CDCl3) (ppm) δ: 1.33 (t, 3H, J = 6.0 Hz); 4.27 (q, 2H, J = 6.0 Hz); 7.36 (d, 1H, J = 0.5 Hz); 7.41 - 7.49 (m, 6H,); 7.61 (d, 1H, J = 5.0 Hz); 8.0 (d, 1H, J = 0.5 Hz); 8.4 (s, 1H). RMN 13C 300 MHz (CDCl3) (ppm) δ: 14.13; 61.45; 111.85; 120.24; 120.25; 121.40; 124.84; 126.84; 126.92; 128.60; 129.17; 130.34; 131.60; 134.08; 134.63; 135.15; 137.63; 139.23; 159.9.
Ethyl furo[2,3-g]thieno[3,2-a]indolizine-5-carboxylate (31). Yellow solid, yield 62%. Melting point 110˚C - 112˚C. LRMS (E.I.) m/z (%): 285 (M+, 10), 149 (100); C15H11NO3S; MW 285.32 g/mol. FT-IR (cm−1): ν = 3396, 3185, 3114, 2950, 2926, 2856, 1690, 1198. RMN 1H 300MHz (CDCl3) (ppm) δ: 1.33 (t, 3H, J = 6.0 Hz); 4.26 (q, 2H, J = 9.0 Hz); 7.10 (d, 1H, J = 5.0 Hz); 7.16 (s, 2H); 7.69 (d, 1H, J = 6.0 Hz); 8.07 (s, 1H); 9.05 (d, 1H, J = 0.5 Hz). RMN 13C 300 MHz (CDCl3) (ppm) δ: 14.38; 61.63; 108.42; 109.36; 119.53; 120.09; 122.82; 124.48; 125.19; 132.47; 137.8; 138.24; 144.47; 154.09; 167.01.
Ethyl furo[2,3-g]thieno[2,3-a]indolizine-5-carboxylate (32). Yellow solid, yield 62%. Melting point 110˚C - 112˚C. LRMS (E.I.) m/z (%): 285 (M+, 10), 149 (100); C15H11NO3S; MW 285.32 g/mol. FT-IR (cm−1): ν = 3396, 3185, 3114, 2950, 2926, 2856, 1690, 1198. RMN 1H 300 MHz (CDCl3) (ppm) δ: 1.33 (t, 3H, J = 6.0 Hz); 4.26 (q, 2H, J = 9.0 Hz); 7.04 (d, 1H, J = 5.0 Hz); 7.45 (d, 1H, J = 5.0 Hz); 7.52 (d, 1H, J = 5.0 Hz); 7.80 (d, 1H, J = 3.0 Hz); 8.07 (s, 1H); 9.12 (s, 1H). RMN 13C 300 MHz (CDCl3) (ppm) δ: 14.07; 61.57; 96.14; 119.53; 121.02; 122.82; 124.48; 125.19; 131.32; 132.47; 137.8; 138.24; 144.13; 154.09; 167.01.
Ethyl 2-phenylfuro[2,3-g]thieno[3,2-a]indolizine-5-carboxylate (33). Beige solid, yield 62%. Melting point 113˚C - 115˚C. LRMS (E.I.) m/z (%): 361 (M+, 10), 149 (100); C21H15NO3S; MW 361.45 g/mol. FT-IR (cm−1): ν = 3396, 3185, 3114, 2958, 2826, 2856, 1690, 1198, 756. RMN 1H 300 MHz (CDCl3) (ppm) δ: 1.33 (t, 3H, J = 6.0 Hz); 4.26 (q, 2H, J = 9.0 Hz); 7.40 - 7.47 (m, 7H); 7.78 (d, 1H, J = 5.0 Hz); 8.07 (d, 1H, J = 0.5 Hz); 8.3 (s, 1H). RMN 13C 300 MHz (CDCl3) (ppm) δ: 14.38; 61.63; 96.14; 108.42; 109.36; 119.53; 120.09; 121.02; 122.82; 124.48; 125.19; 131.32; 132.47; 137.81; 138.24; 144.13; 144.47; 154.09; 167.01.
Ethyl 2-phenylfuro[2,3-g]thieno[2,3-a]indolizine-5-carboxylate (34). Beige solid, yield 62%. Melting point 113-115˚C. LRMS (E.I.) m/z (%): 361 (M+, 10), 149 (100); C21H15NO3S; MW 361.45 g/mol. FT-IR (cm−1): ν = 3396, 3185, 3114, 2958, 2826, 2856, 1690, 1198, 756. RMN 1H 300 MHz (CDCl3) (ppm) δ: 1.33 (t, 3H, J = 6.0 Hz); 4.26 (q, 2H, J = 6.0 Hz); 7.33 (s, 1H); 7.40 - 7.47 (m, 5H); 7.6 (d, 1H, J = 5.0 Hz); 7.80 (d, 1H, J = 5.0 Hz); 8.09 (s, 1H); 8.28 (s, 1H). RMN 13C 300 MHz (CDCl3) (ppm) δ: 14.07; 61.57; 96.14; 108.42; 109.36; 119.53; 120.09; 121.02; 122.82; 124.48; 125.19; 131.32; 132.47; 137.81; 138.24; 144.13; 144.47; 154.09; 167.01.
Ethyl 3-methyl-3H-pyrrolo[2,3-g]thieno[3,2-a]indolizine-5-carboxylate (35). Red solid, yield 53%. Melting point 110˚C - 112˚C. LRMS (E.I.) m/z (%): 299 (M+, 32), 225 (100); C16H14N2O2S; MW 298.36 g/mol. FT-IR (cm−1): ν = 3056, 2980, 2951, 2924, 1733, 1182, 719. RMN 1H 300 MHz (CDCl3) (ppm) δ: 1.37 (t, 3H, J = 6.0 Hz); 3.62 (s, 3H); 4.30 (q, 2H, J = 6.0 Hz); 6.72 (d, 1H, J = 4.0 Hz); 6.81 (s, 1H); 7.39 (d, 1H, J = 4.0 Hz); 7.45 (s, 1H); 7.56 (d, 1H, J = 5.5 Hz); 7.94 (d, 1H, J = 5.5 Hz). RMN 13C 75 MHz (CDCl3) (ppm) δ: 14.13; 36.16; 61.45; 111.15; 118.99; 120.73; 123.92; 124.07; 125.03; 128.05; 128.78; 129.83; 130.79; 134.63; 139.84; 160.9.
Ethyl 3-methyl-3H-pyrrolo[2,3-g]thieno[3,2-a]indolizine-5-carboxylate (36). Red solid, yield 53%. Melting point 110˚C - 112˚C. LRMS (E.I.) m/z (%): 299 (M+, 32), 225 (100); C16H14N2O2S; MW 298.36 g/mol. FT-IR (cm−1): ν = 3056, 2980, 2951, 2924, 1733, 1182, 719. RMN 1H 300 MHz (CDCl3) (ppm) δ: 1.37 (t, 3H, J = 6.0 Hz); 3.6 (s, 3H); 4.30 (q, 2H, J = 6.0 Hz); 6.44 (d, 1H, J = 3.0 Hz); 6.81 (s, 1H); 6.94 (d, 1H, J = 3.0 Hz); 7.45 (s, 1H); 7.71 (d, 1H, J = 5.0 Hz); 7.80 (d, 1H, J = 5.0 Hz). RMN 13C 75 MHz (CDCl3) (ppm) δ: 14.13; 36.16; 61.45; 111.15; 118.99; 120.73; 123.92; 124.07; 125.03; 128.74; 128.78; 129.83; 130.79; 134.63; 139.84; 159.8.
Ethyl 3-benzyl-3H-pyrrolo[2,3-g]thieno[3,2-a]indolizine-5-carboxylate (37). Red solid, yield 49%. Melting point 80˚C - 82˚C. LRMS (E.I.) m/z (%): 374 (M+, 100), 301 (54); C22H18N2O2S; MW 374.45 g/mol. FT-IR (cm−1): ν = 3093, 2985, 2951, 2923, 1730, 1102, 729. RMN 1H 300 MHz (CDCl3) (ppm) δ: 1.42 (t, 3H, J = 7.0 Hz); 4.43 (q, 2H, J = 7.0 Hz); 5.18 (s, 2H); 6.72 (d, 1H, J = 3.0 Hz); 6.93 (d, 1H, J = 3.0); 7.19 (d, 1H, J = 5.0 Hz); 7.28-30 (m, 6H); 7.62 (s, 1H); 8.51 (s, 1H). RMN 13C 75 MHz (CDCl3) (ppm) δ: 14.1; 52.7; 60.53; 101.51; 108.67; 119.92; 120.36; 123.02; 124.07; 125.03; 125.7; 127.32; 128.74; 128.78; 129.83; 130.79; 134.63; 137.3; 139.84; 159.8.
3. Results
Our group has already been interested in iminophosphorane chemistry. For example, these substrates were used in the synthesis of several heterocyclic products such as thienopyridines [39]. In this work we report a new Tandem process of three consecutive reactions: aza-Wittig, Imine condensation and Electrophilic Aromatic Substitution, for obtaining heterocyclic structures of indolizines.
3.1. Iminophosphoranes Intermediates Synthesis
First, the starting aromatic/heteroaromatic iminophoshorane acrylate intermediates were constructed through two consecutive reactions. Aromatic/ heteroaromatic aldehydes were the starting materials, which through an initial aldol condensation reaction with ethyl azido acetate in a basic ethanolic medium produced the corresponding aryl/heteroaryl azido acrylates. Later, with the use of triphenylphosphine, the azide group was transformed to the intermediated aryl/heteroaryl iminophosphoranes (1-11, Scheme 1). The overall yields of these two consecutive reactions for the iminophosphoranes intermediates are good, ranging from 76% to 95% (see Table 1).
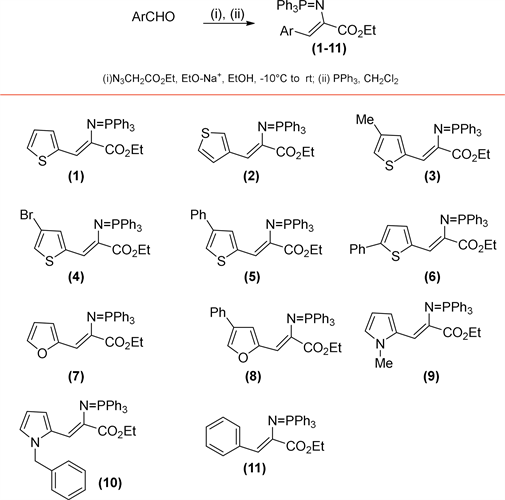
Scheme 1. Intermediates iminophosphoranes formed by two consecutive reactions from aldehydes. (i) aldolic condensation, (ii) Staudinger reaction.
![]()
Table 1. Iminophosphoranes intermediates synthesized (see Scheme 1).
a. Yield for isolated compound.
3.2. Isoindolones Strategy
Once the iminophosphoranes intermediates were obtained, the work was focused on studying their reactivity with different ortho-dialdehydes. In previous studies reported, the reaction of iminophosphoranes with aromatic dialdehydes produces the corresponding isoindolones [40]. Our first experiments were designed to test the reactivity of iminophosphoranes intermediates with phtalaldehyde, 2,3-Naphthalenedicarboxaldehyde and 2,3-thiophenecar-boxalde- hyde. The results obtained shown that the phtalaldehyde reaction with any type of iminophosphoranes intermediate results in the corresponding isoindolone (12, 13 and 15, Scheme 2), same results were observed with 2,3-Naphthalenedicarboxal- dehyde (14 and 16, Scheme 2). Furthermore, when the reaction occurs between 2,3-thiophenedicarboxaldehyde with ethyl (Z)-3-phenyl-2-((triphenyl-l5-phospha- neylidene)amino)acrylate (11), is also observed the formation of the isoindolones (17 and 18, Scheme 2). These results proven that any combination in which the benzene rings is present, both in the iminophosphoranes intermediate and the dialdehyde, results in the formation of the isoindolone heterocycle. Yields for these reactions agree with those reported in previous works (Table 2) [40].
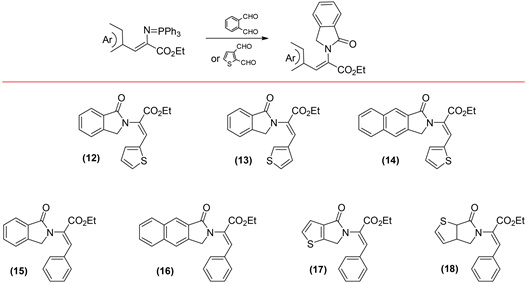
Scheme 2. Isoindolones formed by iminophosphorane intermediates (1, 2, 11) and phtaladehyde, 2,3-Naphthalenedicarboxaldehyde and thiophene-2,3-dicarboxaldehyde reaction.
![]()
Table 2. Iminophosphorane reactivity using aromatic or heteroaromatic dialdehydes (see Scheme 2).
a. Yield for isolated derivatives.
3.3. Indolizine Protocol
During the study of iminophosphoranes intermediates reactivity with aromatic dialdehydes, we carry out the reaction between the iminophosphoranes ethyl (Z)-3-(thiophen-2-yl)-2-((triphenyl-l5-phosphaneylidene)amino)acrylate (1) with 2,3-thiophendicarboxaldehyde, detecting a different product from that isoindolones previously observed, the structural elucidation showed a indolizine heterocycle as the product of this reaction.
This reaction seems to indicate that existence of thiophen moiety on both reagents, dialdehyde and iminophophoranes, changes the reaction course toward production of indolizine rather than isoindolone compounds earlier reported. An important characteristic of this reaction was the obtaining of indolizine as a pair of structural isomers (19 and 20, Scheme 3), in a proportion of 50% each.
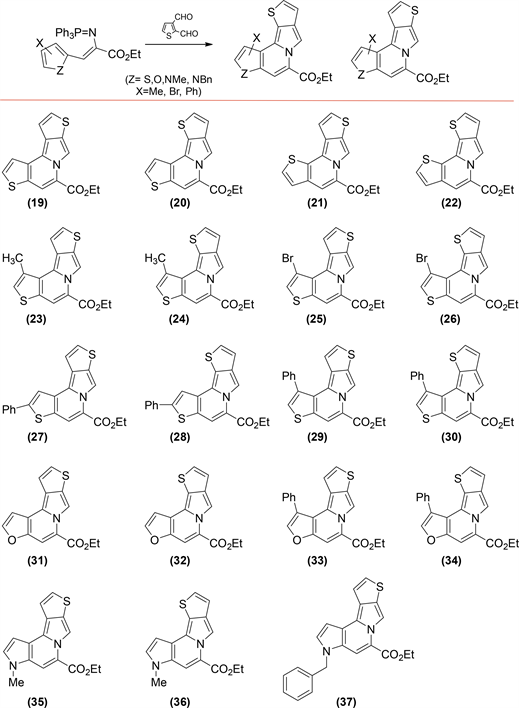
Scheme 3. Indolizines formed by iminophosphorane heteroaromatic intermediates (1-10) and, 2,3-thiophenedicarboxaldehyde reaction.
We were then essentially interested in the formation of this latter family of indolizine compounds; whereby we chose several iminophosphorane intermediates with thiophene as the central heterocycle. Initially, the isomer derived from 3-thiophenyl (2) was chosen; as well as the 2-thiophenyl derivatives substituted in position four with methyl (3), bromine (4), and phenyl (6) groups, and finally the derivative of 2-thiophenyl substituted in position five by phenyl group (5) (Scheme 1).
Continuing with the study for the formation of indolizine, the reaction of iminophosphorane 2 with 2,3-thiophenedicarboxaldehyde was carried out, resulting in indolizine as its structural isomer pair (21 and 22, Scheme 3). Thiophene iminophosphorane derivatives 3 to 6 were reacted with 2,3-thiophenedicarboxaldehyde finding the same result, the formation of indolizine as its pair of isomers in the same proportion each (23-30, Scheme 3). The yields for this indolizines 19-30 shown in Table 3 (entries 1-6) are good ranging from 70% to 82%.
Understanding that aromatic carbocycles as part of iminophosphoranes or dialdehydes are not suitable materials for indolizine formation, we set out to carry out this strategy using furans (7 and 8, Scheme 1) and pyrrole (9 and 10, Scheme 1) heterocycles instead thiophene moiety in iminophosphoranes. The results shown that indolizine are obtained as its two structural isomers when the iminophosphoranes with pyrrole or furan react with 2,3-thiophenedicarboxaldehyde but yields of indolizines dropped to 50% - 60% level (entries 7 - 10, Table 3), means lower than for more aromatic thiophene analog. Finally, the mechanism of this tandem aza-Wittig reaction of thiophenyliminophosphorane acrylates with 2,3-thiophenedicarboxyaldehyde leading to indolizines should be re-examined in view of electrocyclic closure-SE hypothesis, because of puzzling high aromaticity of thiophene ring factor.
3.4. Proposed mechanism
According to this mechanism (as highlighted in Scheme 3 and detailed in
a. Yield for isolated compounds.
Schemes 4 and Scheme 5) the initial attack of the iminophosphorane can take place on the electrophilic carbon of aldehyde group on C-2, then, via betaine (i) to a formation of oxazaphosphetane ring (ii) followed by elimination to imine (iii). The presented mechanism (Scheme 4) is showing the initial attack on C-2. The reverse sequence, on carbon C-3 aldehyde will produce the isomeric indolizine (Scheme 5).
This sequence of reactions can occur on either of two aldehyde groups, 2-aldehyde or 3-aldehyde, because of already stated similar activation of these two carbons exercised by sulfur pulling effect. According to the initial two aldehyde site attacks, two isomeric indolizine skeletons, which differ in the position of sulfur on the thiophene ring (S-6 or S-4) could be obtained. The calculated total energies of these two isomers are very close (Hyper Chem 7.02 Mm+, ∆E = 0.4 kcal/mole, 19 and 20, Scheme 4 and Scheme 5).
The final proposal for this mechanism outcome involves an intramolecular
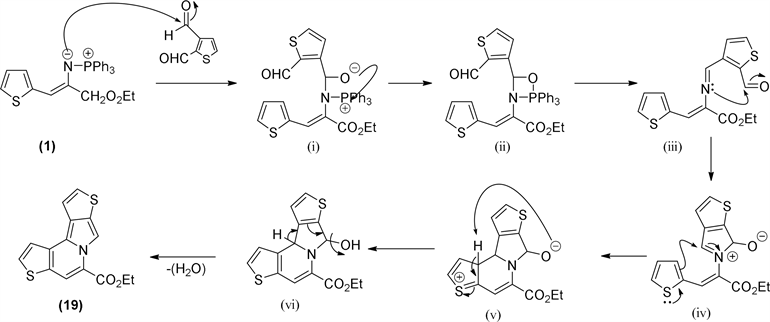
Scheme 4. Proposed mechanism for ethyl bisthieno[3,2-a:2,3-g]indolizine-5-carboxylate (19) isomer formation.

Scheme 5. Proposed mechanism for ethyl bisthieno[2,3-a:2,3-g]indolizine-5-carboxylate (20) isomer formation.
imine (iii) reaction with the remaining aldehyde to generate a reactive iminium intermediate (iv). Interestingly, this same intermediate has been involved on isoindolone formation; the main change here is that the iminium intermediate undergoes an aromatic electrophilic substitution on the thiophene ring to give the system of four fused heterocyclic rings (v) which is finally dehydrated to aromatize the entire indolizine system.
3.5. Spectroscopic Differentiation of Structural Isomers
In a series of reported reactions, summarized in Scheme 3 and Table 3, the formation of two isomeric indolizines was found. Table 4 describes the detailed examination of NMR spectra for the indolizines 19 and 20 obtained. Differentiating the 19 and 20 isomers was made possible by their long-distance spin
![]()
Table 4. NMR data characterization for two indolizine structural isomers.
a. W coupling.
coupling systems. For example, in isomer 19 two long-distance couplings are observed in W, first hydrogen on C1 occurs as a double of double system due to three bond coupling with hydrogen on C2 (3J = 5.5 Hz), but also hydrogen on C1 has a long-distance coupling in W with hydrogen on C5 (5J = 0.5 Hz). Second, the hydrogen on C12 in isomer 19 is a double of double system presenting a three-bond coupling with the hydrogen on C11 (3J = 5.5 Hz), and the W long-distance coupling with hydrogen on C8 (5J = 0.5 Hz).
On other hand, isomer 20 only exhibits one W long-distance coupling, hydrogen on C12 a double of double system has three-bonds coupling with hydrogen on C11(3J = 5.0 Hz) and a W long-distance coupling with hydrogen on C8 (5J = 1.0 Hz). The number of long-distance couplings is therefore the key to the unequivocal isomer’s differentiation. This observation shown for isomers 19 and 20, applies to the rest of the isomers obtained in this series with different starting heterocycles materials.
4. Conclusions
Nineteen indolizine derivatives (eight pairs of structural isomers, Scheme 3) were obtained using various heterocyclic compounds containing thiophene, furane or pyrrole moieties. This synthetic route represented an advantage over previously reported, since it was carried out in a single reaction step from the generally easy synthesized iminophosphoranes.
In this synthesis, we faced a problem of orientation of aza-Wittig tandem reaction toward isoindolone or indolizine. For this last family compound two isomeric structures are formed, this corresponding to the initial attack on C-2 of thiophene aldehyde, to obtain the isomers 19, 21, 23, 25, 27, 29, 31, 33 and 35, or the initial attack on C-3 of thiophene aldehyde to produce the isomers 20, 22, 24, 26, 28, 30, 32, 34 and 36.
The mechanism explaining the formation of indolizine core compounds, over isoindolone, obtained in this reaction is proposed according to Novel Tandem three consecutive reactions, aza-Wittig, Imine Condensation and Electrophilic Aromatic Substitution Strategy. Finally, it was clear that the activation role of thiophene ring, as a sulfur containing five-member heterocycle, is essential to the success of this synthesis. The same phenyl containing iminophosphoranes or dialdehydes does not produce the indolizine of core products.
Acknowledgements
A financial support from UAEMex (Project No. 4734/2019CIB) and CONACyT (postgraduate scholarship) is gratefully acknowledged. The authors would like to thank M.N. Zavala-Segovia, L. Triana-Cruz (CCIQS UAEM-UNAM) for the technical support.