Fabrication of Poly(Aspartic Acid)-Nanogold Modified Electrode and Its Application for Simultaneous Determination of Dopamine, Ascorbic Acid, and Uric Acid ()
1. Introduction
Dopamine (DA) is an important neurotransmitter molecule and an indispensable intermediate species in human metabolism [1-3]. The loss of DA may cause some serious diseases, such as schizophrenia and Parkinson’s disease [4]. Similarly, ascorbic acid (AA) functions as a redox cofactor and catalyst in a broad array of biochemical reactions and processes. AA is used for the prevention of scurvy and treatment of common cold, mental illness, cancer and AIDS [5]. Uric acid (UA) is produced by xanthine oxidase from xanthine and hypoxanthine, which are, in turn, produced from purine. UA is more toxic to tissues than either xanthine or hypoxanthine because, at high concentrations, it may cause such diseases as hyperuricaemia, gout, and the Lesch-Nyan disease [6]. Usually DA, AA and UA are coexisting in our body fluids. Therefore, it is very important to develop simple and rapid methods for their simultaneous determination in routine analysis.
Electrochemical detection of DA, AA and UA is a preferred method, because they are electro-chemically active. In addition, electrochemical method offers advantages such as simplicity, speed and sensitivity. A major problem in simultaneous determination is the resolution between DA and AA as well as DA and UA. At nearly all traditional bare electrodes, DA, AA and UA are oxidized at nearly same potential, resulting in an overlapping voltammetric response. To overcome the above problem, a number of studies as reported in the literature have been carried out to develop novel, modified electrodes for simultaneous determination of DA, AA and UA, such as silver doped poly(L-valine) modified GCE [7], zinc oxide composite film [8], palladium nanoparticle-loaded carbon nanofibers modified electrode [9], carbon ceramic electrode prepared by sol-gel technique [10], Pt-Au hybrid film modified electrode [11], carbon ionic liquid electrode [12], and ruthenium oxide modified electrode [13]. In recent years, electro-synthesized polymers modified electrode have been used to detect DA and/or DA, AA and UA, simultaneously, such as poly (vinyl alcohol) [14], poly(evans blue) [15], poly(oracet blue) [16], poly(chromotrope 2B) [17], poly(4-(2-pyridylazo) resorcinol) [18], poly(eriochrome black T) [19], poly(sulfonazo III) [20], poly(N,N’-dimethylaniline) [21], poly(3,4-ethylenedioxy) thiophene [22], poly(methylene blue) [23], poly(3,4-ethylenedioxy-thiopheneco-(5-amino-2-napthalenesulfonic acid)) [24] and poly(5-amino-2-mercapto-1,3,4-thia-diazole) [25] modified electrodes.
On the other hand, nanomaterials show higher electrocatalytic activity, as a kind of special sensing material of chemically modified electrodes. Particularly, the gold nanoparticles have attracted much attention in recent decades, owing to their excellent optical and electronic properties, as well as biocompatible ability. Very recently, we reported the electrodeposition preparation of nanogold modified glassy carbon electrode and its application to simultaneous voltammetric determination of dihydroxyhenzene isomers [26].
Composite electrodes have received great attention in the last decade due to their wide applications in analytical chemistry as chemical and biosensors [27]. Preparation of composite electrodes by incorporation of metal nanoparticles or polymers on the electrode surface can be extensively enhance the catalytic activity of the electrodes towards electrochemical behaviors of many biologically importance molecules [25,28].
The poly aspartic acid film has been used to fabricate modified electrode which can enhance the electron transfer rate and reduce the overpotential for the oxidation of AA [29]. Here we report for the first time a simultaneous voltammetric determination of DA, AA and UA using a poly aspartic acid incorporated nanogold particles modified glassy carbon electrode (PAA-nano-Au/GCE). It illustrated that the PAA-nano-Au/GCE not only can catalytically oxidize DA, AA and UA simultaneously, but separate their oxidation peaks distinctly. Compared with the existing reports about simultaneous determination of DA, AA and UA (shown in Table 1), the proposed method is much more convenient to prepare the modified electrode, the selectivity and stability is better.
2. Experimental Method
2.1. Chemicals
Dopamine was purchased from Sigma-Aldrich. Uric acid, ascorbic acid, HAuCl4 and aspartic acid were obtained from Shanghai Chemical Reagents Co. Ltd. (Shanghai, China). All chemicals were of analytical reagent grade and used without further purification. Phosphate buffer solutions (PBS) with different pH values were prepared by mixing 0.10 M Na2HPO4 and 0.10 M NaH2PO4 and pH values were adjusted by addition of 1.0 M H3PO4 and/or NaOH solution. Freshly prepared solution of DA, AA, and UA were used in all experiments. Doubly distilled water was used throughout the experiments.
2.2. Apparatus
A CHI 800 electrochemical analyzer (Shanghai Chenhua Instrument Company, China) was used to perform electrode characterization and voltammetric measurements. A JEOL JSM-7600F Scanning Electron Microscope (SEM) was used to provide the information about the surface topography of electrodes. The Nyquist plots of the EIS measurement were recorded by the Potentiostat/Galvanostat Model 273A. A conventional three-electrode system was employed with a saturated calomel electrode (SCE) as the reference electrode, a platinum wire as the auxiliary electrode and a bare or modified glassy carbon electrode (GCE, 3 mm in diameter) as the working electrode. All potentials in the text were against SCE. The electrolyte solution was purged with highly purified nitrogen for at least 10 min before the experiment. The all experiments were sustained in the nitrogen atmosphere and carried out at room temperature.
2.3. Preparation of the Modified Electrode
The glassy carbon electrode was polished subsequently with 0.5 and 0.05 μm alumina slurry, then thoroughly rinsed with water and sonicated in ethanol and distilled water in turn. After being cleaned, the electrode was continuously CV scanned from –1.5 to 2.0 V at 100 mV∙s–1 for 30 cycles in 10–3 M aspartic acid aqueous solution to form poly aspartic acid film on the electrode surface. Followed, this electrode was taken out and rinsed with water, then was immersed into 0.1 M KNO3 containing 0.4 g∙L–1 HAuCl4, electrodeposition from HAuCl4 was conducted for 60 s at –0.2 V. Finally, the resulting electrode, PAA-nano-Au/GCE, was activated by several successive cyclic voltammetric scanning from –0.5 to 1.0 V with 100 mV∙s–1 in phosphate buffer solution (pH 6.5) until a steady voltammogram was obtained.
2.4. Analytical Procedure
The buffer solution was degassed with N2 and kept under a N2 blanket. After quantitative DA, AA and UA were introduced into the electrochemical cell, and then the voltammograms were recorded. The peak current was measured and recorded as a signal (Ip).
3. Results and Discussion
3.1. Surface Morphology of Poly(Aspartic Acid)-Nanogold Film and Its Electrochemical Properties
Figure 1 displays the continuous cyclic voltammograms (CVs) of aspartic acid electropolymerization onto a GCE in 10–3 M aspartic acid aqueous solution over the potential range of –1.5 V to 2.0 V for 15 cycles at a scan rate
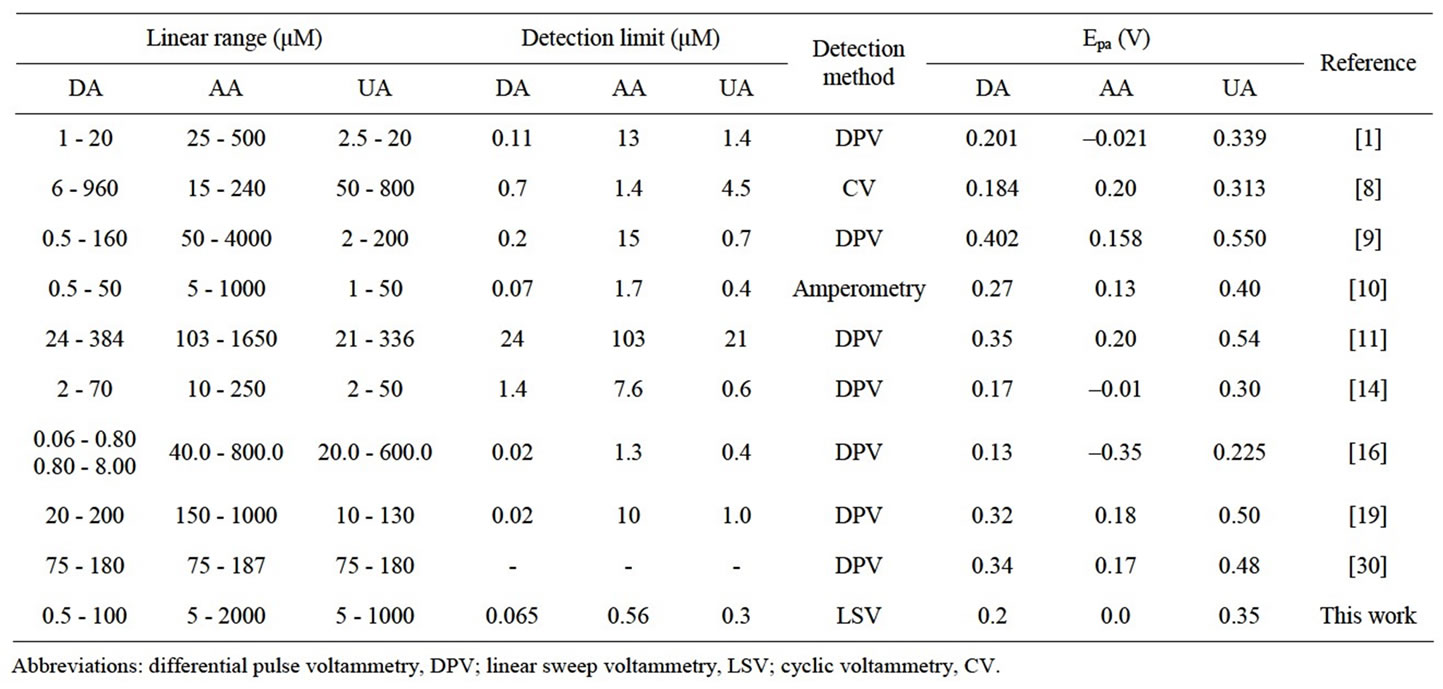
Table 1. Comparison of working range and detection limit of PAA-nano-Au/GCE with reported methods.
Figure 1. Cyclic voltammograms (CVs) of the poly aspartic acid film growth in the 10–3 M aspartic acid aqueous solution. Scan rate = 100 mV∙s–1.
of 100 mV∙s–1. As can be seen, all the anodic peak and cathodic peak currents enhanced gradually, indicating the formation and growth of an electroactive layer on the GCE surface. After the eighth cycle, the increase of these peaks current tended to be stable, implying that polymerization reach saturation. In addition, a uniform dark blue film presents on the surface of GCE, demonstrating the formation of poly aspartic acid film on GCE. As a primary amine, the electrochemical behavior of aspartic acid is in agreement with the literature [29,31,32]. We demonstrated that the potential range would affect the electropolymerization, only at high potential over 1.5 V, the oxidation of the amino group could turn into its corresponding cation radical, and these cation radicals can form carbon-nitrogen links at the carbon electrode surface. Accordingly, we propose this process illustrated in Scheme 1. The immobilized polymers through electropolymerization have many advantages in the detection of bio-molecules because of its selectivity, sensitivity and homogeneity in electrochemical deposition, strong adherence to electrode surface and chemical stability of the film.
The optimization of the PAA film thickness was also investigated. With the polymerizing cycles increasing, the electroresponse of DA, AA and UA increased at first. But when the polymerizing cycles is more than 15, the currents begin to fall. This could be explained that an increase in thickness of the film would prevent the electron transfer. Therefore, 15 cycles was used in the experiments.
Figure 2 shows the surface morphologies of bare electrode and modified electrode characterized by scanning electron microscopy (SEM). From the SEM images, It can be seen, the surface of bare GCE is smooth (Figure 2(a)). After separate gold nanoparticles were deposited on GCE, the surface presents spheroidal particles with well-distributed size, and particles’ average diameter is about 50 nm (Figure 2(b)). Similarly, separate poly aspartic acid film on the GCE shows a more uniform surface topography with pycnotic nanostructure with round parvules (Figure 2(c)). After the aspartic acid was previously electropolymerizated on the GCE, the electrodeposition of gold nanoparticles were conducted for 60 s. The small snowflake-like particles could be observed at top level layer. These observations suggest that gold nanoparticles had been incorporated into the initially formed poly aspartic acid film (Figure 2(d)).
The electrochemical impedance spectroscopy (EIS) is an effective method to probe the surface features of the modified electrodes. The Nyquist plot of EIS includes a semicircle portion and a linear portion, with the former at

Scheme 1. The modification process of aspartic acid on glassy carbon electrode.
Figure 2. SEM image of (a) Bare GCE; (b) Nano-gold/GCE; (c) Poly Aspartic Acid/GCE; (d) Poly(aspartic acid)-nanogold/ GCE.
higher frequencies corresponding to the electron-transfer limited process and the latter at lower frequencies corresponding to the diffusion process. The electron-transfer resistance (Rct) at the electrode surface is equal to the semicircle diameter, which can be used to describe the interface properties of the electrode [33].
Figure 3 displays the EIS of bare GCE (a), PAA/GCE (b), nano-Au/GCE (c) and PAA-nano-Au/GCE (d), respectively, in 0.10 M KNO3 solution containing 5.0 mM K3Fe(CN)6/K4Fe(CN)6 with the frequencies range from 0.1 to 1,00,000 Hz. As shown, the semicircle diameters decrease gradually from Figure 3(a) to (d), which indicates the electron transfer resistance at the electrode/ electrolyte interface decrease gradually. On the other hand, gold nanoparticles can act as electron transfer medium and enhance electron transfer and the result also confirms that the poly aspartic acid film incorporated gold nanoparticles had been successfully fabricated onto the glassy carbon electrode.