The Impact of Microscopic Fungi on the Morphological and Structural Properties of Carbon Steel ()
1. Introduction
The investigation of the steel surface destruction is important due to iron importance in the industry and environment [1] -[6] . Atmospheric deterioration of steel is a result of interaction between the existing surface with its abiotic destruction products and microscopic fungi with their metabolites. The development of a biofilm is facilitated by the production of extracellular polymeric substances such as proteins, polysaccharides, nucleic acids and lipids. All microorganisms require water, carbon and many trace elements for growth. Possible biomineralization in heterogeneous aqueous systems results from a complex association between metal surface, fungi, extracellular biomacromolecules and neoformed precipitates. The sequence of abiotic and biotic reactions produces a pathway of electron flow from the metal to the universal electron acceptor—oxygen. The chemical and/or biodeterioration are the new sources of modification of fungi treated surface, that can change type and concentration of ions, pH values, oxidation-reduction potential on the interface and can induce iron ions redox and transformation reactions [7] -[14] .
The oxides formed under wet influence on steel surfaces play a major role in fungal physiology. The exceptional physicochemical particularities of iron oxides prove a good biocompatibility and sometimes impressive antimicrobial properties [2] [15] -[20] .
The microscopic fungi due to their different adaptation capabilities and vital capacity affect metals surface differently: some of them pronouncedly intensify biodeterioration processes on the iron surface, others diminish the intensity of biodeterioration process and sometimes even suspend them [5] [10] [21] .
It is known, that P. palitans produces numerous organic acids and is adaptable to various substrata, A. phaeospermum produced a wide spectrum of organic products [2] [22] -[24] . It was observed, that Penicillium palitans strain 6 (P. palitans 6) and Arthrinium phaeospermum strain 10 (A. phaeospermum 10) grew most quickly onto steel surfaces under natural conditions. The purpose of this study was to determine the influence of two strains of micromycetes: P. palitans 6 and A. phaeospermum 10 on the morphological and structural changes of steel surface under modeled conditions. This investigation is interesting not only from the point of view of materials science, but also for biocompatibility and environment.
2. Experimental Part
The following strains of two species of micromycetes from our culture collection were used for studies [2] [19] [25] : P. palitans 6: Penicillium palitans Westling, strain 6, Section Viridicata, Series Camemberti [22] [23] and A. phaeospermum 10: Arthrinium phaeospermum (Corda) M. B. Ellis strain 10 [2] [24] .
To determine the capacity of micromycetes strains to adapt to metals, experiments were performed under laboratory conditions using a sterile malt agar extract supplied with chloramphenicol (50 mg∙1−1). Plates (10 × 30 × 3 mm) cut of low carbon steel (steel) (C 0.05% - 0.12%, Cu 0.003% - 0.10%, P < 0.07%) were used for investigations. Metal plates were placed in Petri dishes filled with a malt agar extract medium; medium was sown up with the fungi of two above mentioned strands, contaminated with P. palitans 6 (Fe6) and A. phaeospermum 10 (Fe10). After these Petri dishes with samples were cultivated in a thermostat of 26˚C ± 2˚C, the extent of fungal growth was assessed by the naked eye and a light microscope MVS-10 (Russia). The reference samples K1 were exposed to air and the reference samples K2 were exposed to medium of malt extract without fungi. The experimental dates are presented after 30 days of exposure.
The surface morphology of samples was examined using scanning electron microscopy (SEM) with a scanning electron microscope EVO 50 EP (Carl Zeis SMTAG, Germany). A strong air blast and cleaning with distilled water as well as ethyl alcohol were used.
A Nicolet model 5700 Fourier transformation infrared (FTIR) spectrometer in conjunction with a 10 Spec (10 Degree Specular Reflectance Accessory) was used for spectroscopy investigations. FTIR spectra were recorded with a 4 cm−1 resolution and 64 scans. Spectral manipulation included baseline correction, removal of carbon dioxide absorption bands and subtraction of water vapor interferences. The samples of damaged steel plates were cleaned with dry filter paper. The fouling products formed on steel surface were collected and their FTIR spectra were recorded.
The phase composition of steel surface was studied by the X-ray diffraction (XRD) method using a diffractometer DRON-3.0 Cu Kα (λ = 0.154 nm, U = 30 kV, I = 20 mA) without any additional preparations.
The chemical composition of steel plates was determined using a Bruker S4 Explorer X-ray fluorescence (XFS) spectrometer.
3. Results and Discussion
The growth of fungi on steel plates presented on Figure 1 suggests that the studied fungi contaminated steel plates differently. The mycelium of P. palitns 6 (Figure 1, Fe6) fungi changed at the interface, in places grew on the edges of steel plates and fungi conidia were strongly attached. The highest intensity of growth covering <75% of the tested surface under the action of A. phaeospermum 10 (Figure 1, Fe10) was observed. The mycelium of A. phaeospermum 10 fungi covered the surface, at the beginning the color changed, darkened especially at the substrate. When the steel plates were exposed in nutrient medium without fungi (Figure 1, K2) zones of metabolic products were formed around metal plates. For comparison, exposed to air plates K1 remain without changes.
The data of morphological studies performed by using the SEM method are given below (Figure 2). The SEM images demonstrate, that the metabolic action of strains P. palitans 6 and A. phaeospermum 10 of fungi on the steel surfaces is not the same. The surface of steel contaminated with P. palitans 6 fungus (Figure 2, Fe 6) was cracked and particles of destruction products were visible on the surface. After steel plates exposure to A. phaeospermum 10 (Figure 2, Fe10) their surface was heavily cracked, with cracks 3 - 4 μm in width. The bunches were visible on the mycelium of fungi.
The FTIR spectra of organic substances for reference samples exposed to malt agar (Figure 3, curve K2) and for samples contaminated with fungi P. palitans 6 (Figure 3, curve Fe6) and A. phaeospermum 10 (Figure 3, curve Fe10) over the experimental period were without great difference. Bands characteristic of the functional groups usually found in biofilm were seen in the FTIR spectra obtained. They are as follows: carboxilate group, (COO−, 1600 cm−1), the broad band (C–O–C) with peaks of the saccharides component action in the region 1200 - 900 cm−1 and the strong band in the region 1290 cm−1 due to C–O stretching vibrations for carbohydrate are observed [26] [27] .
The FTIR spectra waves in the range less than 700 cm−1 characteristic of metals oxides [3] [14] [16] [28] have appreciable different width, possibly because of incorporation of bioinspired constituents.
The interface composition was further investigated by XFS. According to XFS analysis sample K2 exposed to
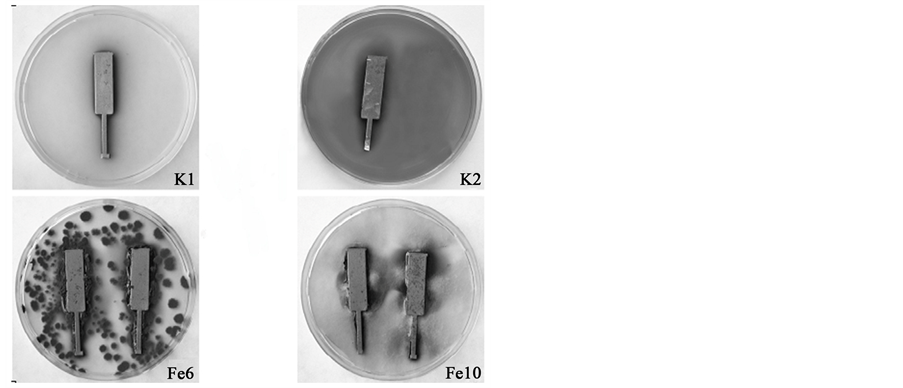
Figure 1. Fungal colonies: K1 (reference), not exposed plate; K2 (reference), exposed without fungi; Fe6, exposed to P. palitans 6; Fe10, exposed to A. phaeospermum 10; 30 days of exposure at 26˚C ± 2˚C.

Figure 2. A SEM image for the biofilm developed on the steel surface: K2 (reference), exposed without fungi plate; Fe6, exposed to P. palitans 6; Fe10, exposed to A. phaeospermum 10; 30 days of exposure at 26˚C ± 2˚C.

Figure 3. FTIR spectra of steel samples: K2 (reference), exposed without fungi plate; Fe6, exposed to P. palitans 6; Fe10, exposed to A. phaeospermum 10; 30 days of exposure at 26˚C ± 2˚C.
the nutrient medium contained impurities of chlorine, potassium, cooper and phosphorus in contrast to their absence in sample K1 and increases of quantity of other elements (manganese, sulphur, natrium, calcium, aluminum) presented in sample K1 precursor (Table 1). The consumption of almost all detected microelements by growth of P. palitans 6 is more significant than that by A. phaeospermum 10 growth (Table 2).
Moreover, the data of XFS analysis have shown a difference in accumulation of newly formed elements manganese for both samples Fe6 (0.01 wt%) and Fe10 (0.03 wt%) and magnesium (0.01 wt%), which was caused only by the growth of P. palitans 6. Worthy of remark, that oxidation-reduction reactions of manganese and magnesium ions are largely influenced by biological activity and they are taking an active role in the metabolism of cells [29] . The elemental analysis has shown, that chlorine is present in minor quantity in samples affected by both P. palitans 6 (0.04 wt% chlorine) and A. phaeospermum 10 (0.07 wt% chlorine), meanwhile the reference sample K2 contained of 0.11 wt% of chlorine. It is important, because chlorine accelerates the corrosion of steel and may increase the porosity of oxide layer [30] . The difference in consumption of chlorine by P. palitans 6 (0.07 wt%) and A. phaeospermum 10 (0.04 wt%) are monitored. More active physiological properties of P. palitans 6 fungus in comparison to those of A. phaeospermum 10 promoted major influence on the activity levels of biofilm organisms. The inherent diversity of biogenic material may offer opportunities for practical applications.
The constituent of destruction products was determined in accordance with XRD spectroscopic results (Figure 4). As shown, (Figure 4, pattern K1), steel plate K1 exposed to air during the experiment time indicated no obvious oxidation. A XRD pattern exhibits peaks at 2Θ = 44.67˚ (110), 2Θ = 65.02˚ (200) and 2Θ = 82.33˚ (211) corresponding to the cubic crystalline metallic iron structure (JCPDS: 06-0696) [31] .
When the samples exposed to the malt agar extract medium (Figure 4, pattern K2) the supplementary scattering band in the 2Θ range 35˚ - 42˚ as a result of bare steel biotic oxidation is observed. We speculate, that only some small amounts possibly the composition of various known iron oxides magnetite, maghemite, ferrihydrite, hematite (JCPDS: 19-0629, JCPDS: 025-1402, JCPDS: 046-1315, JCPDS: 33-066) [3] [28] [32] [33] and biogenic manganese compounds are detected [34] . By the influence of fungi (Figure 4, pattern Fe6, Fe10) the bands of damage in the XRD patterns become broadening. In the case Fe10 diffraction peak at 2Θ = 40.1˚ becomes stronger, thus can be attributed to the influence of the fungal deposited manganese in the manganese oxide hydroxide (JCPDS: 88-0649) and Na4Mn14O27 9H2O (JCPDS: 23-1046) containing crystalline phases [34] [35] .
4. Conclusion
The morphological and structural studies demonstrate the interaction between the steel surfaces with strains P. palitans 6 and A. phaeospermum 10 of fungi in the nutrient medium. The biofilms were extensively characterized

Figure 4. XRD pattern of steel samples: K1 (reference), not exposed plate; K2 (reference), exposed without fungi; Fe6, exposed to P. palitans 6; Fe10, exposed to A. phaeospermum 10; 30 days of exposure at 26˚C ± 2˚C.
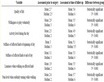
Table 1. Microelements quantity in the steel surface layer, wt%.
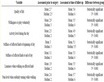
Table 2. Consumption of microelements in the steel surface layer, wt%.
and their impact on the morphological and structural properties of carbon steel was investigated. XFS analysis has shown the difference in consumption and production of microelements, which was caused by the growth of P. palitans 6 and A. phaeospermum 10. The surface XRD data indicate the biotic oxidation and formation of the mixed oxides on the biomodified steel surface.
NOTES
*Corresponding author.