Response of Salt-Tolerant Rice Varieties to Biocompost Application in Sodic Soil of Eastern Uttar Pradesh ()
1. Introduction
Rice (Oryza sativa L.) is the staple food of 2.7 billion people in the world and cultivated in all the continents except Antarctica, covering tropical and sub-tropical regions between 55˚ North and 36˚ South in a variety of ecosystems, ranging from favourable irrigated to rainfed uplands and lowlands, flash flood and deep water areas [1]. In recent years, rice occupied about 154 million ha area with an average productivity of 3.83 t ha−1. Rice cultivation is of immense importance to food security of Asia, where more than 90% of the global rice is produced and consumed, and which is the home of about 70% impoverished people of the world. Rice is essential for feeding the world’s population, and is especially important in Asian countries. It plays a major role as a staple food, supporting more than 3 billion people [2].
Salt-affected soils occur across continents and under almost all climatic conditions. More than 800 million ha of land throughout the world are salt affected [3]. Among South Asian countries, India has the largest area (6.73 million ha), followed by Bangladesh (1 million ha). In India alone, 1.4 million ha are characterized by coastal salinity, and inland alkaline (sodic) and saline soils (referred to as “Usar” in the local dialect) cover about 5.33 million ha. Many of these salt-affected areas are either barren or have very low and unstable productivity, particularly in coastal deltas. Rice has previously been reported as being salt susceptible in the early seedling and reproductive stages [4,5], leading to yield reduction of more than 50% in crops exposed to 6.65 dS m−1 EC [6]. Salt affected soils often have multiple constraints including high pH, high soluble salts, deficiency and toxicity of one or several micronutrients and poor water holding capacity, leading to low productivity. It causes a billion dollar losses in annual crop production globally.
Soil salinity is a major abiotic stress limiting plant growth and development. In crops known as glycophyte or salt susceptible [7,8], it causes yield losses by depressing the uptake of water, and disturbing mineral and normal metabolism. Salt-affected soils are identified by excessive levels of water-soluble salts, especially sodium chloride (NaCl) [9]. NaCl is a small molecule which when ionized by water, produces sodium (Na+) and chloride (Cl−) ions. Excess Na+ in plant cells directly damages membrane systems and organelles, resulting in growth reduction and abnormal development prior to plant death. The toxic ions cause ionic and osmotic stress at the cellular level in higher plants, especially in susceptible germplasm [10,11]. Salinity reduces plant growth through osmotic effects and reduces the water uptake, thereby causing a reduction in growth.
There are many effective ways for improving salt-affected land, such as leaching, chemical remediation and phytoremediation [8,12]. The remediation of salt-affected soils using chemical agents, including gypsum (CaSO4∙2H2O), pyrite (FeS2), calcite (CaCO3), calcium chloride (CaCl2.2H2O), and organic matter (farmyard manure, green manure, organic amendment and municipal solid waste), was successful in many cases and has been implemented worldwide, being effective and simple [12-15]. Gypsum and pyrite are the most effective reclamation agents for sodic soils, but they are expensive and beyond the reach of poor farmers in rainfed lowland areas. But the physical, chemical and biological properties of soils in salt-affected areas can also be improved by the application of organic matter, leading to enhanced plant growth and development [16,17]. Pressmud, a sugar industry by-product, is readily available in eastern Uttar Pradesh (U.P.) and less expensive compared to gypsum. Biocompost is prepared by composting pressmud received from cane juice filtration and spent wash received from distilleries through microbial aerobic decomposition. It contains nutrients like N, P, K, Zn and big amounts of organic carbon. Calcium replaces Na+ from the cation exchange complex, and about 2% - 3% sulphur converts into sulphuric acid and lowers soil pH. In addition, it contains bioagents like Trichoderma and Azatobacter which protect plants from several fungal pathogens, enhance growth and development through robust root formation, and enhance soil N availability through atmospheric N2 fixation (Table 1). Therefore, use of biocompost can improve the fertility status and chemical properties of sodic soils as well as increase crop yields.
Recently released rice varieties in India, including CSR 36, CSR 43, Narendra usar 3, and NDR 359, have shown great promise for cultivation in sodic/saline soils of Uttar Pradesh and Bihar. In addition, IRRI made considerable progress in developing a Marker Assisted Backcrossing (MABC) system for the major QTL Saltol, associated with salinity tolerance in rice. Through MABC, this locus is now introgressed into three popular varieties (BR11, BRRI dhan 28, and IR64). Trials conducted under field conditions showed that introgression of this QTL significantly improved the salt tolerance of these varieties, and seeds of these three varieties were now ready for testing in farmers’ fields. The availability of these salt-tolerant varieties provides a great opportunity for increasing and stabilizing productivity in salt-affected areas. Particularly when combined with best management practices specific for salt-affected areas, salt tolerant rice varieties could become a great opportunity for improving productivity and soil quality of saline and sodic soils. Considering this background, we conducted experiments to evaluate the benefits of combining biocompost and salt tolerant varieties together to harness the sodic soil potential.
2. Materials and Methods
The field experiments were conducted during the kharif (wet) season of 2011 and 2012 at the main experiment station of Narendera Deva University of Agriculture and Technology, Kumarganj, Faizabad, India. The experimental site lies between latitude of 26˚47" North and longitude of 82˚12" East, on an elevation of about 113 meters above mean sea level in the Gangetic alluvium of Eastern Uttar Pradesh. The Faizabad district has a semiarid climate, receiving a mean annual rainfall of 1100 mm, and about 80% of the total precipitation occurs during the monsoon season (July to end of September) with few
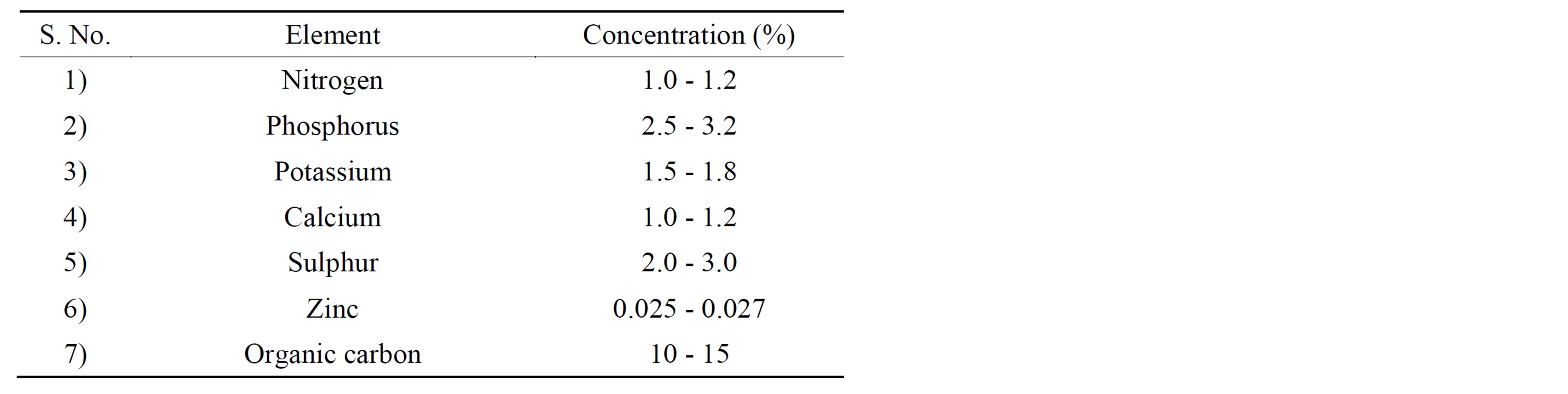
Table 1. Average nutrient and carbon concentration in the applied biocompost.
showers in winter. The soil of the experimental field had a silty clay texture (24% sand, 55% silt, 21% clay), pH 9.3, EC 2.8 dS m−1 and 210, 22.5 and 231.4 kg of available N, P and K ha−1, respectively. Soil organic C, N, P, and K were analyzed by using standard methods [18-21]. The experiment was laid out in a randomized complete block design (RCBD) with three replications, and had four biocompost treatments: T1 Control, recommended doses of N-P2O5-K2O at 120-40-40 kg ha−1 through inorganic fertilizers); T2 Biocompost at 2 t ha−1 + recommended doses of N-P2O5-K2O at 120-40-40 kg ha−1 through inorganic fertilizers; T3 Biocompost at 4 t ha−1 + recommended doses of N-P2O5-K2O at 120-40-40 kg ha−1 through inorganic fertilizers; T4 Biocompost at 6 t ha−1 + recommended doses of N-P2O5-K2O at 120-40-40 kg ha−1 through inorganic fertilizers. The biocompost was brought from the local sugar industry situated in eastern U.P.
Elemental concentrations of biocompost for selected nutrient elements and carbon are given in Table 1. Each treatment was tested with two NDUAT-developed salt tolerant rice varieties, Narendra usar 3 and NDR 359. Narendra usar 3 was released in 2001 with an attainable yield of 2.5 - 3.0 t ha−1 in sodic soils, whereas NDR 359 was released in 1999 having an attainable yield of 3.0 - 3.5 t ha−1 under sodic soil conditions. Both varieties can perform well up to soil pH values of 9.3 - 10.1 with the use of soil amendments like biocompost (pressmud) and gypsum.
In both experimental seasons, biocompost was mixed in the soil 15 days before transplanting and 5 cm water was continuously ponded in each plot for a week. Thirty days old seedlings were transplanted at 15 × 20 cm spacing using 3 seedlings per hill. Full phosphorous and potash and one third of the nitrogen were applied basal one day before transplanting. The remaining two thirds of nitrogen were applied in two equal splits, one at maximum tillering and the other at panicle initiation. Standard recommended cultural practices and plant protection measures were adopted to grow the crop. Observations on various growth parameters, phenology and data on yield and yield components i.e. plant height (cm), biomass per plant (g), EBT, fertile grains panicle−1, sterile grains per panicle−1, 100 seed weight (g) and average yield (t ha−1) were recorded at maturity. Plant height, biomass per plant and yield attributes were determined by randomly sampling 10 hills from each plot. Panicles were hand-threshed and the fertile and sterile grains were separated by submerging threshed grains in 10% saline water. The samples were oven dried at 70˚C to get constant weight. Ear bearing tiller, 100 grain weight, were then computed. Grain yield was determined on a 10 m2 area marked in the middle of each plot. Grains were harvested, dried, and weighed. Soil samples were taken from the plots before start of the experiment and after harvesting of the experiment. The samples were dried and sieved through a 2 mm sieve. Subsamples were used to determine the soil pH and EC with a 1:5 soil: water suspension. All data were analyzed using standard statistical procedures [22].
3. Results and Discussion
Soil samples taken just after harvest showed that biocompost applied at 6 t ha−1 (T4) recorded the highest values of plant available N (252 and 260 kg ha−1), P (44.6 and 47.2 kg ha−1) and K (265 and 266 kg ha−1) in the year 2011 and 2012, respectively (Table 2). Significant increases of plant available N, P and K were also achieved with lower biocompost rates (T2 and T3). Biocompost is organic manure, containing nutrients which are released into the soil after decomposition, increasing the plant available N, P and K. The total increase in available N is similar to the total amount of N applied with the biocompost (20 to 24 kg N in 2 t biocompost), but considerable N was also applied with inorganic fertilizer. Assuming that at least some of the biocompost was decomposed during the season, the soil pool of available N at the end of the season probably contained N from the organic and inorganic fertilizer. The observations also indicate that more N was applied than needed by the crop. Unclear remained if a part of the excessive N entered unavailable soil N pools, and if or how much N had been lost through percolation or gaseous losses. In the case of P, much more P had been applied then found back in the available P pool (50 to 64 kg N in 2 t biocompost). Losses of P are usually small and most of the excess P entered most likely unavailable soil P pools. The availability of P is mainly controlled by soil pH, clay content, calcareousness and organic matter percentage of the soil. Total amount of K applied with the biocompost was between 30 to 36 kg K per 2 t biocompost, and only a small part of that is found back in the available soil K pool at the end of the season (Table 2). As in the case of N, part of the K may have entered unavailable soil K pools and a part might have been lost through percolation into the subsoil.
The biocompost application also had beneficial effects on soil chemical properties like soil pH, electrical conductivity, and organic carbon content (Table 3). Maximum reduction of soil pH was observed in T4 (6 t ha−1 of biocompost), but the trend of falling pH was observed in all biocompost treatments and continued across both seasons. Since soil pH is a soil characteristic indicating an overall picture of the medium for plant growth, including nutrient availability, fate of added nutrients, and sodicity status, this change is very important. Production of inorganic and organic acids (amino acid and humic acid) during mineralization of organic materials by heterotrophs

Table 2. Effect of different doses of biocompost on plant available N, P, and K in the top soil (15 cm) at the end of the season.

Table 3. Effect of different doses of biocompost on soil pH, EC and Organic carbon.
and nitrification by autotrophs would have caused this decrease in soil pH. This effect of organic materials added to sodic soils has been repeatedly described [23]. In parallel, electrical conductivity decreased with the application of biocompost from 2.8 dS m−1 to 2.7 dS m−1 after harvesting of the crop during 2011, but there was no further decrease in the following season. The underlying process is that the decomposition of organic materials releases acids or acid forming compounds that react with the soluble salts present in the soil and either convert them into insoluble salts or decrease their solubility. In addition, organic matter has a very high exchange capacity and will have removed further cations and anions from the soil solution.
Application of biocompost also increased the very low level of soil organic carbon (only 0.4% in the control soil; Table 3). This effect was of course strongest in T4 but also occurred if only 2 t biocompost per ha were applied. Organic matter is an important source of nutrients and microbial activity in the soil, improves soil structure, water holding capacity, infiltration rate, aeration and porosity of the soil. Most likely, the high Ca2+ content of the biocompost would also have replaced Na+ cations from the exchange complex but we did not determine the sodium absorption ratio. Overall, these results confirm the observations [24], who reported that the increase in organic carbon and decrease in soil pH and EC were stronger in organic manured treatments as compared to fertilizers alone. Thus, the biocompost treatments had an overall beneficial effect on soil physical and chemical properties, and increased nutrient availability. The buildup in N, P and K supplies has a residual effect and improves the soils lastingly [25].
The response of both rice varieties to biocompost application was clearly visible during both years although plant height did not differ significantly between treatments. But all treatments significantly influence the yield attributes and yield over the control treatment in both seasons. The beneficial effect of higher doses of biocompost was more pronounced but the effects occurred also at lower doses. The effect of biocompost on plant biomass was found significant at higher doses but the interaction of variety with biocompost was non-significant. In 2012, lower plant height and higher biomass of NDR 359 were recorded as compared with 2011, possibly due to the residual effect of the biocompost (Table 4). In comparison with the control, maximum increase in ear bearing tillers (EBT) (35 and 37% of Narendra usar 3, and 60 and 21% of NDR 359 in 2011 and 2012, respectively) was recorded with treatment T4 (biocompost at 6 t ha−1). Treatment T4 also increased fertile grains per panicle (32 and 9% of Narendra usar 3, and 28 and 20% of NDR 359 in 2011 and 2012, respectively), reduced sterile grains per panicle (37 and 26% of Narendra usar 3, and 36 and 54% and 10 and 7% of NDR 359 in 2011 and 2012, respectively) (Table 5).
Application of biocompost significantly increased grain yield as a consequence of these effects on yield components, and increasingly so with higher biocompost rates (Figure 1). A higher relative yield increase was recorded in Narendra usar 3, but the actual yield and yield increase was higher in NDR 359 in both experimental years and in all treatments. It is logical that a higher dose of biocompost application resulted in a higher N, P and K supply, but the additional biocompost effects on soil pH, conductivity, and soil organic carbon will have further increase nutrient availability to the crop. Other effects could include an increased mineralization of soil organic matter due to the low C:N ratio of the biocompost, and possibly plant growth stimulants and beneficial microflora contained in the biocompost [26]. Several other workers also reported the positive cumulative effect of organic manures and the direct effect of fertilizers on rice yields [27-29].
It is clear from the economic analysis (Table 6) which only considered additional costs and gains related to the treatment, that use of biocompost provided additional income as compared with the control (without biocompost application) in case of both varieties. The yield improvement with biocompost ranged between15% to 25% in the salt tolerant Narendra user 3 and between 7% to 24% in NDR 359. Higher doses of biocompost (T4, 6 t ha−1)
produced higher yields than the lower doses (4 t ha−1 in T3 and 2 t ha−1 in T2), but the combination of costs and gains resulted in highest treatment gains from T3 combined with Narendra user 3. Thus, biocompost application led to a net profit increase of up to Rs. 3900 ha−1 per rice crop from a degraded sodic soil.
4. Conclusions
The results of the field experiments indicated that the application of biocompost at 2 to 6 t ha−1 in sodic soils 15 days before transplanting enhanced available nutrient contents, thus increasing the overall soil fertility status. The combination of improved soil conditions and applied nutrients caused improved values for yield attributes like ear bearing tillers, sterile grains per panicle, 100 grain weight, and finally grain yield.
Assuming an average yield advantage of 20% - 30% over the existing rice yield of 2.9 - 3.3 t ha−1, it can contribute to food security and improve the livelihood of rice

Table 4. Plant height (cm) and biomass per plant (g) of two rice varieties in response to different doses of biocompost application in sodic soil.
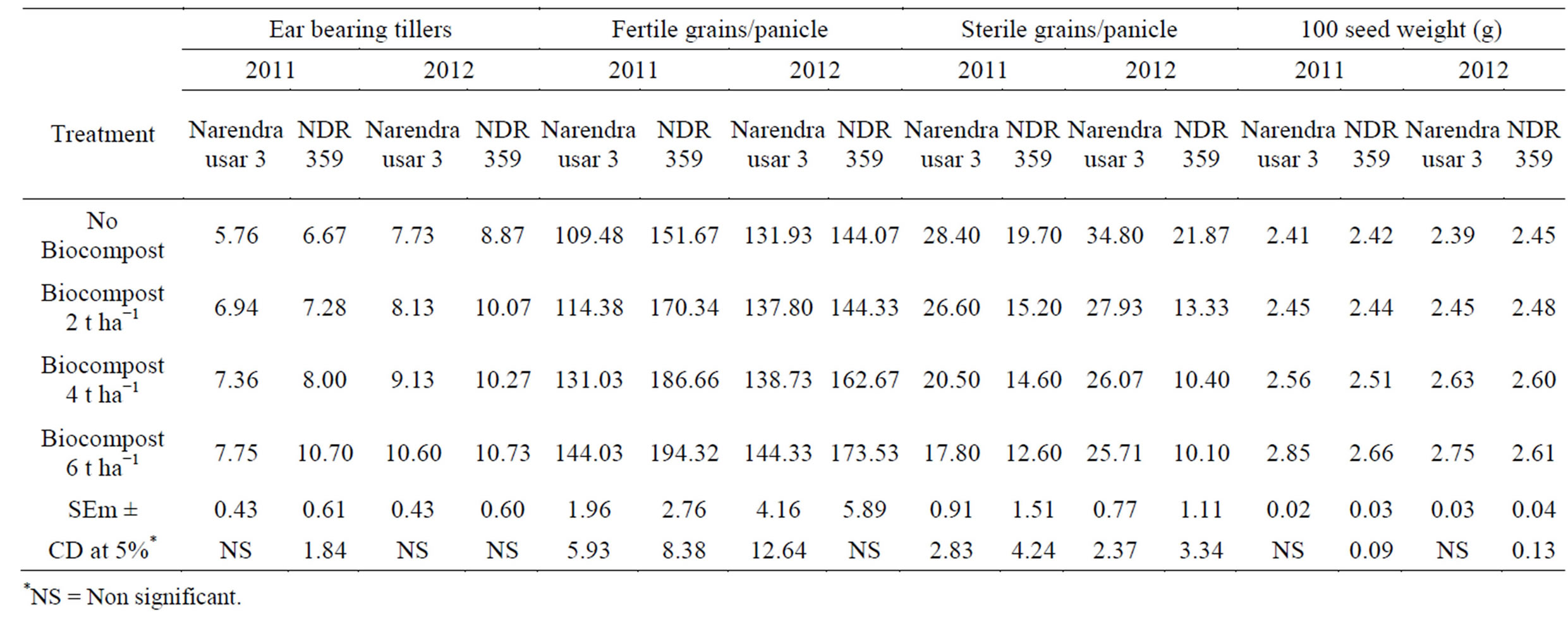
Table 5. Yield components of the two rice varieties in response to different rates of biocompost application in sodic soil.
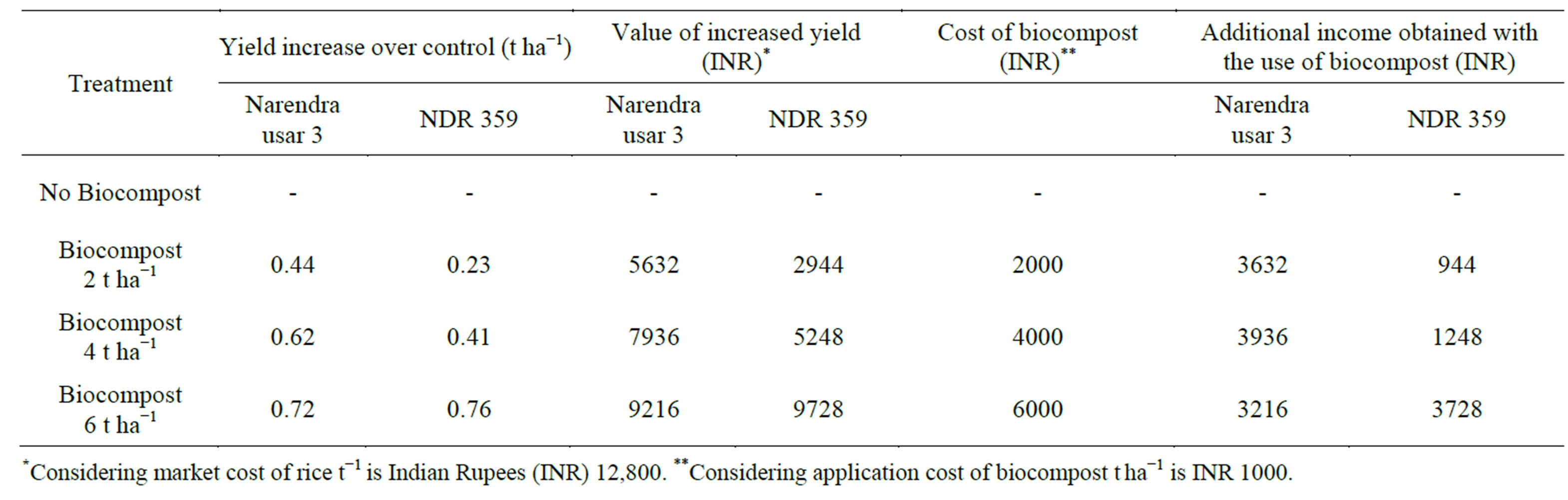
Table 6. Economic analysis dependent on treatments and varieties used.
(a)
(b)
Figure 1. Effect of different doses of biocompost on grain yield of rice varieties in sodic soil conditions. (T1—No biocompost, T2—Biocompost at 2 t ha−1, T3—Biocompost at 4 t ha−1 and T4—Biocompost at 6 t ha−1).
farmers in rainfed lowlands. By applying nutrient rich biocompost in rice, the farmer may also be benefited in the succeeding crop, getting an additional benefit not evaluated in our study. Thus, application of biocompost can be effectively introduced as an eco-friendly component in the integrated nutrient management system to enhance the soil fertility status and achieve sustainability in crop production in sodic soils of rainfed lowlands.
Treatment dependent yield increases after biocompost applications were highest in Narendra usar 3, but the absolute yield of NDR 359 was still higher in all cases. However, the shorter duration of Narendra usar 3 can be attractive to farmers in cases where late planting and late harvesting cannot be avoided, reducing the attainable yield of the following wheat crop, or where water scarcity at the end of the season is common.
Acknowledgements
The authors are highly grateful to the Narendra Deva University of Agriculture and Technology for providing technical support for experimental work, including soil analysis, and the European Commission and International Fund for Agricultural Development (EC-IFAD) for providing the financial support as part of the project “Improved rice crop management for raising productivity in the submergence prone and salt affected rainfed lowlands in South Asia”.
NOTES