Electrochemical Characterization of Plasma Sprayed Alumina Coatings ()
1. Introduction
Ceramic coatings generated by plasma spraying have been extensively used as anti-corrosion, abrasion and wear resistant layers for metallic structural components [1-3]. Such coatings are used by many industries such as automotive, mining and aerospace to protect internal components from corrosion and friction under severe condition such as temperature and corrosive materials. Pla-sma-sprayed (PS) alumina and alumina/titania ceramic coatings have been studied extensively because they are electrical and thermal insulators and improve the corrosion, wear, and erosion resistance of steel that has been configured [4-7].
The disadvantages associated with plasma-spraying of ceramic coatings are the rapid solidification of the flight particles and thermal stresses which propagate microcracks within the coatings and limit the coating thickness to a few hundred microns. Moreover, the pores initiated from unmelted and semi-melted particles cause defects in the thermal spray coatings. In addition, exposed metallic oxide powders can undergo phase changes, as in the case of the phase transition of plasma-sprayed Al2O3 to Al2O3 [8,9] due to the difficulty of nucleating stable -phase [10] under the rapid solidification rate associated with the plasma spraying process.
Plasma sprayed alumina coatings are corrosion-resistant; however, the porous structure of PS alumina coatings enables localized corrosion by penetration to the surface/coating interface of corrosive agents. In the case of an alumina/mild steel system, localized corrosion occurs and iron oxide is released by immersion in 3.5 wt% NaCl solution. The corrosion products at the interface could cause a failure of the whole coatings due to the decrease in adhesive strength.
In mining industries, PS alumina coatings provide excellent protection for steel tools and components against wear, abrasive and corrosion. However, the coatings defects such as cracks and pores usually cause localized corrosion in wet environment.
Pore sealing is one method to improve the corrosion resistance of the coatings. This can be performed by applying inhibitors or a phosphate conversion treatment. Heung et al. [11] found that the electrochemical impedance spectroscopy (EIS) is advanced technique to investigate the thermal barrier coatings contain metallic and ceramic layers.
In this work, the corrosion behavior of PS alumina coatings on mild steel was evaluated by means of OCP, polarization and EIS. The work will focus on the problem of corrosion in porous PS ceramic coatings and investigate it with electrochemical.
2. Experimental Method
2.1. Materials
Mild steel samples (50 × 50 × 3 mm) were grit-blasted on one side to clean and roughen the surface and followed by ultrasonic cleaning using acetone to remove grease and dust. Alumina coating was applied on the prepared mild steel surface by plasma spray system (Bay-tate-Coaken Techno-Osaka-Japan) in air. The spray parameters are listed in Table 1. Argon was employed as the working gas as well as a carrier for the alumina particles into the plasma flame. Under plasma power of 25 kW, the alumina was sprayed continuously by moving the plasma gun, attached to a programmable robot, in front of the substrate. For all coatings, the spray distance was 100 mm.
2.2. Characterization
Cross-sectioned alumina coated steel was mounted in resin and polished with diamond paste (15, 9, 3, 1 m). Scanning electron microscopy (SEM) imaging and mapping, and energy-dispersive spectroscopy (EDS) was carried out using a Philips XL30 FESEM with a LinkISIS X-ray analysis system (Oxford Instruments). EDS was carried out with a beam current of 5 kV. All samples were mounted using conductive carbon tape.
A JEOL JDX-3530 M X-ray diffractometer system was employed to analyze the phase structure of both the alumina feedstock and coatings. In the phase analysis, the radiation source was CuK; the operating voltage was 40 kV and current 40 mA.
A three-electrode corrosion cell, interfaced with a potentiostat (model HSV-100, Hokuto Denko Co., Japan), measured Tafel polarization plots. Electrochemical measurements were conducted at 25˚C. Cathodic and anodic polarizations were recorded from −1.5 to −0.2 V with a sweep rate of 0.5 mV/s in a 3.5 wt% NaCl solution. The electrochemical cell consisted of Calomel electrode (Hg/ Hg2Cl2) as the reference electrode, a Ti mesh (10 × 20 mm2) as counter electrode, with the samples mounted in the substrate holder as working electrode. The area of the sample exposed to the electrolyte was 3.14 cm2. Electrolyte solutions were prepared with analytical-grade NaCl and distilled water. The polarization curves were measured immediately after recording the open circuit potential (OCP) of the samples for 50 min in 3.5 wt% NaCl solution.
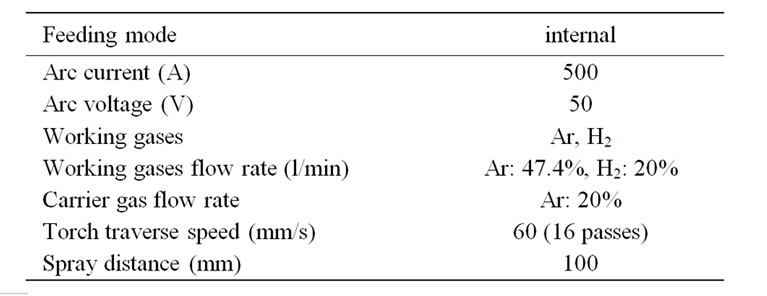
Table 1. Spray parameters used for PS alumina coatings.
3. Results and Discussion
3.1. Microstructure and Phase Structure
The PS alumina coating of thickness 500 - 600 m has lamella structure (Figure 1) formed by accumulation of molten alumina particles. The microstructure reveals the different coating defects such as pores, micro-cracks, and non-/semi-molten particles (white angular dots). The coating is well-adhered to the steel surface as there are no cracks observed at the interface.
From X-ray diffraction analysis (Figure 2), the starting alumina powder is mainly comprised of -alumina, while the main phase in the PS alumina coatings are due to -alumina, which is formed from -alumina phase transition at high plasma flame temperature.
3.2. Electrochemical Measurements
3.2.1. Open Circuit Potential
Figure 3 show the alumina-coated steel before and after corrosion test. The yellow color may be revealed to the corrosion products at the steel/coating interface that accumulated in the pores.