Coordination Behavior and Biological Activity of Some Transition Metal Complexes with 2-Acetyl and 2-Formyl-3-Amino-1,4-Naphthoquinone Ligands ()
1. Introduction
Chemical synthesis is a hot topic because of a global crisis of drug resistance, in pathogens of both clinical and agriculture importance. Many of these pathogens are resistant to multiple classes of antibiotics and is increasingly common for them to be resistant to practically all available drugs, leaving few alternatives for the treatment of infections, especially in immunocompromised patients [1] [2]. Despite addition of new classes of antimicrobials, the number of currently available drugs for infections treatment remains limited. Therefore, there is a continuing need to develop new, simpler, more effective and less toxic antimicrobials agents; so naphthoquinones and derivatives are a group of great importance that has attracted interest of the scientific community. Naphthoquinones are natural aromatic compounds that can be found in several plant families, as well as isolated of fungi, algae and bacteria. Traditionally used for their dyeing properties, however, recently a variety of biological activities of these compounds has been reported [3] [4]. In most cases, these pharmacological activities are related to redox and acid-base properties, which can be modulated synthetically by modifying the substituents attached to the 1,4-naphthoquinone ring, in order to enhance their therapeutic actions. At the present time, the synthetic methods should be designed according the principles of green chemistry to promote process more sustainable with the environmental and human safe. Because of this, in this chapter is described the chemistry and green synthesis of natural and synthetic naphthoquinones as potential antibacterial, antifungal, anti-parasitic and antiviral agents, as well as its mechanism of action. Contributing in the area of synthesis and screening of novel chemical compounds for antimicrobial action. Naphthoquinones are structurally related to naphthalene, are characterized by the presence of two carbonyl groups in the 1,4 position and 1,2 position with lower incidence, which are named as 1,4-naphthoquinones and 1,2-naphthoquinone respectively. Naturally present hydroxyl and methyl groups as substituents, can be found in free form or condensed with oligosaccharides. Naphthoquinones are highly reactive organic compounds, traditionally used as natural or synthetic dyes whose colors range from yellow to red [5] [6]. The effect of metal complexation on the antimicrobial activity of 1,4-naphthoquinones was investigated. Nickel-, chromium-, iron-, copper-, and cobalt-containing metal chelates of 5-amino-8-hydroxy-1,4-naphtoquinone (2) and its acyl-derivatives (3 - 8) were synthesized and characterized, and their antimicrobial activity was evaluated. Data from infrared spectroscopy indicate that naphthoquinones coordinate through oxygen and nitrogen atoms for (2), and through oxygen atoms when ligands were acyl derivatives (3 - 8). Susceptibility tests for antimicrobial activity showed that 2 and its acyl derivatives were effective on inhibiting the growth of pathogenic bacteria such as Staphylococcus aureus, Streptococcus uberis and Bacillus cereus, but not Gram-negative bacteria. The metal complexation often caused decrease of biological activity. Nickel complex of (2) was the most effective against Gram-positive bacteria, showing MIC values ranging from 375 to 1400 mg/ml. Metal chelates may be useful tools for the understanding of the antimicrobial mechanism of 1,4-naphthoquinones on these bacteria [5].
2. Experimental
2.1. Materials and Reagents
Analytical grade chemical reagents were used. All chemicals used in this study are of the highest purity from commercial suppliers such as Merck; BDH and Aldrich they include 1,4-Naphthoquinone, Acetamide and Formamide, CoCl2∙6H2O, NiCl2∙6H2O and CuCl2∙2H2O. The organic solvents such as absolute ethanol and methanol, DMF and DMSO are purchased from Alpha Easer.
2.2. Instrumentation
Melting point apparatus (Gallen Kamp, Germany) was used to investigate the melting points. Elemental microanalysis of the separated solid chelates for C, H and N were performed in the Micro analytical Center, Cairo University, using CHNS-932(LECO) Vario Elemental analyzers. Infrared spectra were recorded on Perkin-Elmer FT-IR type 1650 spectrophotometer in wave number region 4000 - 40 cm−1. The spectra were recorded as KBr pellets. The 1H NMR spectra were recorded using 300 MHz Varian-Oxford Mercury. The deuterated solvent used was dimethylsulphoxide (DMSO-d6) and the spectra extended from 0 - 15 ppm. DTA-TG apparatus. The electron impact (EI) mass spectra (MS) at 70 eV of the tested compounds has been done using MS-5988 GS-MS Hewlett-Packard instrument. TGA was carried out in dynamic nitrogen atmosphere (10 mL∙min−1) with a heating rate of 10˚C∙min−1 using DTG-50 H Shimadzu simultaneous. The molar conductance of solid chelates in DMF was measured using WPA CM35 Conductivity meter fitted with platinized platinum electrodes. The antibacterial and antifungal activities were evaluated at the Microbiological laboratory, Micro analytical center, Cairo University, Egypt.
2.3. Methods
2.3.1. Synthesis of Free Ligands
1,4 Naphthoquinone (2 g, 0.0126 mol) mixed with an equivalent amount (0.74 g, and 0.57 g, 0.0126 mol) of acetamide and formamide respectively, then the mixture was added to an aqueous solution of sodium hydroxide and left for two hours on water bath to fused. The crude product was recrystallized from ethanol and dried under vacuum over P2O5. The yield was 90%. The melting point was measured and listed in Table 1. The procedure cited in respective reference [7] [8].
2.3.2. Preparation of Metal Complexes
The metal complexes were prepared by dissolving (1075 g and 1.005 g, 0.005 mol) of ligand (L1 - L2) respectively in hot ethanol (50 ml) and added drop wisely with stirring to a stoichiometric amount of 1:1 (M:L) molar ratio to (1.189 g, 1.888 g, and 0.852 g, 0.005 mol) of CoCl2.6H2O, NiCl2∙6H2O and CuCl2∙2H2O respectively. The reaction mixture was refluxed for 40 min and left overnight. The isolated solid complexes were filtered off, washed with distilled water until the solution became colorless and washed with 10 ml hot ethanol-water mixture (1:1) to remove any traces of the unreacted materials. The solid complexes were dried at 70˚C for several hours kept in desiccator containing dry P2O5. Analytical data were listed in Table 1.
![]()
Table 1. Analytical and physical properties of 1,4-Naphthoquinone complexes.
effμ: effective magnetic moment.
2.4. Biological Activity
Modified Kirby-Bauer disc diffusion method [9], has been used to determine the antimicrobial activity of the tested samples [10]. Examined 100 μl of the tested bacteria or fungi and found it was developed in 10 ml of fresh media until they reached a count of approximately 108 cells/ml for bacteria and 105 cells/ml for fungi. 100 μl of microbial suspension was spread onto agar plates corresponding to the broth in which they were maintained. Isolated colonies of each organism that might be playing a pathogenic role should be selected from primary agar plates and tested for susceptibility by disc diffusion method of the many media available, NCCLS recommends Mueller-Hinton agar due to it results in good batch-to-batch reproducibility. Disc diffusion method for filamentous fungi tested by using approved standard method (M38-A) developed. For evaluating the susceptibilities of filamentous fungi to antifungal agent. Disc diffusion method for yeast developed by National Committee for Clinical Laboratory Standards using approved standard method (M44-P). Plates inoculated with filamentous fungi as Asprgillus flavus at 25˚C for 48 hours; Gram (+) bacteria as Staphylococcus aureus; Gram (−) bacteria as Escherichia coli, they were incubated at 35˚C - 37˚C for 24 - 28 hours and yeast as Candida albicans incubated at 30˚C for 24 - 28 hours, then the diameters of the inhibition zones were measured in millimeters with slipping calipers of the National Committee for clinical Laboratory Standards [11], have been used standard discs of tetracycline (antibacterial agent), and amphotericin B (antifungal agent) served as positive controls for antimicrobial activity but filter discs impregnated with 10 μl of solvent (distilled water, chloroform, DMSO) were used as a negative control. The agar used is Mueller-Hinton agar that is rigorously tested for composition and pH. Further the depth of the agar in the plate is a factor to be considered in the disc diffusion method. This method is well documented and standard zones of inhibition have been determined for susceptible and resistant values. Blank paper discs (Schleicher and Schuell, Spain) with a diameter of 8.0 mm were impregnated with 10 μl of tested concentration of the stock solutions. When a filter paper disc impregnated with a tested chemical is placed on agar, the chemical will diffuse from the disc into the agar. This diffusion will place the chemical in the agar only around the disc. The solubility of the chemical and its molecular size will determine the size of the area of chemical infiltration around the disc. If an organism is placed on the agar, it will not grow in the area around the disc if it is susceptible to the chemical. This area of no growth around the disc is known as zone of inhibition or clear zone. For the disc diffusion, the zone diameters were measured [12], found that, agar based methods such test and disc diffusion can be good alternatives because they are simpler and faster than broth-based methods.
3. Results and Disscussion
3.1. Elemental Analysis and Physical Properties
The results of elemental analyses and physical properties of the free ligands and its metal chelates shown in Table 1 are in good agreement with those required by proposed formulae. The isolated solid complexes are stable at room temperature, partly soluble in organic solvents (L1 - L2), but completely soluble in DMF and DMSO. Based on the above mentioned results, it can propose the general structural formulae of the complexes (M:L) ratio 1:1 is represented in Figure 1 and Figure 2.
3.2. Molar Conductivity Measurements
The metal chelates were dissolved in DMF at 25˚C ± 2˚C and the molar conductivities of 5 × 10−4 M of their solutions were measured by recommended procedure [13]. The obtained molar conductance values are listed in Table 1. The molar conductivity value of Co(II), Ni(II) and Cu(II) chelates of free ligands (L1 - L2) are found to be 5.20 up to 7.76 Ω−1∙mol−1∙cm2. The chelates are nonionic in nature and they are considered as non-electrolytes.
3.3. FT-IR Spectroscopy
The main FT-IR bands of 2-acetyl-3-amino-1,4-naphthoquinone (L1) and (L2) and their corresponding metal chelates are presented in Table 2.
The vibration spectra of prepared ligand L1 exhibit a very broad band at 3433 cm−1 and at 3413 cm−1 assigned to the υ (NH2) stretching vibration of (C2-NH2) of the naphthoquinone. The stretching band at 1624 cm−1 and at 1690 cm−1 can be assigned to the ʋ (C=O). The stretching band at 1577 cm−1 and at 1536 cm−1 can be assigned to the υ (C-N). The vibration spectra of the prepared complexes of L1 exhibits a broad bands around 3548, 3542 and 3553 cm−1 due to the υ (OH)
![]()
Figure 1. The proposed structure of the prepared metal complexes of 2-acetyl-3-amino-1,4-naphthoquinon (L1).
![]()
Figure 2. The proposed structure of the prepared metal complexes of 2-formyl-3-amino-1,4-naphthoquinon (L2).
![]()
Table 2. Infrared spectral data of free ligand (L1 - L2) and their metal chelates.
stretching, similarly L2 exhibits a broad bands around 3537, 3543 and 3539 cm−1 due to the υ (OH) stretching. The lower shift of υ (NH2) band in at 3392, 3378 and 3355 cm−1 for L1 and 3400, 3370 and 3362 cm−1 for L2 - Co(II) Ni(II) and Cu(II) complexes support the contribution of N-atom of NH2 group in complex formation. On complexation L1 show lower shift at 1618, 1609 and 1616 cm−1 for Co(II), Ni(II) and Cu(II) ions respectively due to stretching vibration of ʋ (C=O), which supports the involvement of oxygen atom of (C=O) group in chelation. In a similar fashion on complexation L2 show lower shift at 1674, 1680 and 1673 cm−1 for Co(II), Ni(II) and Cu(II) ions respectively due to stretching vibration of υ (C=O), which supports the involvement of oxygen atom of (C=O) group in chelation. Also on complexation of L1 and L2 with Co(II), Ni(II) and Cu(II) ions respectively, a red shift has been observed in υ (C-N) stretching vibration at 1586, 1584 and 1588 cm−1 and at 1587, 1583 and 1591 cm−1 respectively which may be due to increase of bond order of carbon to the nitrogen link following the coordination of the imine nitrogen atom to metal ions. The stretching bands of the coordinated water molecules υ (H2O) was observed at 808, 868 and 896 cm−1 for Co(II), Ni(II) and Cu(II) - L1 complexes and at 987, 972 and 924 cm−1 for Co(II), Ni(II) and Cu(II) - L2 complexes. The new bands observed at 582, 578 and 586 cm−1 of L1 complexes assigned to υ (M-O) and at 472, 469 and 467 cm−1 assigned to υ (M-N). Also new band are observed at 572, 577 and 583 cm−1 in all complexes of L2 under study assigned to υ (M-O) and at 469, 466 and 468 cm−1 assigned to υ (M-N).
3.4. 1H-NMR Measurements
3.4.1. 1H-NMR of 2-Acetyl-3-Amino-1,4-Naphthoqunone (L1) and Its Cobalt Complex
1H-NMR spectrum 300 MHz of 2-acetyl-3-amino-1,4-naphthoqunone (L1) shows several signals and the resulted data are tabulated in Table 3.
The 1H
NMR
spectrum (L1) shows a singlet signal at δ 2.5 ppm of relative intensity (s, 3H) may be attributed to CH3 protons, a multiplet at δ 7.2 - 7.5 ppm of relative intensity 4H, may be assigned to four protons (m, 4H) in quinone, Harm. The multiplet signal observed at δ 8 ppm may be assigned to two protons of amino group (m, 2H, NH2) [14].
On comparing the investigated 1H-NMR signals of the cobalt chelate [CoC12H9NO3Cl2∙(H2O)2]∙4H2O with those of the L1 ligand protons signals, multiplets and the chemical shifts. It has been found that methyl protons of the free ligand are slightly shifted to δ 2.49 ppm. Also the NH2 protons of the free ligands are slightly shifted to δ 7.7 ppm this suggests that the metal ion coordination takes place through the nitrogen atom of NH2 group. The proton signal observed at δ 3.52 ppm, which may be assigned to the presence of water molecules is in agreement with the suggested formulae of metal chelates.
3.4.2. 1H-NMR of 2-Formayl-3-Amino-1,4-Naphthoqunone (L2) and Its Nickel Complex
1H-NMR spectrum 300 MHz of 2-formayl-3-amino-1,4-naphthoqunone (L2) shows several signals and the resulted data are tabulated in Table 3.
The 1H
NMR
spectrum (L2) shows a singlet signal at δ 9.6 ppm of relative intensity (s, 1H) may be attributed to CHO protons, a multiplet at δ 7.13 - 7.5 ppm of relative intensity 4H, which may be assigned of four protons (m, 4 H) in quinone, Harm.
![]()
Table 3. 1H-NMR data for free ligands (L1 - L2) and their metal chelates.
The multiplet signal observed at δ 8.4 ppm may be assigned to two protons of amino group (m, 2H, NH2) [15].
The comparison of the protons signals, multiplets and the chemical shifts of L2 ligand with its corresponding nickel chelate [NiC11H7NO3Cl2∙(H2O)2]∙4H2O is investigated. It has been found that the aldehyde CHO protons of the free ligand are slightly shifted to δ 9.4 ppm. Also the NH2 protons of the free ligands are slightly shifted to δ 7.8 ppm this suggests that the metal ion coordination takes place through the nitrogen atom of NH2 group. The proton signal is observed at δ 3.18 ppm, which may be assigned to the presence of water molecules is in agreement with the suggested formulae of metal chelates. The 1H-NMR spectrum of 2-formayl-3-amino-1,4-naphthoqunone nickel chelate shows several characteristic signals.
3.5. Mass Spectroscopic Studies
3.5.1. Mass Spectra of 2-Acetyl-3-Amino-1,4-Naphthoqunone (L1) and Its Copper Complex
The electron impact mass spectrum of [CuC12H9NO3Cl2 (H2O)2] Figure 3 shows many fragment ions which consists of two principle pathways as shows in Scheme 1. The signal that appears at m/z = 385.05 (mole mass = 385.68) may be referred to the appearance of main general molecular weight of metal chelate which undergo two pathways of fragmentation. Pathway I stated that; the main metal chelates lose two molecules of water leaving a fragment at m/z = 352.86 (mole mass = 349.65, RI = 36%) followed by the appearance of a signal at m/z = 176.68 (mole mass = 174.64, RI = 4%); which may be refer to the loss of C7H4O (cyclohexa-1,3-dien-5-yne-1-carbaldehyde) and Cl2 followed by the appearance
![]()
Figure 3. Mass Spectrum of [Cu L1Cl2 (H2O)2].
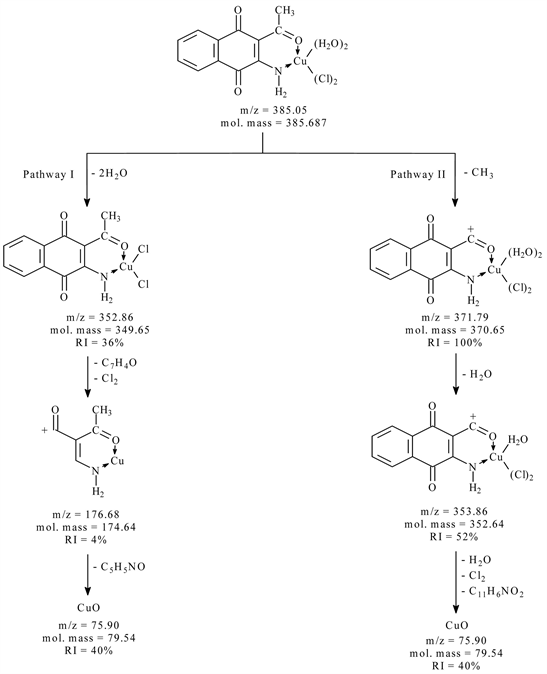
Scheme 1. The mass fragmentation pathways of copper complex with (L1).
of a signal at m/z = 75.90 (mole mass = 79.54, RI = 40%) as rupture of C5H5NO (4-Hydroxypyridine) from the fragment. Pathway II shows a fragment at m/z = 371.79 (mole mass = 370.65, RI = 100%); which may be refer to the loss of CH3, followed by the appearance of a signal at m/z = 353.86 (mole mass = 352.64, RI = 52%); which may be refer to the loss of one molecules of water, Cl2 and C11H6NO2 (furo[2,3-b]quinolin-8-olate) leaving a fragment give a signal at m/z = 75.90 (mole mass = 79.54, RI = 40%).
3.5.2. Mass Spectra of 2-Formyl-3-Amino-1,4-Naphthoqunone (L2) and Its Copper Complex
The electron impact mass spectrum of [CuC11H7NO3Cl2∙(H2O)2] shows many fragment ions which consists of two principle pathways as shows in Scheme 2. The signal that appears at m/z = 371.29 (mole mass = 371.66) may be referred to the appearance of main general molecular weight of metal chelate which undergo two pathways of fragmentation. Pathway I stated that; the main metal chelate
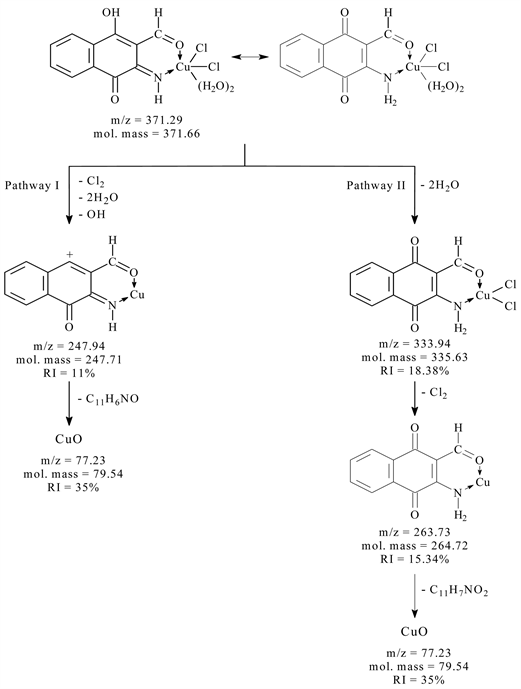
Scheme 2. The mass fragmentation pathways of copper complex with (L2).
lose two molecules of water, hydroxyl ion and Cl2 leaving a fragment at m/z = 247.94 (mole mass = 247.71, RI = 11%) followed by the appearance of a signal at m/z = 77.23 (mole mass = 79.54, RI = 35%); which may be refer to the loss of C11H6NO (furo[2,3-b]quinolin-8-olate). Pathway II shows a fragment at m/z = 333.94 (mole mass = 335.63, RI = 18.38%); which may be refer to the loss of two molecules of water, followed by the appearance of a signal at m/z = 263.73 (mole mass = 264.72, RI = 15.34%); which may be refer to the loss of Cl2, followed by the appearance of a signal at m/z = 77.23 (mole mass = 69.54, RI = 35%) as rupture of C11H7NO (1,4-dioxo-2,3-dihydrona-phthalene-2-carbonitrile) from the fragment.
3.6. Thermogravimetric Analyses (TGA)
The TGA thermal analyses data of the synthesized metal chelates are tabulated in Table 4.
![]()
Table 4. Thermoanalytical analyses data for newly synthesized chelates of ligands (L1 - L2).
3.6.1. Thermal Analysis of 2-Acetyl-3-Amino-1,4-Naphthoqunone (L1)- Cu Complex
The TGA curve is refers to the thermal degradation process of [CuL1Cl2∙(H2O)2] complex which occurs in two successive stages within the temperature range of 50˚C - 1000˚C. The first stage of decomposition starts at 50˚C and end at 300˚C. The weight loss corresponds to the removal of two coordinated water molecules and Cl2 gas. The experimental mass loss of 24.75% agrees well with the calculated mass loss of 27.72%. The final stage of decomposition reveals that the complex was then further decomposed within 300˚C - 1000˚C. This corresponds to the complete decomposition of the organic portion of the ligand. The observed mass loss value of 50.38% in this stage is in good agreement with the calculated mass loss value of 51.65% and the final product is quantitatively proved to be copper oxide. The total experimental mass loss 75.13% agrees well with the calculated mass loss of 79.37%. All results are shown in Table 4.
3.6.2. Thermal Analysis of 2-Formyl-3-Amino-1,4-Naphthoqunone (L2)- Cu Complex
The thermogram of the complex [CuL2Cl2∙(H2O)2] displays two stages of decomposition within the temperature range of 50˚C - 1000˚C. The first stage of decomposition between 50˚C and 450˚C corresponds to loss of two coordinated water molecules and Cl2 gas. The experimental mass loss of 25.46% agrees well with the calculated mass loss of 28.77%. Step two starts at 200˚C and comes to end at 1000˚C corresponding to mass loss range 48.91% (calcd = 49.82%) due to decomposition of the remaining organic ligand molecule. The mass losses are in agreement with calculated mass loss based on the obtained data (Table 4). The final residue is quantitatively proved to be copper(II) oxide. The total experimental mass loss 74.37% agrees well with the calculated mass loss of 78.60%.
4. Biological Activity
The comparison of biological activity of the free ligands (L1 up to L2) and its complexes with the standard disc of Ampicillin (antibacterial G+ agent and antibacterial G− agent), Amphotericin B (antifungal agent), towards the different organisms was carried out. The data are listed in Table 5 and shown in Figure 4 and Figure 5. The free ligands and its metal chelates were screened against Staphylococcus aureus and Bacillis subtilis (G+) and Escherichia coli (G-) bacteria and candida albicans (fungi) to assess their potential antimicrobial agents.
![]()
Figure 4. Biological activity of (L1) and its metal complexes.
![]()
Figure 5. Biological activity of (L2) and its metal complexes.
![]()
Table 5. Biological activity of L1 and L2 its metal chelates.
4.1. Biological Activity of 2-Acetyl-3-Amino-1,4-Naphthoqunone (L1)
The biological activity of Ligand (2-Acetyl-3-Amino-1,4-Naphthoquinone) L1 and its metal complexes Figure 4 shows higher results than that of the free ligand. But all of them are lower than standard. Therefore, the biological activity of the complexes follow the order Co(II) > Cu(II) > Ni(II) against Staphylococcus aureus, Bacillus subtilis and Escherichia coli organisms. But with Candida albicans the biological activity follows the order Co(II) > Ni(II) > Cu(II).
4.2. Biological Activity of 2-Formyl-3-Amino-1,4-Naphthoqunone (L2)
The biological activity of Ligand (2-formyl-3-amino-1,4-naphthoqunone) L2 and its metal complexes Figure 5 shows higher results than that of the free ligand. But all of them are lower than standard. Therefore, the biological activity of the complexes follow the order Co(II) > Cu(II) > Ni(II) against Bacillus subtilis and Escherichia coli organisms for (L2) and complexes Meanwhile, the biological activity of the complexes follow the order Cu(II) > Co(II) > Ni(II) against Staphylococcus aureus. But with candida albicans the biological activity follows the order Ni(II) > Co(II) > Cu(II).
The importance of this, lies in the fact that these complexes could be applied fairly in the treatment of some common diseases caused by E. coli e.g. Septicemia, Gastroenteritis, Urinary tract infections and hospital acquired infections according to [16]. However, the complexes were specialized in inhibiting Gram-positive and Gram-negative bacterial strains. The importance of this unique property of the investigated complexes lies in the fact that, it could be applied safely in the treatment of infections caused by any of these particular strains. Generally, the activity of the free ligand was increased upon complexation with metal ions; the enhancement in activity can be explained on the basis of chelation theory, reported by [17] [18]. Chelation reduces the polarity of the metal ion considerably, mainly because of the partial sharing of its positive charge with donor groups and the possible p electron delocalization over the whole chelate ring. Chelation not only reduces the polarity of metal ion, but also increases the lipophilic character of the chelate. As a result of this, the interaction between the metal ion and the cell walls is favored, resulting in interference with normal cell processes.
5. Conclusion
In the present study, the free ligands (L1, L2) and its metal complexes Co(II), Ni(II) and Cu(II) were prepared and structurally identified. The structures of free ligands and its metal chelates are proved by elemental analyses and applying spectroscopic measurements (FT-IR, H-NMR, and mass spectra) and confirmed by thermal analyses. The synthesized free ligands are found to be biologically active and their metal complexes showed significantly enhanced antibacterial and antifungal activities against microbial strains in comparison to the free ligand.